Abstract
Background
Evidence suggests that sarcopenia is a significant predictive factor of worst outcomes and treatment-associated toxicities in patients with metastatic solid tumours. The aim of this study was to explore the relationship between low muscle mass and clinical outcomes and immune-related severe toxicities (IrST) in patients treated with immune checkpoint inhibitors (ICIs).
Methods
A retrospective cohort of 261 consecutive metastatic solid tumour patients treated with ICIs were included in our study. Low muscle mass was defined as skeletal muscle index <41 cm2/m2 for females and <43 cm2/m2 for males if body mass index (BMI) <25 kg/m2 or <53 cm2/m2 if BMI ≥ 25 kg/m2. Severe toxicities (ST), including grade III-IV toxicities and side effects leading to treatment interruption, were recorded.
Results
The majority of patients (n = 179; 69%) included in this study had metastatic lung cancer. The prevalence of low muscle mass was 47%. The median progression-free survival (PFS) was 32.2 weeks for low muscle mass patients and 24.3 weeks for non-low muscle mass patients (adjusted HR, 0.80; 95% CI, 0.60–1.055; p = 0.11). For low muscle mass and non-low muscle mass lung cancer patients, median PFS was 24.0 weeks and 18.8 weeks (adjusted HR, 0.70; 95% CI, 0.50–0.98; p = 0.04) and median overall survival was 50.7 weeks and 41.1 weeks (adjusted HR, 0.77; 95% CI, 0.54–1.10, p = 0.15) respectively. Immune-related severe toxicities occurred in 3.3% and 9.4% of low muscle mass and non-low muscle mass patients respectively (adjusted OR, 0.69; 95% CI: 0.31–1.49; p = 0.35).
Conclusion
No difference in outcomes and safety was observed for low muscle mass and non-low muscle mass patients treated with ICIs.
Introduction
Response and tolerance to anti-cancer agents vary immensely among cancer patients. Immune checkpoint inhibitors (ICIs) have entirely transformed the management of metastatic cancer, especially in melanoma and non-small cell lung cancer [Citation1,Citation2]. Program death-1 (PD-1)/PD-Ligand-1 (PD-L1) pathway negatively regulates the anti-tumour activity of T-cells; inhibition of this pathway using blocking antibodies re-activates T lymphocytes, thereby unleashing anti-tumour immune responses. Monoclonal antibodies against PD-1 or PD-L1 provide durable responses in a subset of patients. Various predictive biomarkers have been evaluated; however, only PD-L1 expression on tumour cells and an inflamed tumour microenvironment have been established as predictive factors of efficacy in non-small cell lung cancer [Citation3]. No clinical factors or biomarkers have been validated as predictive factors of toxicity in ICIs.
Over the last decade, the development of sarcopenia in cancer patients has garnered increasing interest. Sarcopenia is characterised by a decline of skeletal muscle mass and low muscle strength and/or physical performance [Citation4]. This condition naturally and gradually occurs during ageing and has been associated with the severity and progression of several chronic diseases and cancer. The prevalence of sarcopenia in cancer patients is high, reaching almost 40% [Citation5], depending on the tumour type and stage of the disease. The development of sarcopenia has been associated with poor performance status (PS), decreased quality of life, and poor survival of cancer patients [Citation6,Citation7]; sarcopenia has also been linked to increased toxicity of chemotherapy and tyrosine kinase inhibitors [Citation8,Citation9].
While many different methods have been used to measure muscle mass, only a few have been incorporated into the routine assessment regimes of cancer patients. Computed tomography (CT), which is often used to evaluate tumour response, has also become the gold standard for assessing muscle mass and diagnosis of sarcopenia [Citation10].
Sarcopenic cancer patients often also suffer from inflammatory syndrome and metabolic disorders that can dampen the anti-tumour immune responses [Citation11]. Inflammatory syndrome, in particular, has a high impact on the response to ICIs [Citation12]. Therefore, the efficacy of ICIs might be reduced in low muscle mass patients. The aim of this study was to investigate the association between low muscle mass and clinical outcomes and toxicities in patients with metastatic solid cancers treated with ICIs.
