Abstract
Background
The standard in Denmark for treating breast cancer patients receiving loco-regional irradiation is tangential 3D Conformal RadioTherapy (3DCRT), treated in deep inspiration breath-hold (DIBH). Treating with Volumetric Modulated Arc Therapy (VMAT) may reduce the treatment time, which is particularly important for DIBH treatments. The VMAT should be performed without increased dose to the heart, lung, and contralateral breast. This study compares VMAT and 3DCRT for left-sided breast cancer patients with intramammary lymph node involvement.
Material and methods
Twenty left-sided breast cancer patients were included. VMAT and tangential plans were created for all patients, with a prescription dose of 50 Gy. The tangential plans used 6 MV and for larger breast combined with 18 MV. The VMAT plans utilised two 6 MV fields in a butterfly configuration. Dose planning was done in Pinnacle3 16.0 using the Auto-Planning module for the VMAT plans. Comparison of the plans was based on: mean doses, metrics provided by DBCG guidelines, dose-volume histograms and required number of breath-holds for treatment delivery in DIBH.
Results
For most OAR, the doses were similar for VMAT and 3DCRT. The target coverage was comparable, with VMAT having a statistically significant improved dose homogeneity of the target volumes. Less than half the number of breath-hold was required for VMAT compared to 3DCRT. Mean gamma pass rates (3 mm and 3%) from ArcCHECK of the VMAT plans was 98.4% (range 96.6–99.8%).
Conclusion
Automatic VMAT planning of left-sided breast cancer patients with lymph node involvement can produce dose distributions comparable to those of tangential 3DCRT, while reducing the number of breath-holds in DIBH by more than a factor of two. The reduction in breath-holds is beneficial for patient comfort and reduces the risk of intra-fraction patient motion.
Introduction
Adjuvant radiotherapy for breast cancer is often delivered as tangential 3D Conformal RadioTherapy (3DCRT) utilising deep inspiration breath-hold (DIBH) during treatment. A small region of the heart and lung may receive doses close to the target dose, especially if the internal mammary lymph nodes (IMN) are part of the target.
Tangential treatment planning using 3DCRT is tailored to the target location and anatomy of the patient. Due to inter-planner variation, the quality of the final treatment plan depends on the experience of the planner. In particular, full dose coverage of the mediastinal nodes can be difficult to achieve for some patient anatomies without increasing irradiation of the heart and lungs beyond acceptable limits, but the coverage is important to maintain good local control [Citation1–3].
Several studies have explored Volumetric Modulated Arc Therapy (VMAT) for left-sided breast cancer with node involvement, with a variety of field configurations [Citation4–7]. One of the drivers for using VMAT is the reduced treatment time delivery seen, for example, in the study from Bertelsen et al. [Citation8]. If VMAT for breast treatment can reduce the delivery time, the number of required breath-holds can be reduced, benefiting the patient comfort and reducing the risk of intra-fractional movement. The overall conclusion from the breast-related VMAT publications is that use of VMAT results in increased homogeneity and conformity of the target dose, but at the expense of increased dose to contralateral organs, ipsilateral lung and heart. Dose sparing of the heart is essential since radiotherapy for breast cancer has been linked with increased cardiac diseases [Citation9–11]. This issue was addressed by Fogliata et al. [Citation12], applying a VMAT approach based on a half arc and two tangential arcs for left-sided patients without lymph node involvement. Their study showed that a two tangential arc configuration reduced the low dose irradiation to the heart and contralateral organs compared to a half arc over the breast.
The aim of this study is to make VMAT plans with similar doses to OAR as 3DCRT for breast cancer patients receiving breast radiotherapy that includes the internal mammary and supraclavicular area. The number of required deep inspirations breath for the patient will be reported and compared to those for 3DCRT.
Methods and materials
Twenty previously treated left-sided breast cancer patients were include in the planning study. Half the patients had a mastectomy, while the other half had a lumpectomy. Within the two types of surgery, patients were selected randomly from all breast cancer patients receiving tangential 3DCRT with a prescribed dose of 50 Gy in 25 fractions from 2017 to 2018 at Odense University Hospital. Seventeen of the patients were treated in DIBH assisted by the Elekta Active Breathing CoordinatorTM. To remove a potential bias by comparing new plans with previous plans, both the 3DCRT plan and the tangential VMAT arcs were replanned within the study. All plans were made following the Danish Breast Cancer Group (DBCG) guidelines.
