Abstract
Background
Biologically created subvolumes enable non-uniform dose distributions in prostate cancer radiotherapy (RT) thus potentially improving therapeutic ratio and reducing toxicity. We present the long-term outcome of men receiving focal boosting of carbon-11 acetate (ACE) PET-CT metabolically active areas in prostate carcinoma.
Material and methods
Thirty men with hormone naïve localized prostate carcinoma underwent ACE PET/CT for RT planning. There were five low-, 17 intermediate-, and eight high-risk patients. Based on thresholding of the standardized uptake values (SUVs) metabolic target volumes (MTVs) corresponding to intraprostatic lesions (IPLs) were contoured. Two planning target volumes (PTVs) were applied i.e., PTVlow-risk for the whole prostate with 8–10 mm margin and PTVhigh-risk for the MTV. Pelvic nodes were not irradiated. Late toxicity of biologically guided RT was reviewed after a median of 63 months and outcome after a median follow-up of 124 months.
Results
Median doses to PTVlow-risk, PTVhigh-risk, prostate, and MTV were 72.9 Gy, 79.4 Gy, 76.6 Gy, and 80.4 Gy, respectively, in 38 fractions. The 10-year cancer-specific survival was 86% and the biochemical failure-free ratio 68%, respectively. The median biochemical progression-free survival (PFS) was 37, 108, and 119 months in the high, intermediate, and low-risk groups, respectively, the difference being significant between high and intermediate-risk groups (p = 0.02). One patient (3%) presented with locoregional and 5 (17%) with distant nodal metastases. Five patients (17%) had a biochemical relapse. A larger MTV was associated with shorter PFS (r = −0.41, p = 0.02), but had no influence on OS. No other statistically significant differences in the dose painting parameters were observed between recurrence-free and recurring patients.
Conclusions
Biological guidance for dose-escalated prostate RT is feasible with ACE PET/CT. Since a larger MTV may be associated with a higher risk for progression, we encourage further study of dose-escalation to ACE-positive lesions considering the low toxicity of our protocol.
Introduction
Dose-escalation in radiotherapy (RT) of localized prostate cancer (PCa) has been established to improve biochemical control rates in several prospective randomized studies [Citation1]. Currently, intensity-modulated and image-guided radiotherapy (IMRT/IGRT) are standard techniques where the whole prostate receives a uniform dose and bladder and rectum represent organs-at-risk (OAR) for dose-escalation [Citation2,Citation3]. Escalation of dose in the actual tumor rather than whole prostate has the potential to improve disease control without increasing toxicity since local recurrences often occur in the site of the primary lesion [Citation4]. This strategy requires multimodal imaging since the microscopic spread of PCa is often multifocal and computed tomography (CT) used for calculation of electron density does not depict tumor foci within the gland [Citation5].
Our experience using carbon-11-labeled tracer [11C]acetate (ACE) with positron emission tomography/computed tomography (PET/CT) indicates that PET-guided RT may be applicable for dose-escalation of intraprostatic lesions (IPLs) [Citation6]. This previous study showed that ACE PET/CT facilitates delivery of non-uniform dose distribution within the prostate while doses to the rectum and the bladder are kept at a low level. The technique is now commonly referred to as dose painting in RT literature [Citation7]. We present now 10-year outcome and 5-year toxicity of patients with PCa who underwent ACE PET/CT guided simultaneous boost (SIB) to IPL as a part of their IMRT dose painting protocol. The focus was to study the effect of dose escalation of metabolic tumor volumes (MTVs) on progression-free survival and long-term toxicity in a patient population where overall survival currently exceeds beyond 10 years.
Material and methods
Patients
This was a non-randomized study where 30 patients referred to radical external beam RT were prospectively enrolled between January 2008 and September 2010. All patients had histologically confirmed untreated PCa and WHO performance status two or better. Other inclusion criteria were: age 50–85 years, histological sampling consisting typically of 3–4 biopsies from both lobes, and clinical stage T1c-T3aN0M0 based on the transrectal ultrasound, pelvic CT/MRI, and bone scintigraphy. Prior to treatment three fiducial markers were implanted into the prostate to enable accurate daily kV-kV image guidance. Patients on neoadjuvant hormonal therapy were excluded. Characteristics of the 30 patients are described in . All patients gave their written informed consent and the study protocol was approved by the Ethical Board of the Hospital District of Southwest Finland.