Materials and methods
Patients
This observational retrospective cohort study was conducted at Bordeaux University Hospital (France). Our analyses included patients treated with ICIs for locally advanced or metastatic solid tumours between January 2013 and December 2017, and who had had a CT scan within four weeks before ICIs treatment. Patients with rare cancer, such as corticosurrenaloma, were excluded from the study.
The clinicopathological data of the patients were extracted from the electronic health records. The following characteristics were collected at the first treatment administration: sex, age, cancer site, metastatic site, previous systemic treatment, and PS. Modality and type of immunotherapy were also collected: anti-PD1, anti-PDL1, anti-CTLA4, ICIs combination, or combination with anti-angiogenic therapy or chemotherapy.
Immune-related severe toxicities (IrST) of included patients were grade III-IV according to NCI-CTC v4.0; data of definitive or temporary interruption of immunotherapy due to IrST, as well as data regarding the administration of corticosteroids >0.7 mg/kg to manage IrST, were collected. The study was conducted in accordance with the guidelines of good clinical practice. No consent was obtained from patients, in accordance with the French regulation.
Nutritional characteristics and anthropometric measurements
Patients’ weight and height at the first ICIs administration were collected from the medical records. Data related to weight changes in the preceding six months for patients previously treated were also collected. The Body Mass Index (BMI) was calculated and expressed in kg/m2. Information regarding appetite loss, fatigue, and pain requiring step 3 intervention of the WHO analgesic ladder were also collected. Moreover, blood parameters, including haemoglobin, albumin, C-reactive protein, and lymphocyte count, were recorded.
CT imaging
The CT scans were analysed using DxMM – MEDASYS software. Fifteen CT scans were independently reviewed by one investigator (LH) and an independent, experienced operator; both reviewers were blinded to patient data. As the evaluations for these 15 patients were identical between the two observers, the subsequent evaluations were only performed by LH. The total cross-sectional areas of skeletal muscle mass were quantified from images at the third vertebrae lumbar (L3), which contains the psoas, paraspinal muscles (erector spinae, quadratus lumborum), and abdominal wall muscles (transversus abdominus, external, and internal obliques, rectus abdominus). The Hounsfield Unit (HU) values were −29 to +150 HU. The ascertained unit was the area (cm2) of total L3 skeletal muscle, which is linearly related to whole-body muscle mass. This value was normalised to the stature (L3 skeletal muscle index, cm2/m2), as this is a common practice for BMI [Citation13]. Low muscle mass was defined as L3 skeletal muscle mass index (SMI) <41 cm2/m2 for females and <43 cm2/m2 for males if body mass index (BMI) <25 kg/m2 or <53 cm2/m2 if BMI ≥ 25 kg/m [Citation14].
Endpoints
The primary endpoint of this study was progression-free survival (PFS) of metastatic solid tumour patients in relation to low muscle mass status. Tumour evaluation was performed according to the RECIST 1.1 criteria and the clinical evaluation of an independent investigator. Secondary endpoints were PFS and Overall Survival (OS) of metastatic lung cancer patients in relation to low muscle mass status. OS was calculated from the date of the first ICIs administration to the date of death from any cause or the date of the last follow up. PFS was calculated from the date of the first ICIs administration to the date of death from any cause, or tumour progression (clinical or radiological). Objective Response Rate (ORR) was calculated according to the RECIST criteria. We also recorded information regarding IrST and rapid progression (progression within 90 days of initiation of immunotherapy according to RECIST 1.1 criteria).
Statistical analysis
Quantitative data were presented as mean ± SD, while qualitative data were shown as percentages. The comparisons between different groups were performed using the chi-square test or Fisher exact test for qualitative variables, and Student’s t-test or Wilcoxon test for quantitative variables, as appropriate.
Kaplan-Meier estimates of OS and PFS were calculated and compared between groups using the log-rank test. Variables known to affect survival were entered as covariates into a multivariable Cox proportional hazard model. These variables included sex, age, type of cancer, PS, line of treatment, and low muscle mass for PFS; sex, age, PS, line of treatment, tobacco exposure, brain metastasis, and low muscle mass were taken into account for OS. The proportionality of the HR over time was evaluated by plotting the log of the cumulative hazard versus the log of time, and by testing the correlation for Schoenfeld residuals with time. The assumptions were met for the models considered.