Target and organs at risk were all delineated according to the ESTRO guidelines [Citation13]. The planning target volume (PTV) was created with a 5 mm margin to the CTV. PTV for DVH evaluation was limited to 3 mm below the skin contour to avoid the build-up region affecting the DVH for target coverage. The CTV included three regions: (1) Primary CTV (CTVp) that for lumpectomy patients included the breast tissue and for the mastectomy patients the chest wall (2) Internal mammary node CTV (CTVn_IMN), which included the ipsilateral parasternal lymph nodes region within intercostal space 1–3, for very inferior placed tumours, the CTVn_IMN was expanded to include the fourth intercostal space (3) Supraclavicular nodes (CTVn_supraclav), which includes the lymph nodes following the veins in the supraclavicular region. Doses were prescribed to the CTVs according to DBCG guidelines – Table A.1 in supplementary notes.
3DCRT
The plans for the 3DCRT were made with manual forward planning. The beams covering the breast or chest wall were medial and lateral fields, and the lymph node area was covered by anterior and posterior fields. The fields for the breast had the MLCs open beyond the patients’ skin surface to ensure the robustness of the plan in case of swelling and intra-fractional variations. Manually created segments were used to obtain a homogeneous dose distribution following the DBCG guidelines. Plans were made by experienced radiographers. The 3DCRT was planned for an Elekta Synergy accelerator (MLCi2 2 × 40 leaves, 1 cm MLC width) using a mixture of 6 MV and 18 MV with step and shoot. The Pinnacle3 collapsed cone algorithm was used for the dose calculations.
VMAT
The VMAT planning was performed utilising the SmartArc algorithm in the AutoPlan module of Pinnacle3. Nineteen of the patients had a two-arc configuration, and one had a dual two-arc configuration due to large breast volume. The two-arc configuration had a medial and a lateral beam. The medial beam had an average beam span of 48 degrees within the gantry angles 284–355 degrees. The lateral beam had an average beam span of 71 degrees within the gantry angles 90–180 degree. The two-arc configuration and gantry angles were chosen to force the beam to deposit the dose tangential on the patient rather than a half arc that potentially would deliver low doses in a larger region than the 3DCRT. The VMAT was planned for an Elekta Versa HD accelerator (MLC Agility 2 × 80 leaves, 0.5 cm MLC width) using 6 MV. The arc had a control point spacing of 2°. For the VMAT plans, the plans were optimised with a 1 cm thick skin flash [Citation14,Citation15]. The skin flash contour was used during the whole optimisation process to force the MLCs to open beyond the patient contour (). The skin flash was removed after finalising the optimisation, and the resulting dose plan was adjusted for hot spots (>107%) by a plan re-normalisation (adjusting the total number of MU). If this process was not sufficient to remove hot spots, the plan was re-optimised. This strategy was chosen to create a robust plan and to account for swellings and inter-fractional variations in the patient. The MLC modulation was limited during optimisation by a constraint on the delivery time per beam between 45 and 55 s and by limiting the leaf motion to 0.8 cm per degree of gantry rotation. The dose calculations algorithm was the collapsed cone from Pinnacle3. Optimisation goals and settings used for Autoplan are reported in Tables A.2–4 in the supplementary material.
Figure 1. Dose distributions for one patient in the breast area on a transverse slice. CTVp is represented with orange, CTVn_IMN is represented with purple and flash bolus is seashell. Lavender line is 20% isodose curve, yellow is 95% isodose curve, blue is the 100% isodose curve, and green is the 105% isodose curve. The thin white lines represent the beam configuration. Figure (a) representing 3DCRT, and figure (b) representing VMAT.
