Introduction
Consolidation radiotherapy after chemotherapy reduces the risk of relapse for localized Hodgkin lymphoma (HL) [Citation1,Citation2]. However, arrhythmias and conduction disorders are frequent radiation-induced complications of mediastinal HL irradiations [Citation3] which are potentially life-threatening [Citation4]. Adams et al. demonstrated that up to 75% of long-term HL survivors had detectable conduction defects after a median follow-up of 14.3 years [Citation5]. However, the relationship between late development of arrhythmias or conduction disorders and doses delivered to the cardiac conduction substructures has never been studied since delineation atlases did not include cardiac conduction substructures until recently [Citation6].
Volumetric modulated arc therapy (VMAT) combined with deep-inspiration breath-hold (DIBH) is currently considered as a standard-of-care for mediastinal HL irradiation by international guidelines, due to its superior dosimetric sparing of cardiac substructures such as the left anterior descending coronary artery [Citation7,Citation8]. However, this technique increases low dose exposure [Citation9] and the dosimetric impact on the cardiac conduction system has never been thoroughly investigated. In addition, proton therapy is increasingly used in clinical practice for mediastinal HL treatment, based on its theoretical advantage for reducing the risk of second cancers and of cardiac complications [Citation10,Citation11]. The magnitude of the potential dosimetric benefit of proton therapy for conduction node sparing has never been evaluated but, if significant, might also motivate its use for patients with underlying arrhythmias or conduction disorders.
The aim of this study was to evaluate the radiation exposure of cardiac conduction nodes in patients treated for mediastinal HL with DIBH-VMAT and to evaluate the potential dosimetric benefit of intensity modulated proton therapy (IMPT) over VMAT.
Materials and methods
Population
This retrospective observational study included patients treated at the Institut Curie (Paris, France) for localized unfavorable mediastinal HL with standard first line chemotherapy regimens (ABVD or BEACOPP) and subsequent consolidation irradiation with involved-site DIBH-VMAT, according to ILROG recommendations [Citation8]. Radiotherapy records from thirty patients treated between January 2018 and May 2020 were selected from the institutional database by random sampling and anonymized. Median age of included patients was 30 years, 17 patients were women, and ECOG performance status ranged between 0 and 2. While all patients had intermediate stage HL according to EORTC criteria, eight patients initially presented with bulky disease. All patients had mediastinal disease, which was localized in the lower mediastinum (i.e., below the take-off of the left main stem coronary artery [Citation12]) for 22 patients (73.3%) and limited to the upper mediastinum for eight patients (26.7%); 15 patients (50.0%) had additional neck targets and two patients (6.7%) axillary targets. ABVD regimen was delivered to 27 patients and the three other patients received BEACOPP chemotherapy. All patients received a consolidative dose of 30 Gy according to ESMO guidelines.
This study was approved by the Institutional Review Board of the Institut Curie.
VMAT treatment and protontherapy replanning
Patients were immobilized supine. Simulation computed tomography (CT) scans were acquired for all patients without contrast agent injection using 2-mm slices and transferred to the Raystation 10B treatment planning system for DIBH-VMAT planning. Patients were treated to the dose of 30 Gy in 15 fractions. DIBH was controlled with spirometer. Median clinical target volumes (CTV) were defined according to the involved-site radiation therapy (ISRT) standard [Citation8]; median CTV was 324 cc [range: 83–837cc]. Planned target volumes (PTV) were defined with a 5 mm expansion margin around clinical target volumes (CTV). A minimum of 95% of the prescribed dose was planned to be delivered to 95% of the PTV and a maximum of 107% of the prescribed dose could be delivered to 2% of the PTV. Dose constraints to organs-at-risk respected ILROG guidelines [Citation12], including mean heart dose ≤5 Gy, mean dose to the breasts ≤4 Gy, lung V5Gy ≤55%, lung V20Gy ≤30%, mean lung dose ≤10 Gy and thyroid V25Gy ≤62.5%.