Table 1. Patient characteristics.
Radiotherapy and plan evaluation
PET/CT applied for RT planning has been previously described in detail [Citation6] and is briefly summarized below. Patients underwent pretreatment PET/CT of the pelvic region and with an intravenous mean dose of 670 MBq [11C] acetate prior to acquisition. Based on the standardized uptake values (SUVs) MTVs corresponding to intraprostatic lesions (IPLs) were delineated using a median SUV 2.9 as a threshold (range 1.9–4.1). Two main dose levels were applied i.e., PTVlow risk and PTVhigh risk. The PTVhigh risk was defined by adding patient anatomy dependent 0–6 mm margin to the MTV to limit the overlap with OARs. Correspondingly, the PTVlow risk was created by expanding the prostate with an 8–10 mm margin as seen on the CT, where the smaller margin was applied posteriorly to spare the rectum whenever necessary. Attention was paid to include the seminal vesicle bases in the PTVlow risk. None of the patients had ACE PET/CT-positive pelvic lymph nodes and did not have pelvic lymph nodes included in the treatment volumes. Boolean operators were applied such that PTVlow risk excluded PTVhigh risk.
Radiotherapy was delivered in 38 fractions, five fractions a week. Total median doses were 72.9 Gy to PTVlow risk, 79.4 Gy to PTVhigh risk, 76.6 Gy to the whole prostate, and 80.4 Gy to the MTV. A typical plan is shown in . At follow up the number of IPLs, the volume, and SUV within the MTVs as well as median doses and dose heterogeneity indices (ref ICRU 83) for the PTVhigh risk, PTVlow risk, the prostate, and the MTV was recorded. Furthermore, dose-volume histograms (DVHs) for the OARs the bladder, the rectum, and the penile bulb were retrieved from the treatment planning system (TPS). DVHs and dose constraint parameters were controlled and compared for patients with and without grade 2–3 toxicity.
Figure 1. Axial (A) and coronal (C) ACE-PET/CT views of a patient with intermediate-risk prostate carcinoma. Dose distribution in basal left peripheral metabolic target volume (MTV, arrow) is shown in planning CT (B) and DVH of MTV, PTVhigh-risk, and PTVlow-risk and critical normal tissues (D). Note physiologic tracer activity of rectal (A) and sigmoid wall (C) indicated with arrows. The patient is recurrence-free 9 years after biologically guided RT.
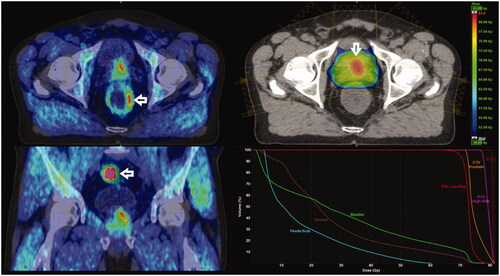
Clinical follow-up
Serum PSA was measured and the patients were seen by an oncologist at baseline, 3 and 12 months after the end of RT and thereafter yearly. Early toxicity was evaluated on follow-up visits 3 and 12 months after RT. The late toxicity was evaluated on the 5-year visit with the IPSS questionnaire [Citation8] using standard Radiation Therapy Oncology Group (RTOG) criteria taking into account medical records, physical examination, and patient-reported symptoms. Biochemical failure was defined by the Phoenix consensus definition as the nadir PSA concentration plus 2 ng/ml [Citation9].
Statistical analysis
Categorial variables are characterized using frequencies and percentages and continuous variables are characterized using means and medians and interquartile range (IQR). Comparison between IPL groups and between risk groups in PFS and OS were studied using the Kruskall-Wallis test and for pairwise comparisons, Mann-Whitney U test with Bonferroni-correction was used. Spearman correlation coefficient (r) was calculated between MTV, PFS, and OS. Associations between relapse or exitus and study variables (MTV, SUV threshold, prostate, PTV high risk, PTV low risk, and number of IPLs) were studied using logistic regression analysis. Kaplan-Meier method was used with a cumulative incidence curve.