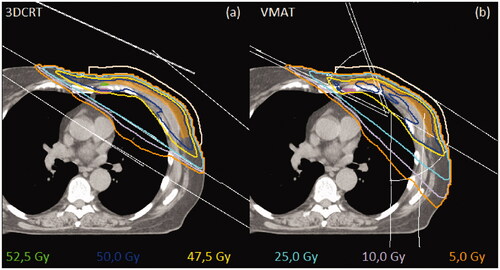
Data evaluation
RT Plan, RT Structures, total RT dose, and the DICOM Image were exported from Pinnacle3, anonymised, and processed in MATLAB R2019a. Comparison of dose distributions was divided into three parts (1) mean doses to the organs (2) the metrics used in the DBCG guideline, and (3) comparison of the entire DVH. Statistical differences were evaluated using Wilcoxon signed-rank test, a non-parametric statistical test used to compare two related samples. For parts 1 and 2, statistical significance was set at a p-value of 0.05. For part 3, the same test was used for each dose level, resulting in a p-value curve. Due to multiple testing, a single point below 0.05 will not indicate statistical significance; only larger regions below 0.05 will indicate dose differences between the two sets of plans. The p-curve is thus a visual tool to indicate potential differences and not as such a statistical test (see further description in Bertelsen et al. [Citation8]). The homogeneity index (HI = (D2%-D98%)/D50%) was calculated according to ICRU Report 83 [Citation16].
Plan delivery evaluation
The dose delivery uncertainty was measured for the VMAT dose plans utilising the ArcCHECK phantom (Sun Nuclear Corporation (SNC), Melbourne, FL, USA). The SNC PatientTM Software Version 8.3 was used for the analysis of irradiation of the ArcCHECK phantom. Details of precision and use of ArcCHECK have been discussed elsewhere [Citation17,Citation18]. In the ArcCheck evaluation, doses were measured as absolute dose, and only doses above 10% of the maximum dose were included in the analysis. Our institution's standard clinical dose and distance metric is 3% 3 mm with plan acceptance for pass rates above 95%. Besides these clinical values, the dose distribution was also evaluated using 2% 2 mm and 5% 2 mm.
The standard time of a deep inspiration breath-hold in our institution is 25 s. For both VMAT and 3DCRT plans, we estimated the minimum number of required breath-holds for treatment delivery. For 3DCRT, multiple step and shoot segments were allowed within the same breath-hold if possible.
Results
For all 20 patients, it was possible to produce VMAT plans with dose distributions similar to the 3DCRT plans. The plans were following the requirements listed in Table A.1 in the supplementary material. The target coverage (V95% >95%, V90%>95% and V90%>95%) for CTVp, CTVn_supraclav and CTVn_IMN for 3DCRT was achieved for 17, 19 and 12 plans respectively. The target coverage for CTVp, CTVn_supraclav and CTVn_IMN for the VMAT plans was achieved for 17, 20, and 18 plans, respectively. The general challenges for achieving coverage for the CTV’s for the two modalities were as following: For the CTVp, patients who had a thin chestwall or had a large breast (>1300 cm3). For the CTVn_IMN, it was due to heart, contralateral breast and lung constraints. For the CTVn_supraclav, the CTV was compromised due to humeral head dose. An example of the isodose distributions for the types of treatment is shown in .
Target volumes
The median and interquartile range of the CTVp volume was for the mastectomy patients 192 cm3 [159–244 cm3], while it was 672 cm3 [562–1030 cm3] for the lumpectomy patients.
shows the mean DVHs for the CTVs and the PTV for the two types of treatment. Except for CTVp, where the dose coverage is very similar, the VMAT has a steeper DVH with an improved dose homogeneity. HI for 3DCRT was median [interquartile range]: CTVp: 0.132 [0.099–0.150], CTVn_IMN: 0.141 [0.132–0.172] and CTVn_supraclav: 0.123 [0.111–0.164] and for VMAT: CTVp: 0.075 [0.065–0.100], CTVn_IMN: 0.072 [0.060–0.084] and CTVn_supraclav: 0.0693 [0.0614–0.0751]. All statistically significant favouring the VMAT treatment (p-values of 0.004, 0.002 and <0.001).
Figure 2. Mean DVHs of the target volumes. 3DCRT (solid, red) and VMAT (dashed, blue) are shown together with a p-value from the Wilcoxon-signed rank test (grey).