Intensity modulated proton therapy (IMPT) with quasi-discrete active scanning [Citation13] was retrospectively re-planned at the National Center for Oncological Hadrontherapy (CNAO, Pavia, Italy) on the initial simulation CT scans, aiming to achieve a similar CTV coverage. Robust optimization with multifield optimization was used for IMPT plan calculations on CTV; a 2 mm isotropic setup error and a 3% range uncertainty were applied. Cardiac motion was not specifically taken into account. Spot spacing was 3 mm with an energy step of 2 mm in water. One to four pencil beam scanning (PBS) fields were planned, depending on the target localization and on the patient anatomy, simulated on a virtual gantry. For complex target volumes, targets could be divided for multifield planning based on the anatomical localization (upper mediastinum, lower mediastinum, neck, axillary, etc.); in cases of diffuse mediastinal targets, a combination of anterior and posterior fields could be used to optimize cardiac and lung sparing [Citation12]; no systematic class solution was used for beam arrangement, which was defined on a case-by-case basis. Use of range shifter depended on the target shape and localization.
Cardiac conduction node delineation
The atrioventricular node (AVN) and the sinoatrial node (SAN) were subsequently delineated on the planning CT scans according to previously published guidelines [Citation6] to ensure reproducibility and homogeneity. According to these guidelines, the SAN was delineated as a 2 cm-diameter sphere, tangent to the external wall of the right atrium, centered at the height of the ascending aorta origin and the AVN was delineated as a 2 cm-diameter sphere centered at the junction between the four cardiac chambers, 1 cm above the last slice where the left atrium was visible.
Statistics
Mean and maximum doses to the AVN, to the SAN and to the whole heart were retrieved from the dose-volume histograms of the VMAT and IMPT plans. Dose were compared between the VMAT and the paired IMPT plans using non-parametric Wilcoxon signed-rank test. Pearson correlation coefficient (r) were calculated between doses to the AVN, to the SAN and to the whole heart. Statistical analyses were performed using R 3.6.1 software.
Results
For VMAT irradiation, the SAN mean dose was 6.6 Gy [range: 0.0–29.4 Gy] and the SAN maximum dose was 11.0 Gy [0.2–30.5 Gy]; the AVN mean dose was 0.9 Gy [0.0–16.7 Gy] and the AVN maximum dose was 1.1 Gy [0.0–19.4 Gy]. IMPT significantly reduced SAN mean dose to 0.3 Gy [0.0–27.1 Gy] (p < 0.01), SAN maximum dose to 2.4 Gy [0.1–31.7 Gy] (p < 0.01), AVN mean dose to 0.02 Gy [0.0–1.1 Gy] (p < 0.01), and AVN maximum dose to 0.05 Gy [0.0–2.8 Gy] (p < 0.01) (,b)). An example of IMPT replanning is provided in . Twenty-two patients (73%) had a mean SAN dose reduction with IMPT greater than 1 Gy and twelve patients (40%) had a mean SAN dose reduction greater than 5 Gy; twelve patients (40%) had a mean AVN dose reduction with IMPT greater than 1 Gy, and four patients (13%) had a mean AVN dose reduction greater than 5 Gy.
Figure 1. Cardiac conduction node exposure during mediastinal Hodgkin lymphoma irradiation with volumetric modulated arc therapy (VMAT) or intensity modulated proton therapy (IMPT) with deep-inspiration breath-hold (DIBH). (a) Mean doses delivered to the heart, to the sino-atrial node (SAN) and to the atrio-ventricular node (AVN). (b) Maximum doses delivered to the heart, to the SAN and to the AVN. Individual values are represented by red spots. (c) Example of conduction node exposure with volumetric modulated arc therapy (VMAT) and intensity modulated proton therapy (IMPT): Sinoatrial node is delineated in green, atrio-ventricular node in blue, and the heart in orange. The CTV, defined according to the involved-site radiation therapy (ISRT) standard, is delineated in red.
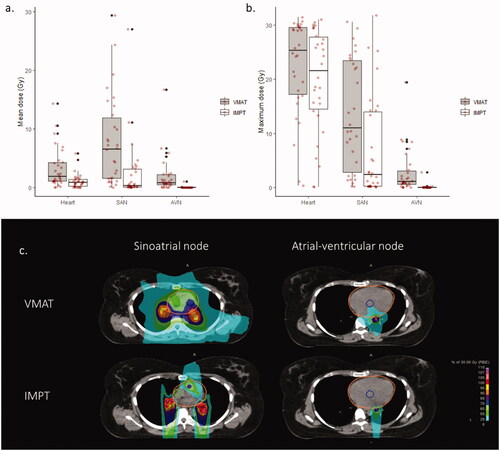
Pearson correlation coefficients between doses to the heart and doses to the SAN or to the AVN are provided in . For VMAT, correlations were strong between mean doses to the heart and to the conduction nodes and weak between maximum doses to the heart and to the conduction nodes. After IMPT replanning, correlations were moderate between mean doses to the heart and to the conduction nodes and unsignificant between maximum doses to the heart and to the conduction nodes.