The associations of low muscle mass with IrST and rapid progression were estimated using multivariable logistic regression models, adjusted for age, corticosteroid use prior to ICIs, ICIs combination, history of autoimmune diseases, and PS, and for age, corticoid use at baseline, type of cancer, and association of treatment, respectively.
Finally, as different thresholds for low muscle mass exist [Citation15,Citation16], we performed sensitivity analyses to estimate the association between SMI as a continuous variable and all outcomes. We tested for non-linear relationships using penalised splines but none was retained and linear associations are presented.
All statistical analyses were performed using R software [Citation17] with a two-sided type 1 error rate set at 0.05.
Results
Clinicopathological characteristics
A total of 261 patients were included in our analyses, the clinicopathological characteristics of whom are presented in . The mean age was 61 years [33; 88], and the cohort consisted of 198 males (76%). The majority of patients had metastatic lung cancer (69%, n = 179). In most cases (82%), the patients had previously received at least one line of treatment. The PS was 1 in 55% of patients, while 57 patients (22%) had a PS ≥2. One hundred and ninety-two (74%), 43 (16%), 3 (1%), and 23 (9%) of the patients were treated with anti-PD1, anti-PD-L1, anti-CTLA-4, and ICIs combination, respectively. Low muscle mass at the start of treatment was observed in 122 patients (47%). A total of 102 (39%) were treated as a part of a clinical trial; among this population, the prevalence of low muscle mass was 37% (n = 38), while 53% (n = 84) of the patients who were not part of a clinical study had developed low muscle mass. The development of low muscle mass at baseline was associated with worse PS (p = 0.002), lower BMI (p < 0.001), weight loss (p = 0.031), and anorexia (p = 0.093). Moreover, low muscle mass patients had lower haemoglobin levels (11.7 g/dL vs. 12.3, p = 0.019) but similar albumin (36.1 mg/l vs. 37.2, p = 0.110).
Table 1. Characteristic of the patients (n = 261).
Outcomes
Response
The median PFS (mPFS) was 28.4 weeks. The mPFS was 32.2 weeks (95% CI, 21.0–48.0) for low muscle mass patients and 24.3 weeks (95% CI, 18.0–35.9) for non-low muscle mass patients (). Multivariable analysis showed that low muscle mass was not associated with PFS (HR, 0.80; 95% CI, 0.60–1.055; p = 0.11). Only Eastern Cooperative Oncology Group (ECOG) PS of >1 was associated with a decreased PFS (p = 0.001; ). The ORR in low muscle mass patients was 30%, with 28% (n = 34) of the patients having a partial response (PR) and 2% (n = 3) a complete response (CR). The ORR in non-low muscle mass patients was 25%; 22% (n = 30) patients had a PR and 3% (n = 4) had a CR. There was no association between continuous SMI and PFS (HR, 1.00; 95% CI, 0.98–1.02; p = 0.99).
Figure 1. Kaplan-Meier plot of progression-free survival for low muscle mass and non-low muscle mass patients for all patients (N = 261 patients).
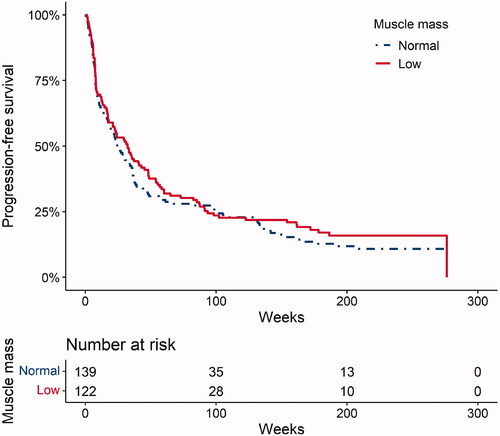
Table 2. Factors associated with progression-free survival for all patients – multivariable Cox model (N = 261 patients).