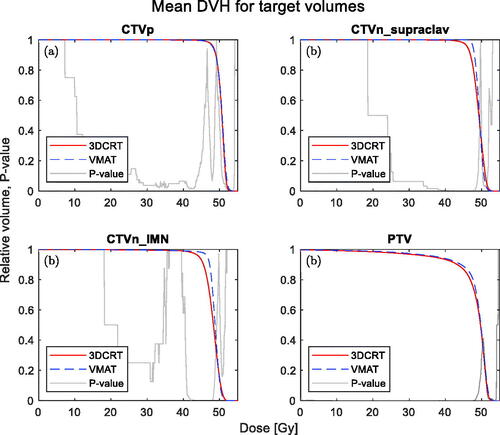
The mean doses for the CTV volumes is shown in . Only the CTVn_IMN showed a statistically significant difference between VMAT and 3DCRT. Median values of DBCG target constraints are given in . Of the three CTVs, the CTV related to the supraclavicular region and internal mammary nodes show a statistically significant difference favouring VMAT; however, no statistically significant difference is observed for the breast/chest wall CTV.
Table 1. The median value of the mean of target organs and OARs.
Table 2. Median of metrics stated in the DBCG of target organs and OARs.
OARs
Lungs
shows the DVHs for the contralateral and ipsilateral lung, respectively. For the contralateral lung, there are no differences between VMAT and 3DCRT. The DVH for the ipsilateral lung shows differences in three dose regions the low (<10Gy), intermediate (the 30–40 Gy range), and high doses (48–50 Gy). The VMAT dose is reduced in the intermediate range but higher in the low- and high-dose regions.
Figure 3. Mean DVHs of the OARs volumes. 3DCRT (solid, red) and VMAT (dashed, blue) are shown together with a p-value from the Wilcoxon-signed rank test (grey).
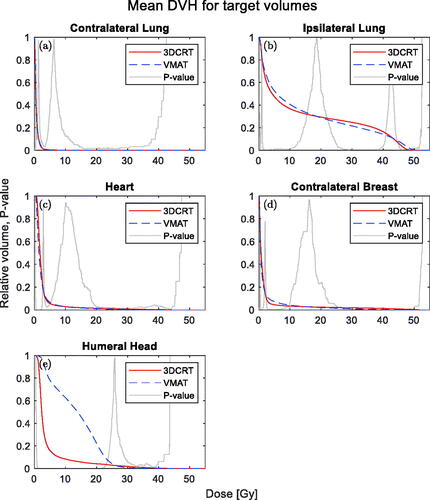
Mean doses of the lung are shown in and do not show any significant differences between the VMAT of 3DCRT treatment. The DBCG guideline metric (V20Gy) for the ipsilateral lung shown in did neither show any significant difference.
Heart
The dose distributions for the heart were almost identical, but the metrics reported were slightly lower for VMAT both in terms of mean heart dose and in terms of V40Gy and V20Gy (see and ).
In , the mean DVHs for the heart is seen. The p-value curve indicates a small difference at doses below 2 Gy and above 20 Gy.
Contralateral breast
In , the median of the mean dose is showed for the contralateral breast, which did not show any statistically significant differences. shows the mean DVHs for the contralateral breast. The p-value curve is below 0.05 in the high doses above 35 Gy.
Humeral head
shows a statistically significant difference between the mean doses to the humeral head favouring 3DCRT. The dose from VMAT was higher for doses below 22 Gy but lower for doses above 30 Gy, as shown in .
Plan delivery and quality
All VMAT plans were delivered successfully on an Elekta Versa HD accelerator. The VMAT dose was delivered with a mean and range of gamma pass rates of 98.5% [96.6–99.8] using the 3% 3 mm criterion, 99% [98.1–100%] using 5% 2 mm and 91.2% [86.1–99.8%] using2% 2 mm.
The median and interquartile range of breath-holds were 9 [9–10] and 4 [4–4] for 3DCRT and VMAT, respectively.
Discussion
In the present study, it was feasible to create tangential VMAT plans for all patients. The VMAT target doses were overall similar to 3DCRT, however, with differences for some OAR and targets. Target coverage was improved for VMAT and except for the humeral head, the dose to OAR were similar or lower.
The number of needed breath-holds was reduced by a factor of two for VMAT compared to 3DCRT, which may lead to better patient comfort and less risk of intra-fractional patient movement.