Table 1. Pearson correlation coefficients (r) between doses to the heart and doses to the atrio-ventricular node (AVN) or to the sinoatrial node (SAN), with volumetric modulated arctherapy (VMAT) or intensity modulated proton therapy (IMPT).
Discussion
This study evaluated the conduction node exposure associated with mediastinal irradiation with DIBH-VMAT for localized unfavorable mediastinal HL patients. VMAT exposes large volumes to low doses and may lead to unintentional overexposure of multiple non-considered potential organs-at-risk, such as cardiac conduction nodes. We observed that VMAT substantially exposed the SAN, with an average mean dose of 7 Gy, more than twice the observed mean heart dose values (around 3 Gy). It could be hypothesized that this observation might be correlated with the high frequency of observed infra-clinical and clinical conduction and rhythmic disorders observed after mediastinal HL irradiation [Citation3–5]. Nevertheless, it should be noted that the SAN is located on the right side of the heart and the exposition might consequently differ based on the laterality of target sites.
The MHD is the most widely used dosimetric parameter; we found that dosimetric parameters of the heart, of the SAN and of the AVN were imperfectly correlated, especially for IMPT and when maximum doses were considered. This observation is concordant with other dosimetric studies focusing on classic cardiac substructures [Citation14]. Recently published trials have demonstrated that dosimetric parameters of specific cardiac substructures were associated with clinical cardiac adverse events [Citation15]; this is yet to be proven for conduction disorders and arrhythmias, but it might also be the case. Manually delineating SAN and NAV is relatively time-consuming, but cardiac auto-segmentation algorithms have shown promising results when substructure dimensions were large enough [Citation16], which is the case for the SAN and the AVN contours. Nevertheless, the bundle of His and the left and right bundle branches are usually considered too small to be identifiable on CT scans and reproducibly delineated [Citation6]. Thus, correlation between ventricular rhythmic disorders and specific cardiac substructure exposure may be more problematic to evaluate, even though ventricular segments could be used as surrogates [Citation17]. In the future, retrospective or prospective studies taking into account conduction system substructures will be needed to obtain normal tissue complication probability (NTCP) models for cardiac conduction disorders, based on dosimetric parameters to the SAN and to the AVN; the limited size of the population did not allow such analysis in our study. Precise dose constraints to the SAN or to the AVN are consequently currently difficult to define; nevertheless, ILROG recommendations to minimize cardiac substructure doses as much as possible, ideally under 5 Gy, may similarly apply for the SAN and the AVN in the meantime. In addition, it is unknown whether the SAN or the AVN has a more important role for conduction disorder development, what can be considered as a clinically relevant dose reduction to the SAN or to the AVN, and which conduction node should be spare in priority. It should be underlined that the development of NTCP models for conduction disorder occurring after HL IMPT will take time, since the worldwide experience of HL IMPT is still limited to this date. While the threshold for a clinically relevant dose reduction is currently difficult to define, we observed that the absolute dosimetric gain with IMPT tended to correlate with the dose to the conduction nodes with VMAT; consequently, the lower the inferior border of the mediastinal CTV was, the more pronounced the dosimetric benefit in favor of IMPT usually was.
We included patients treated with consolidative radiotherapy after a first-line chemotherapy; this population currently represents the majority of irradiated HL patients. In this context, proton therapy is increasingly used, especially for selected young patients [Citation10,Citation11]; we found that proton therapy substantially reduced SAN exposure and delivered virtually no dose to the AVN; one can hypothesize that this might potentially reduce radiation-induced conduction and rhythmic disorders, which would be clinically beneficial for long-term HL survivors. While patient selection for HL proton therapy is currently subject to intense debate [Citation10], it may be suggested that patients with preexisting cardiac arrhythmia or conduction disorders could represent potential candidates for mediastinal HL proton therapy.
In conclusion, we observed that the SAN was substantially exposed during mediastinal HL radiotherapy with DIBH-VMAT; it could be hypothesized that this observation might be correlated with the high frequency of observed infra-clinical and clinical conduction and rhythmic disorders after mediastinal HL irradiation and may justify considering conduction nodes as potential organs-at-risk for future clinical researches. Doses to SAN and AVN are imperfectly correlated with doses to the whole heart and precisely evaluating their exposure might require their delineation. Proton therapy significantly reduces radiation doses to the cardiac conduction nodes; consequently, it might hypothetically reduce the incidence of conduction disorders which are frequent radiation-induced adverse events for HL survivors, and proton therapy may possibly be considered in case of underlying rhythmic or conduction disease.