The median of PFS and OS for lung cancer patients (n = 179) was 22.3 and 44.3 weeks respectively. Half of lung cancer patients exhibited low muscle mass (49%, n = 87). The results of univariable and multivariable analyses for PFS and OS in patients with lung cancer are summarised in . The mPFS of lung cancer patients was 24.0 weeks (95% CI, 16.4–45.1) for low muscle mass patients and 18.8 weeks (95% CI, 10.3–34.1) for non-low muscle mass patients. The median OS of low muscle mass lung cancer patients and non-low muscle mass lung cancer patients were 50.7 (95% CI, 29.3–86.1) and 41.1 weeks (95% CI, 28.7–62.4), respectively. Multivariable analysis indicated that low muscle mass was not associated with OS (HR, 0.77; 95% CI, 0.54–1.10; p = 0.15) but was associated with PFS (HR, 0.70; 95% CI, 0.50–0.98; p = 0.04). The other factor associated significantly with OS or PFS was the PS (p = 0.001 and p < 0.001 respectively). There was no association between continuous SMI and PFS (HR, 1.00; 95% CI, 0.98–1.02; p = 0.90) and OS (HR, 0.99; 95% CI, 0.97–1.01; p = 0.52).
Table 3. Factors associated with Progression-Free Survival and Overall Survival in lung cancer patients – multivariable Cox model (N = 179).
Toxicity
IrST occurred in 4 (3%) and 13 (10%) of low muscle mass and non-low muscle mass patients, respectively (Supplementary data Table 1). Fatal myocarditis occurred in one patient, while three patients required treatment with immunosuppressive agents. Low muscle mass was not associated with IrST after adjusting for (OR, 0.69; 95% CI, 0.31–1.49; p = 0.35). The only factor that was associated with increased toxicity was the history of autoimmune diseases (p = 0.05; ). There was no association between continuous SMI and IrST (OR, 1.00; 95% CI, 0.96–1.04; p = 0.93).
Table 4. Factors associated with immune-related severe toxicities for all patients – Multivariable Cox model (n = 261).
Rapid progression
Ninety-one patients (35%) presented with progressive disease (PD) in the first three months. Multivariable analysis revealed that low muscle mass was not associated with rapid progression (OR, 0.73; 95% CI, 0. 42–1.25; p = 0.25) after adjusting for (Supplementary data Table 2). There was no association between continuous SMI and rapid progression (OR, 0.99; 95% CI, 0.97–1.02; p = 0.74).
Discussion
In our retrospective cohort of 261 patients treated with ICIs, we found that low muscle mass was not a significant prognostic factor for PFS. Moreover, low muscle mass and non-low muscle mass patients had similar ORR. Similar outcomes were confirmed in lung cancer patients, who represented the majority of cases in our cohort. Surprisingly, in the lung cancer patient, low muscle mass status had a favourable impact on PFS. Intriguingly, the presence of low muscle mass was not a risk factor for increased IrST.
Immune checkpoint inhibitors have radically changed the outcome of several cancers [Citation1,Citation18–20]. PD-L1 expression on tumour cells is currently the only validated predictive factor for ICIs efficacy in non-small cell lung cancer [Citation21]. Several biomarkers are currently evaluated, including the level of immune cell infiltration, intrinsic tumour characteristics (such as tumour mutational burden and metabolism), or extrinsic factors (such as microbiota) [Citation12,Citation22]. Tumour metabolism can have a great impact on the function of T lymphocytes and other immune cells [Citation23]. For example, Indoleamine 2,3-dioxygenase (IDO) expression by tumour cells results in tryptophan depletion in the tumour microenvironment, hampering T cell function [Citation24,Citation25]. The metabolic reprogramming often observed in patients with cachexia or low muscle mass could, therefore, affect the immune system and impair anti-tumour immune response, hampering the efficiency of ICIs.