P-values less than 0.05 were considered statistically significant. Statistical analyses were carried out using the SAS System for Windows, release 9.4 (SAS Institute Inc., Cary, NC).
Results
Survival and recurrences
At a median follow-up time of 124 (range, 105–137) months, there were 11 recurrences in 30 patients (37%). Of these, five were biochemical, one locoregional, and five distant relapses. A total of eleven deaths were observed during the follow-up time. Three men died of prostate cancer and another four of lung cancer, gastric cancer, and melanoma. The remaining four patients died because of vascular disease or unknown reasons but were free from the progression of PCa. All 11 patients received hormonal therapy after the relapse.
At 10 years, the disease-specific survival rate was 86% and biochemical failure-free ratio 68%, respectively. The median overall survival (OS) in the high, intermediate, and low-risk groups was 69, 122, and 125 months, respectively. Compared to the intermediate-risk group, OS was significantly shorter in the high-risk group, (p = 0.006) (). The median biochemical progression free survival (PFS) in the high, intermediate, and low-risk group was 37, 108, and 119 months, respectively. PFS was significantly shorter in the high-risk group, compared with the intermediate-risk group (p = 0.02), but not with the low-risk group (). The low number of patients in the low-risk group precluded a comparison of their survival with other risk groups.
Dosimetric and metabolic evaluation of risk for recurrence
represents all dosimetric parameters and clinical target volumes derived from PET/CT imaging. In general, there were no significant differences in delivered dose between patients in dose levels of the PTVhigh-risk and PTVlow-risk or whole prostate. Furthermore, the dose homogeneities were similar between groups for both PTVs, the prostate as well as the MTVs (data not shown). By contrast, the median dose of MTV in recurring patients was higher than that of patients remaining stable (80.7 vs 80.2 Gy, p = 0.04). While this finding may be clinically insignificant it shows that recurring patients were not underdosed compared to non-recurring patients. Second, median MTV was 2.6 (range, 0.3–13.0) cm3 for non-recurring and 8.2 (range, 1.3–23.3) cm3 for recurring patients. This difference was not statistically significant (p = 0.2280). In line with this, there was a moderate negative correlation between MTV and PFS (r = −0.41, p = 0.02), but not with OS. We also evaluated the effect of the number of imaging-defined IPLs on survival. Most commonly the patients had two IPLs (n = 17) while nine patients had more than two lesions and four only one, respectively. The number of IPLs did not show a statistically significant impact on PFS or OS. Another non-significant observation suggested that the odds ratio for risk of relapse increased 13% by every increase of 1 cm3 in MTV size. Furthermore, MTV rather than the number of lesions was decisive in predicting risk for recurrence.
Table 2. Dose painting characteristics.
Acute and late toxicity
Some acute but generally mild toxicity typical for contemporary RT techniques and fractionation was seen which resolved during the first 1–2 years after RT. Five (5/30, 17%) patients had radiation proctitis or bleeding hemorrhoids and 2/30 (7%) patients had urinary retention or hematuria after RT. There was no grade 3 or 4 short-term toxicity.
Twenty-four men (24/30, 80%) were seen at a 5-year follow-up visit for evaluation of long-term toxicity. Of these, in one patient grade 2, in another grade 3 urinary symptoms, and in two patients grade 3 erectile symptoms were present already before RT. These patients were excluded from the toxicity evaluation. In line with urinary symptoms, late gastrointestinal (GI) symptoms were uncommon. Only 1/25 patients (4%) suffered from grade 3 GI diarrhea and no grade 2 GI symptoms were present. By contrast, erectile dysfunction was quite common with 15/23 of men (65%) reporting G 2 and G 3 symptoms respectively. The toxicity of 24 men at a 5-year-control visit is shown in supplementary Table 1. Dose-Volume-Histograms (DVH) for the rectum and bladder are shown in .
Figure 3 Dose-volume histograms (DVH) of patients who were evaluated for late radiation toxicity five years after treatment. The upper panel shows pooled DVHs for the bladder in 23 patients (left) and the rectum in 24 patients (right). In the lower panel, the same DVHs are averaged by long-term toxicity where grade 0–1 is shown by dashed and grade ≥2 by solid lines, respectively.