Acknowledgement
The authors declare that they have no funding and no financial support.
Disclosure statement
No potential conflict of interest was reported by the author(s).
Data availability statement
Research data are stored in an institutional repository and will be shared upon request to the corresponding author
References
- Engert A, Plütschow A, Eich HT, et al. Reduced treatment intensity in patients with early-stage Hodgkin's lymphoma. N Engl J Med. 2010;363(7):640–652.
- André MPE, Girinsky T, Federico M, et al. Early positron emission tomography response-adapted treatment in stage I and II Hodgkin lymphoma: final results of the randomized EORTC/LYSA/FIL H10 trial. J Clin Oncol. 2017;35(16):1786–1794.
- Jaworski C, Mariani JA, Wheeler G, et al. Cardiac complications of thoracic irradiation. J Am Coll Cardiol. 2013;61(23):2319–2328.
- Adams MJ, Lipshultz SE, Schwartz C, et al. Radiation-associated cardiovascular disease: manifestations and management. Semin Radiat Oncol. 2003;13(3):346–356.
- Adams MJ, Lipsitz SR, Colan SD, et al. Cardiovascular status in long-term survivors of Hodgkin’s disease treated with chest radiotherapy. J Clin Oncol. 2004;22(15):3139–3148.
- Loap P, Servois V, Dhonneur G, et al. A radiotherapy contouring atlas for cardiac conduction node delineation. Pract Radiat Oncol. 2021;11(4):e434–e437.
- Filippi AR, Meregalli S, DI Russo A, Fondazione Italiana Linfomi (FIL) Radiotherapy Committee, et al. Fondazione Italiana Linfomi (FIL) expert consensus on the use of intensity-modulated and image-guided radiotherapy for Hodgkin's lymphoma involving the mediastinum. Radiat Oncol. 2020;15(1):62.
- Specht L, Yahalom J, Illidge T, ILROG, et al. Modern radiation therapy for Hodgkin lymphoma: field and dose guidelines from the international lymphoma radiation oncology group (ILROG). Int J Radiat Oncol Biol Phys. 2014;89(4):854–862.
- Qiu J, Liu Z, Yang B, et al. Low-dose-area-constrained helical tomotherapy-based whole breast radiotherapy and dosimetric comparison with tangential field-in-Field IMRT [Internet]. Biomed Res Int. 2013;2013:513708.
- Loap P, De Marzi L, Mirandola A, et al. Development and implementation of proton therapy for Hodgkin lymphoma: challenges and perspectives. Cancers. 2021;13(15):3744.
- Loap P, Mirandola A, De Marzi L, et al. Current situation of proton therapy for Hodgkin lymphoma: from expectations to evidence. Cancers. 2021;13(15):3746.
- Dabaja BS, Hoppe BS, Plastaras JP, et al. Proton therapy for adults with mediastinal lymphomas: the international lymphoma radiation oncology group guidelines. Blood. 2018;132(16):1635–1646.
- Mirandola A, Molinelli S, Vilches Freixas G, et al. Dosimetric commissioning and quality assurance of scanned ion beams at the Italian National Center for oncological hadrontherapy. Med Phys. 2015;42(9):5287–5300.
- Hoppe BS, Bates JE, Mendenhall NP, et al. The meaningless meaning of mean heart dose in mediastinal lymphoma in the modern radiation therapy era. Pract Radiat Oncol. 2019;10(3):e147–e154.
- Atkins KM, Chaunzwa TL, Lamba N, et al. Association of left anterior descending coronary artery radiation dose with major adverse cardiac events and mortality in patients with non-small cell lung cancer. JAMA Oncol. 2021;7(2):206–219.
- Loap P, Tkatchenko N, Nicolas E, et al. Optimization and auto-segmentation of a high risk cardiac zone for heart sparing in breast cancer radiotherapy. Radiother Oncol J Eur Soc Ther Radiol Oncol. 2020;153:146–154.
- Duane F, Aznar MC, Bartlett F, et al. A cardiac contouring atlas for radiotherapy. Radiother Oncol. 2017;122(3):416–422.