There was a high accuracy of the dose delivery for the VMAT plans. However, the accuracy is related to the degree of dose modulation during delivery. Therefore, it is essential to limit the modulation/complexity of the treatment plans to achieve high dose delivery accuracy. In an initial part of the work (data not shown), the modulation constrains (leaf motion) described in the method section were not included in the plan optimisation, resulting in unacceptable delivery accuracy. It is therefore essential to use dose modulation constraints during plan optimisation.
The robustness of the VMAT plans was enhanced by using a skin flash contour during plan optimisation to compensate for the patients’ swelling or movement; however, the skin flash was not explicitly tested within the study. The impact of the skin flash was investigated previously in a study by Lizondo et al. [Citation14]. They showed plan robustness with isocentre displacements up to 5 mm based on a 1 cm skin flash and a PTV-CTV margin of 5 mm. Other aspects of robustness are not covered in this study. Thus, for clinical implementation, robustness should be considered to ensure sufficient target coverage.
The use of Autoplan for treatment planning compared to the 3DCRT forward planning will likely reduce the inter-planner variation. The reduced inter-planner variation has been reported by Clemente et al. [Citation19] but was not evaluated in this study.
The irradiation of the heart was almost identical between the two types of plans, with a small reduction of the mean heart dose for the VMAT plans. A recent study by Lorenzen et al. [Citation11] showed that mean dose is correlated to major coronary events (19% increase in the excess odds ratio per Gy of mean heart dose). The reported values from Lorenzen et al. and the observed difference in mean heart dose will result in 3DCRT having an odds ratio of 1.06 on average for major coronary events relative to the VMAT treatment.
The risk of radiation-induced secondary tumours is a concern for the healthy tissue in the contralateral breast and lung, as reported by Stovall et al. [Citation20] and Grantzau et al. [Citation21]. It is, therefore, crucial to keep the contralateral doses as low as for the 3DCRT method when using VMAT as done in this study.
The only potentially unfavourable observation for VMAT was the humeral head, where doses below 22 Gy was increased compared to 3DCRT. There is limited evidence for critical dose limits for the humeral head. A study by Johansen et al. [Citation22] showed a correlation between the shoulder joint and arm irradiation and related morbidity. Their maximum detected Spearman correlation between irradiation and morbidity was below 0.2 and observed at dose levels around 15 Gy. Although the correlation is limited, it might indicate that a dose reduction to the humeral head might be warranted before clinical implementation. For the current study, the applied constraint was defined according to the DBCG guidelines, thus a maximum dose below 25 Gy.
The findings in this study show promising potential for VMAT in breast cancer radiotherapy with the major benefit of reducing the number of required deep inspiration breath-holds to less than half of that for the 3DCRT treatment. In a clinical implementation of VMAT, each plan should be carefully inspected for doses outside the delineated regions. This may occur in inverse planning where the optimiser primarily adapts the plans to delineated volumes. Furthermore, anatomical changes during the treatment course should be monitored carefully as the VMAT plans could be less robust to such changes.
Supplemental Material
Download PDF (541.4 KB)Supplemental Material
Download PDF (231.4 KB)Acknowledgment
We thank the DBCG Radiotherapy committee for providing helpful input and discussion at national meetings throughout the study.
Disclosure statement
No potential conflict of interest was reported by the author(s).
References
- Whelan TJ, Olivotto IA, Parulekar WR, MA.20 Study Investigators, et al. Regional nodal irradiation in early-stage breast cancer. N Engl J Med. 2015;373(4):307–316.
- Poortmans PM, Collette S, Kirkove C, EORTC Radiation Oncology and Breast Cancer Groups, et al. Internal mammary and medial supraclavicular irradiation in breast cancer. N Engl J Med. 2015;373(4):317–327.
- Thorsen LBJ, Offersen BV, Danø H, et al. DBCG-IMN: a population-based cohort study on the effect of internal mammary node irradiation in early node-positive breast cancer. J Clin Oncol. 2016;34(4):314–320.
- Popescu CC, Olivotto IA, Beckham WA, et al. Volumetric modulated arc therapy improves dosimetry and reduces treatment time compared to conventional intensity-modulated radiotherapy for locoregional radiotherapy of left-sided breast cancer and internal mammary nodes. Int J Radiat Oncol Biol Phys. 2010;76(1):287–295.