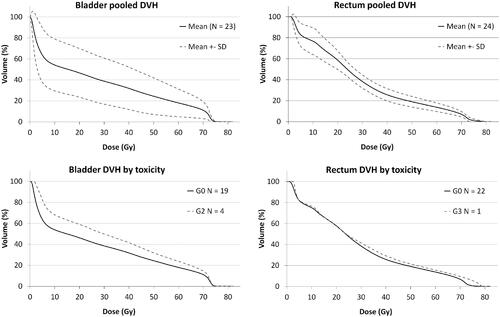
Discussion
Modern prostate RT attempts to improve outcomes by dose escalation with neoadjuvant antiandrogen therapy and, more recently, by hypofractionation using conformal techniques, such as stereotactic body RT. Independent of the chosen technique, sparing of normal tissue is essential to minimize late toxicity since survival beyond 10 years after RT is common.
We designed this protocol to study the impact of ACE PET/CT on the outcome of IMRT in patients with localized PCa. We aimed to reduce toxicity with RT dose painting by applying SIB delineated with metabolic imaging. Randomized studies boosting IPLs in PCa RT will soon define the role of multimodality imaging with PET and magnetic resonance imaging (MRI) and our results are among the first to report the long-term outcome of such a technique.
PET with prostate-specific membrane antigen (PSMA) ligands is now recommended for evaluation of PCa after biochemical recurrence [Citation2] and Hofman et al. have recommended replacing conventional CT and bone scan with PSMA targeted imaging in men with high-risk PC in staging before curative-intent therapy, due to its superiority to define metastases and use as a single-step method [Citation10]. Anyhow, the use of PSMA PET/CT in staging or management of the localized disease is less well established although encouraging [Citation11]. At the time of patient enrollment in the current study, PSMA was not, however, available. We, therefore, used ACE for therapy planning since the tracer shows minimal excretion in urine and has earlier been shown to be feasible for evaluating patients experiencing PSA-relapse after radical prostatectomy or RT [Citation12]. Uptake of ACE in PCa is based on altered lipid metabolism and although some uptake in benign prostatic hyperplasia does occur it does not compromise biologically boosted RT as long as the minimum prostate dose is equal to clinical standard and OARs receive the acceptable dose. In line with this, we were able to design plans with the low rectum and bladder doses () which translated to low toxicity at 5-years and reasonable outcome considering the modestly escalated dose in IPL(s).
Other dose painting studies have used different PET-tracers and multiparametric MRI in their protocols. Zamboglou et al. studied 10 patients who underwent 68Ga-HBED-CC PSMA-PET/CT prior to prostatectomy. Virtual IMRT plans of 77 Gy to the whole prostate and a SIB to the GTV-PET up to 95 Gy were generated. The authors concluded this to be technically feasible and resulting in significantly higher tumor control probability without higher normal tissue complication probability [Citation13]. Kerkmeijer et al. [Citation14], evaluated the survival and late toxicity after MRI-defined RT of 77 Gy (n = 287) with an MRI-defined SIB up to 95 Gy (n = 284) in the newly reported FLAME study. At five-year follow-up, biochemical DFS was significantly higher in the focal boost compared with the standard arm (92% and 85%, respectively), but there were no differences in PC-specific survival or OS. The cumulative incidence of late GU and GI toxicity grade two or higher was 23% and 12% in the standard arm versus 28% and 13% in the SIB arm, respectively [Citation14]. Hypo-FLAME-study reported acceptable acute GU and GI toxicity after extreme hypofractionated RT where no ≥ Grade 3 toxicity was observed. The mpMRI-defined prostate tumors received 35 Gy in 5 weekly fractions with SIB up to 50 Gy but as of now, no long-term-toxicity data is available [Citation15]. Standard and hypofractionated dose-escalated prostate RT was delivered also in DELINEATE-trial [Citation16]. Cohort A underwent RT of 74 Gy in 37 fractions with SIB 82 Gy to mpMRI-defined lesions, and cohort B moderately hypofractionated RT of 60 Gy in 20 fractions with SIB up to 67 Gy, respectively. The cumulative toxicity was evaluated at 1 year and was comparable to contemporary series without an intraprostatic boost.