Previous studies have evaluated the relationship between nutritional status and the efficacy of anticancer therapies. Albiges et al. demonstrated that high BMI (≥25 kg/m2) was associated with improved OS and PFS for patients with metastatic renal cell carcinoma treated with targeted therapies [Citation26]. Ichiara et al. evaluated the significance of BMI on ICIs efficacy in patients with metastatic lung cancer [Citation27]. For patients with high expression of PD-L1 (≥50%) and who had received pembrolizumab as a first-line treatment, there was no significant difference in the PFS and OS between patients with high and low BMI. However, for patients treated with pembrolizumab as subsequent line treatment, patients with high BMI had a significantly longer PFS and OS. In a melanoma cohort, McQuade et al. demonstrated the role of BMI in male patients treated with ICIs for metastatic melanoma [Citation28]. However contradictory data have been published by Boi et al. [Citation29] who find that obesity is associated with diminished efficacy of anti-PD-1-based therapies in renal cancer, due in part to increased inflammatory IL-1.
To our knowledge, our cohort was one of the first to evaluated ICIs and low muscle mass in the non-obesity patient. In our cohort, 50% of patients have BMI lower than 25 kg/m2 contrary to Young et al. which this population represented 25% [Citation30]. In this retrospective cohort, the authors showed that total adipose tissue index was associated with decreased response rate and PFS among women. Patients with higher muscle and low or intermediate fat have a better outcome than obese sarcopenia patients (high fat/low muscle). These data are contrary to our study. In our cohort, low muscle mass and non-low muscle mass patients had a similar median PFS and ORR, suggesting that the presence of low muscle mass did not affect the efficacy of ICIs. In lung cancer patients, we found statistical differences in mPFS between low muscle mass and non-low muscle mass patients. Contrary Roch et al. described a negative effect of sarcopenia and cachexia for lung cancer patients treated with ICIs [Citation31]. However, Roch et al. used different criteria for low muscle mass, which might also explain different results. We analysed the association between SMI as a continuous variable and all outcomes and we did not find any association.
Multivariable analysis showed that PS was the only factor that was significantly associated with mPFS. Recently Khaki et al. evaluated the impact of PS on ICIs treatment in urothelial cancer patients in a retrospective study [Citation32]. They found that patients with poor PS had a similar ORR to patients with higher PS for each line of treatment. However, patients with PS 0–1 had a longer OS than patients with PS >1 when ICIs was administrated as a first-line treatment (mOS, 15.2 vs. 7.2 months; HR, 0.62; p = 0.01); this was not the case when ICIs was given in subsequent lines (mOS, 9.8 vs. 8.2 months; HR, 0.78; p = 0.27). Hyperprogressive disease (HPD) is a condition that has been described in patients treated with ICIs. In our study, although we did not measure hyperprogression as a treatment outcome, we recorded patients with rapid progression (<3 months). We found no significant differences in this outcome between the two arms.
For patients treated with chemotherapy (cisplatinum, taxan, or anthracycline) sarcopenia has been correlated with increased toxicity [Citation6,Citation33]. Huillard et al. demonstrated that patients with BMI <25 treated with sunitinib for metastatic clear cell carcinoma experienced increased toxicity [Citation8]. Similar results were shown in patients treated with sorafenib [Citation34,Citation35]. The rate of IrST reported in the present study is similar to that reported by the majority of clinical trials with ICIs, which have shown that approximately 10% of the patients develop grade ≥3 side effects [Citation36]. The safety profile of ICIs was similar in low muscle mass and non-low muscle mass patients. Nevertheless, due to the retrospective nature of our data, we were not able to compare differences in low toxicity (grade 1–2), which are the major side effects caused by ICIs. Revel et al. found that, in a retrospective cohort of 115 patients treated with ICIs for renal and lung cancer, more low muscle mass patients experienced severe toxicity compared to non-low muscle mass patients (12.5% vs. 1.5%) [Citation37]. Similarly, in a cohort of 78 patients with metastatic non-small-cell lung carcinoma (NSCLC), grade 3–4 toxicities were more frequent in low muscle mass patients than in non-low muscle mass patients (15% vs. 0%) [Citation38]. However, as the cohort sizes were small, these results need to be interpreted with caution. Recently Hirsch et al. analysed the impact of body composition in a retrospective cohort of 92 lung cancer patients treated with nivolumab; forty-five (51.7%) of the patients were sarcopenic. The occurrence of immune-related grade 3 or 4 toxicities, or any toxicity leading to treatment discontinuation, occurred in 19 patients (21%), 76.5% of whom were sarcopenic [Citation39]. After adjusting for age, gender, BMI, CRP, PS, and tumour type, sarcopenia was the only parameter associated with an increased risk of severe toxicity (OR, 3.84; 95% CI, 1.02–14.46; p = 0.047). However, due to the small size of this cohort, this result must be interpreted with caution. The immune toxicity for patients with low muscle mass seems unclear. However, due to the low toxicity rate and a similar outcome in patients with low and non-low muscle mass as found in the present study, the benefice is to treat patients with immune checkpoint inhibitors even patients with low muscle mass.