- Nicolini G, Fogliata A, Clivio A, et al. Planning strategies in volumetric modulated are therapy for breast. Med Phys. 2011;38(7):4025–4031.
- Tyran M, Mailleux H, Tallet A, et al. Volumetric-modulated arc therapy for left-sided breast cancer and all regional nodes improves target volumes coverage and reduces treatment time and doses to the heart and left coronary artery, compared with a field-in-field technique. J Radiat Res. 2015;56(6):927–937.
- Dumane VA, Bakst R, Green S. Dose to organs in the supraclavicular region when covering the internal mammary nodes (IMNs) in breast cancer patients: a comparison of volumetric modulated arc therapy (VMAT) versus 3D and VMAT. Zhang Q, editor. PLOS One. 2018;13(10):e0205770.
- Bertelsen A, Hansen CR, Johansen J, et al. Single arc volumetric modulated arc therapy of head and neck cancer. Radiother Oncol. 2010;95(2):142–148.
- Rehammar JC, Jensen M-B, McGale P, et al. Risk of heart disease in relation to radiotherapy and chemotherapy with anthracyclines among 19,464 breast cancer patients in Denmark, 1977-2005. Radiother Oncol. 2017;123(2):299–305.
- EBCTCG. Effects of radiotherapy and of differences in the extent of surgery for early breast cancer on local recurrence and 15-year survival: an overview of the randomised trials. Lancet. 2005;366:2087–2106.
- Laugaard Lorenzen E, Christian Rehammar J, Jensen M-B, et al. Radiation-induced risk of ischemic heart disease following breast cancer radiotherapy in Denmark, 1977–2005. Radiother Oncol. 2020;152:103–110.
- Fogliata A, De Rose F, Franceschini D, et al. Critical appraisal of the risk of secondary cancer induction from breast radiation therapy with volumetric modulated arc therapy relative to 3D conformal therapy. Int J Radiat Oncol Biol Phys. 2018;100(3):785–793.
- Offersen BV, Boersma LJ, Kirkove C, et al. ESTRO consensus guideline on target volume delineation for elective radiation therapy of early stage breast cancer. Radiother Oncol. 2015;114(1):3–10.
- Lizondo M, Latorre-Musoll A, Carrasco P, et al. Pseudo skin flash on VMAT in breast radiotherapy: optimization of virtual bolus thickness and HU values. Phys Med. 2019;63:56–62.
- Bogue J, Wan J, Lavey RS, et al. Dosimetric comparison of VMAT with integrated skin flash to 3D field-in-field tangents for left breast irradiation. J Appl Clin Med Phys. 2019;20(2):24–24–29.
- ICRU: Prescribing, Recording, and Reporting Photon-Beam Intensity-Modulated Radiation Therapy (IMRT). ICRU Report 83, J. ICRU, Volume 10(1). Oxford (UK): Oxford University Press; 2010.
- Thiyagarajan R, Sinha S, Yadav G, et al. SU-E-T-130: IMAT patient specific quality assurance using ArcCHECK diode array detector. Med Phys. 2012;39(6Part11):3733–3733.
- Hansen CR, Bertelsen A, Hazell I, et al. Automatic treatment planning improves the clinical quality of head and neck cancer treatment plans. Clin Transl Radiat Oncol. 2016;1:2–8.
- Clemente S, Oliviero C, Palma G, et al. Auto- versus human-driven plan in mediastinal Hodgkin lymphoma radiation treatment. Radiat Oncol. 2018;13(1):202.
- Stovall M, Smith SA, Langholz BM, et al. Dose to the contralateral breast from radiotherapy and risk of second primary breast cancer in the WECARE study. Int J Radiat Oncol. 2008;72(4):1021–1030.
- Grantzau T, Thomsen MS, Vaeth M, et al. Risk of second primary lung cancer in women after radiotherapy for breast cancer. Radiother Oncol. 2014;111(3):366–373.
- Johansen S, Fosså K, Nesvold IL, et al. Arm and shoulder morbidity following surgery and radiotherapy for breast cancer. Acta Oncol 2014;53(4):521–529.