An encouraging 10-year survival rate of 69% (n = 71) was reported by Schild et al. [Citation17] who applied 111In capromab pendetide (ProstaScint) gamma imaging for dose painting of intraprostatic lesion to 82 Gy while the rest of prostate received 75.6 Gy. In line with our study, the incidence of long-term G3-4 toxicity was low. Our corresponding PCa-specific 10-year survival rate of the entire cohort was 86%. Also, Vora et al. reported similar 5-year toxicity rates in IMRT-treated PCa patients who received a mean radiation dose of 75.6 Gy [Citation18]. Pinkawa et al. [Citation19] evaluated survival and long-term toxicity in 383 intermediate- and high-risk PC patients treated with 70.2/1.8 Gy–72/1.8–2.0 Gy. They reported 10-year PC-specific survival of 84%, and biochemical PFS of 78%, respectively, in the 72 Gy subgroup. They also reported the GU and GI toxicity to increase with an increasing follow-up period of 1–10 years.
In comparison to these three studies [Citation17–19], our results are encouraging in particular if higher dose-escalation of 85–90 Gy [Citation20] would be applied to the IPLs while maintaining de-escalation of the dose in the whole prostate at the current level. In our patients, hormonal therapy was not started until progression and five (17%) patients belonged to the low-risk group. We acknowledge that these factors make a direct comparison of outcomes to other dose-painting studies difficult. Furthermore, our findings suggest that size rather than the number of IPLs matters, but this will have to be confirmed in a larger trial. While smaller MTVs were associated with lower risk for recurrence it would seem prudent to give higher doses than presented in the current study to larger MTVs. Large MTVs have a higher risk for radioresistant and probably hypoxic cells [Citation21] which would justify less dose-escalation to smaller IPLs having less uptake of ACE and presumably less hypoxic cells. While partial volume effect affects more delineation of small IPLs it is comforting that they seem to be controlled by smaller doses than large tumors which show a high uptake ratio against normal prostate. A limitation for use of ACE in treatment planning is its potential sensitivity to androgen depletion, which decreases uptake in the prostate of patients receiving neoadjuvant hormonal therapy. We have seen this phenomenon clinically and restricted therefore enrollment in the current study is only for hormone-naïve patients. Another limitation was the lack of baseline evaluation of urinary, gastrointestinal, and erectile function and second toxicity evaluation at 10 years while at least genitourinary toxicity may increase beyond 5 years [Citation3].
Nevertheless, the low toxicity at 5 years and modern IMRT technique using image guidance make an increase in cumulative toxicity after that time pointless likely compared to the study of Zelefsky et al. where online image guidance was not generally available.
Conclusion
In conclusion, biological guidance for dose-escalated whole prostate RT is feasible with ACE PET/CT and does not seem to compromise outcome or increase late toxicity. A potential advantage of radionuclide rather than MRI-based techniques is easier quantification of the signal, which may allow dose painting by numbers rather than contours. This was reflected in the current study by the fact that higher MTV was associated with recurring tumors. A large MTV is thus likely to mandate a higher dose than less active tumors, which may offer advantages for individual dosimetric planning. Whether our findings can be outperformed by more specific tracers, such as radiolabelled PSMA remains to be seen in future studies.
Supplemental Material
Download MS Word (11.4 KB)Disclosure statement
No potential conflict of interest was reported by the authors.
References
- Viani GA, Stefano EJ, Afonso SL. Higher-than-conventional radiation doses in localized prostate cancer treatment: a meta-analysis of randomized, controlled trials. Int J Radiat Oncol Biol Phys. 2009;74(5):1405–1418.
- Mottet N, Cornford P, van den Bergh RCN, et al. EAU-EANM-ESTRO.ESUR-SIOG Guidelines on Prostate Cancer; 2020.
- Zelefsky MJ, Levin EJ, Hunt M, et al. Incidence of late rectal and urinary toxicities after three-dimensional conformal radiotherapy and intensity-modulated radiotherapy for localized prostate cancer. Int J Radiat Oncol Biol Phys. 2008;70(4):1124–1129.
- Cellini N, Morganti AG, Mattiucci GC, et al. Analysis of intraprostatic failures in patients treated with hormonal therapy and radiotherapy: implications for conformal therapy planning. Int J Radiat Oncol Biol Phys. 2002;53(3):595–599.