Our study has several limitations. First, it was a retrospective and monocentric cohort with heterogeneous patients. Our cohort included patients with different tumour types, with different outcomes and complications. However, we found no differences in efficacy or toxicity between low muscle mass and non-low muscle mass patients who had different tumour types (data not shown). Moreover, our cohort included patients treated in the context of a clinical trial and patients who were not part of a clinical study. Interestingly, 31% of the patients included in clinical trials were sarcopenic. Of note, only a small number of lung cancer patients were involved in clinical trials. Another limitation is that our study did not assess the differences in low toxicity (grade ≤2). Moreover, due to the observational and retrospective nature of our study, we could not exclude residual confounding and bias due to differences in characteristics and care between low muscle mass and non-low muscle mass patients.
In conclusion, our study did not show significant differences in efficacy and toxicity for low muscle mass and non-low muscle mass patients receiving ICIs. In contrast to chemotherapy or tyrosine kinase inhibitors (TKI), ICIs could be used for low muscle mass patients without the risk of increased toxicity. However, nutrition is an important point for the treatment of patients with cancer and sarcopenia cancer patients should be detected with the rapid test as Minimal Nutrion Assesment (MNA) and all patients (except end of life care) should receive adapted nutrition (eventually with parenteral nutrition) and nutritional care should be accompanied by exercise training [Citation40]. Interestingly, our findings highlighted PS as an important factor that predicts ICIs treatment efficacy.
Author contributions
LH collected data, interpreted and write manuscript; AG Statistical analysis, interpretation of data; AQ review manuscript; MGG conception and review manuscript; RV review manuscript; EF Statistical analysis, interpretation of data and review manuscript; AR review manuscript; CD conception and review manuscript; AD conception, design, review of manuscript.
All authors approved the final version.
Ethics approval and consent to participate
The National Data Inspectorate and the Regional Committee for Medical Research Ethics approved the study. The study was performed in accordance with the Declaration of Helsinki.
Supplemental Material
Download MS Word (32 KB)Supplemental Material
Download MS Word (36.5 KB)Disclosure statement
A. Ravaud is a member of Hlobal, European and/or French advisory boards in RCC for Pfizer, Novartis, BMS, received institutional grant support by Pfizer and Novartis and housing and travel grants for meetings by Pfizer, Novartis and BMS. A Daste housing and travel grants for meetings by Merck, Astra Zeneca and BMS. M Gross-Goupil Honoraria: Ipsen, Janssen-Cilag, Sanofi, Consulting or Advisory Role: Bristol-Myers Squibb, MSD, Pfizer, Ipsen, Roche, Research Funding: Pfizer, MSD, Roche, Ipsen, Janssen-Cilag; Travel, Accommodations, Expenses: Amgen, Roche, Ipsen, MSDThe other authors declare that they have no conflicts of interest.
References
- Hodi FS, O'Day SJ, McDermott DF, et al. Improved survival with ipilimumab in patients with metastatic melanoma. N Engl J Med. 2010;363(8):711–723.
- Reck M, Rodríguez-Abreu D, Robinson AG, et al. Pembrolizumab versus chemotherapy for PD-L1-positive non-small-cell lung cancer. N Engl J Med. 2016;375(19):1823–1833.