- Chen ME, Johnston DA, Tang K, et al. Detailed mapping of prostate carcinoma foci: biopsy strategy implications. Cancer. 2000;89(8):1800–1809.
- Seppälä J, Seppänen M, Arponen E, et al. Carbon-11 acetate PET/CT based dose escalated IMRT in prostate cancer. Radiother Oncol. 2009;93(2):234–240.
- Blake W, Stapleton A, Brown A, et al. A prospective trial of intensity modulated radiation therapy (IMRT) incorporating a simultaneous integrated boost for prostate cancer: long-term outcomes compared with standard image guided IMRT. Phys Imag Radiat Oncol. 2020;15:66–71.
- Barry MJ, Fowler FJ, O’Leary MP, et al. The American Urological Association symptom index for benign prostatic hyperplasia. The measurement committee of the American Urological Association. J Urol. 1992;148(5 Part 1):1549–1557.
- Amling CL, Bergstralh EJ, Blute ML, et al. Defining prostate specific antigen progression after radical prostatectomy: what is the most appropriate cut point? J Urol. 2001;165(4):1146–1151.
- Hofman MS, Lawrentschuk N, Francis RJ, et al. DG; proPSMA study group collaborators. Prostate-specific membrane antigen PET-CT in patients with high-risk prostate cancer before curative-intent surgery or radiotherapy (proPSMA): a prospective, randomised, multicentre study. Lancet. 2020;395(10231):1208–1216.
- Grubmüller B, Baltzer P, Hartenbach S, et al. PSMA ligand PET/MRI for primary prostate cancer: staging performance and clinical impact. Clin Cancer Res. 2018;24(24):6300–6307.
- Oyama N, Akino H, Kanamaru H, et al. 11C-acetate PET imaging of prostate cancer. J Nucl Med. 2002;43(2):181–186.
- Zamboglou C, Sachpazidis I, Koubar K, et al. Evaluation of intensity modulated radiation therapy dose painting for localized prostate cancer using 68Ga-HBED-CC PSMA-PET/CT: a planning study based on histopathology reference. Radiother Oncol. 2017;123(3):472–477.
- Kerkmeijer LGW, Groen VH, Pos FJ, et al. Focal boost to the intraprostatic tumor in external beam radiotherapy for patients with localized prostate cancer: results from the FLAME randomized phase III trial. J Clin Oncol. 2021;39(7):787–796.
- Draulans C, van der Heide UA, Haustermans K, et al. Primary endpoint analysis of the multicentre phase II hypo-FLAME trial for intermediate and high risk prostate cancer. Radiother Oncol. 2020;147:92–98.
- Murray JR, Tree AC, Alexander EJ, et al. Standard and hypofractionated dose escalation to intraprostatic tumor nodules in localized prostate cancer: efficacy and toxicity in the DELINEATE trial. Int J Radiat Oncol Biol Phys. 2020;106(4):715–724.
- Schild MH, Schild SE, Wong WW, et al. A prospective trial of intensity modulated radiation therapy (IMRT) incorporating a simultaneous integrated boost for prostate cancer: long-term outcomes compared with standard image guided IMRT. Int J Radiat Oncol Biol Phys. 2017;97(5):1021–1025.
- Vora SA, Wong WW, Schild SE, et al. Analysis of biochemical control and prognostic factors in patients treated with either low-dose three-dimensional conformal radiation therapy or high-dose intensity-modulated radiotherapy for localized prostate cancer. Int J Radiat Oncol Biol Phys. 2007;68(4):1053–1058.
- Pinkawa M, Gharib A, Schlenter M, et al. Quality of life more than 10 years after radiotherapy for localized prostate cancer-impact of time after treatment and prescription dose. Qual Life Res. 2021;30(2):437–443.
- Koskela K, Palmgren JE, Heikkilä J, et al. Hypofractionated stereotactic body radiotherapy for localized prostate cancer – first Nordic Clinical Experience. Acta Oncol. 2017;56(7):978–983.
- Hara T, Bansal A, DeGrado TR. Effect of hypoxia on the uptake of [methyl-3H]choline, [1-14C] acetate and [18F]FDG in cultured prostate cancer cells. Nucl Med Biol. 2006;33(8):977–984.