- Gibney GT, Weiner LM, Atkins MB. Predictive biomarkers for checkpoint inhibitor-based immunotherapy. Lancet Oncol. 2016;17(12):e542–51–e551.
- Fielding RA, Vellas B, Evans WJ, et al. Sarcopenia: an undiagnosed condition in older adults. Current consensus definition: prevalence, etiology, and consequences. International working group on sarcopenia. J Am Med Dir Assoc. 2011;12(4):249–256.
- Bozzetti F. Forcing the vicious circle: sarcopenia increases toxicity, decreases response to chemotherapy and worsens with chemotherapy. Ann Oncol. 2017;28(9):2107–2118.
- Shachar SS, Williams GR, Muss HB, et al. Prognostic value of sarcopenia in adults with solid tumours: a meta-analysis and systematic review. Eur J Cancer. 2016;57:58–67.
- Bye A, Sjøblom B, Wentzel-Larsen T, et al. Muscle mass and association to quality of life in non-small cell lung cancer patients. J Cachexia Sarcopenia Muscle. 2017;8(5):759–767.
- Huillard O, Mir O, Peyromaure M, et al. Sarcopenia and body mass index predict sunitinib-induced early dose-limiting toxicities in renal cancer patients. Br J Cancer. 2013;108(5):1034–1041.
- Antoun S, Birdsell L, Sawyer MB, et al. Association of skeletal muscle wasting with treatment with sorafenib in patients with advanced renal cell carcinoma: results from a placebo-controlled study. J Clin Oncol. 2010;28(6):1054–1060.
- Mourtzakis M, Prado CMM, Lieffers JR, et al. A practical and precise approach to quantification of body composition in cancer patients using computed tomography images acquired during routine care. Appl Physiol Nutr Metab. 2008;33(5):997–1006.
- Argilés JM, Busquets S, Stemmler B, et al. Cancer cachexia: understanding the molecular basis. Nat Rev Cancer. 2014;14(11):754–762.
- Blank CU, Haanen JB, Ribas A, et al. CANCER IMMUNOLOGY. The "cancer immunogram". Science. 2016;352(6286):658–660.
- Mourtzakis M, Prado CMM, Lieffers JR, et al. A practical and precise approach toquantification of body composition in cancer patients using computed tomography images acquired during routine care. ApplPhysiolNutrMetabol. 2008;33:997–1006.
- Martin L, Birdsell L, MacDonald N, et al. Cancer cachexia in the age of obesity: skeletal muscle depletion is a powerful prognostic factor, independent of body mass index. J Clin Oncol. 2013;31(12):1539–1547.
- van der Werf A, Langius JAE, de van der Schueren MAE, et al. Percentiles for skeletal muscle index, area and radiation attenuation based on computed tomography imaging in a healthy Caucasian population. Eur J Clin Nutr. 2018;72(2):288–296.
- Derstine BA, Holcombe SA, Ross BE, et al. Skeletal muscle cutoff values for sarcopenia diagnosis using T10 to L5 measurements in a healthy US population. Sci Rep. 2018;8(1):11369.
- R Core Team. R: a language and environment for statistical computing. Vienna (Austria): R Foundation for Statistical Computing; 2019.
- Gandhi L, Rodríguez-Abreu D, Gadgeel S, et al. Pembrolizumab plus chemotherapy in metastatic non-small-cell lung cancer. N Engl J Med. 2018;378(22):2078–2092.
- Motzer RJ, Tannir NM, McDermott DF, et al. Nivolumab plus ipilimumab versus sunitinib in advanced renal-cell carcinoma. N Engl J Med. 2018;378(14):1277–1290.
- Ferris RL, Blumenschein G, Fayette J, et al. Nivolumab for recurrent squamous-cell carcinoma of the head and Neck. N Engl J Med. 2016;375(19):1856–1867.
- Rimm DL, Han G, Taube JM, et al. A prospective, multi-institutional, pathologist-based assessment of 4 immunohistochemistry assays for PD-L1 expression in non-small cell lung cancer. JAMA Oncol. 2017;3(8):1051–1058.
- Roy S, Trinchieri G. Microbiota: a key orchestrator of cancer therapy. Nat Rev Cancer. 2017;17(5):271–285.
- Domblides C, Lartigue L, Faustin B. Control of the antitumor immune response by cancer metabolism. Cells. 2019;8(2):E104.
- Munn DH, Shafizadeh E, Attwood JT, et al. Inhibition of T cell proliferation by macrophage tryptophan catabolism. J Exp Med. 1999;189(9):1363–1372.
- Metz R, Rust S, Duhadaway JB, et al. IDO inhibits a tryptophan sufficiency signal that stimulates mTOR: a novel IDO effector pathway targeted by D-1-methyl-tryptophan. Oncoimmunology. 2012;1(9):1460–1468.
- Albiges L, Hakimi AA, Xie W, et al. Body mass index and metastatic renal cell carcinoma: clinical and biological correlations. J Clin Oncol. 2016;34(30):3655–3663.
- Ichihara E, Harada D, Inoue K, et al. The impact of body mass index on the efficacy of anti-PD-1/PD-L1 antibodies in patients with non-small cell lung cancer. Lung Cancer. 2020;139:140–145.
- McQuade JL, Daniel CR, Hess KR, et al. Association of body-mass index and outcomes in patients with metastatic melanoma treated with targeted therapy, immunotherapy, or chemotherapy: a retrospective, multicohort analysis. Lancet Oncol. 2018;19(3):310–322.
- Boi SK, Orlandella RM, Gibson JT, et al. Obesity diminishes response to PD-1-based immunotherapies in renal cancer. J Immunother Cancer. 2020;8(2):e000725.
- Young AC, Quach HT, Song H, et al. Impact of body composition on outcomes from anti-PD1 +/− anti-CTLA- 4 treatment in melanoma. J Immunother Cancer. 2020;8:e000821.
- Roch B, Coffy A, Jean-Baptiste S, et al. Cachexia – sarcopenia as a determinant of disease control rate and survival in non-small lung cancer patients receiving immune-checkpoint inhibitors. Lung Cancer. 2020;143:19–26.
- Khaki AR, Li A, Diamantopoulos LN, et al. Impact of performance status on treatment outcomes: a real-world study of advanced urothelial cancer treated with immune checkpoint inhibitors. Cancer. 2020;126(6):1208–1216.
- Prado CMM, Lima ISF, Baracos VE, et al. An exploratory study of body composition as a determinant of epirubicin pharmacokinetics and toxicity. Cancer Chemother Pharmacol. 2011;67(1):93–101.
- Antoun S, Baracos VE, Birdsell L, et al. Low body mass index and sarcopenia associated with dose-limiting toxicity of sorafenib in patients with renal cell carcinoma. Ann Oncol. 2010;21(8):1594–1598.
- Mir O, Coriat R, Blanchet B, et al. Sarcopenia predicts early dose-limiting toxicities and pharmacokinetics of sorafenib in patients with hepatocellular carcinoma. PLOS One. 2012;7(5):e37563.
- Boutros C, Tarhini A, Routier E, et al. Safety profiles of anti-CTLA-4 and anti-PD-1 antibodies alone and in combination. Nat Rev Clin Oncol. 2016;13(8):473–486.
- Strulov Shachar S, Fried R, Shafran I, et al. Body composition as predictor of toxicity and outcomes in patients with metastatic non-small cell lung cancer (mNSCLC) receiving nivolumab (nivo). J Clin Oncol. 2018;36(15_Suppl):e21010–e21010.
- Revel M-P, Raynard B, Pigneur F, et al. Sarcopenia and toxicity of the anti-PD1 inhibitors in real-life lung cancer patients: results from the French nationwide SCAN study. J Clin Oncol. 2018;36(15_Suppl):e21066–e21066.
- Hirsch L, Bellesoeur A, Boudou-Rouquette P, et al. The impact of body composition parameters on severe toxicity of nivolumab. Eur J Cancer. 2020;124:170–177.
- Arends J, Bachmann P, Baracos V, et al. ESPEN guidelines on nutrition in cancer patients. Clin Nutr. 2017;36(1):11–48.