Abstract
Background
Definitive external beam radiotherapy (EBRT) is an unusual treatment of unresectable soft-tissue sarcomas (STS). Recent technical innovations and physical advantages of particle therapies may improve results of this therapeutic option. The role of this review was to report the clinical results of photon- and particle-based EBRT in unresectable STS.
Material and Methods
We performed a systematic review of the literature on Pubmed database to identify studies investigating the efficacy and safety of EBRT. The primary endpoint was local control (LC) and secondary endpoints were progression-free survival (PFS), overall survival (OS) and adverse events in a subset of patients with gross disease STS.
Results
We identified 29 studies involving 1409 patients (pts) evaluating photon (n = 18; 956 pts), proton (n = 1; 21 pts), carbon ion (n = 2; 152 pts), neutron (n = 7; 259 pts) or pion (n = 1; 21 pts) therapy. Definitive EBRT achieves valuable 5-year LC rates of 28–73% with photon and 52–69% with particle therapies. Most local failures (66–100%) occurred within 3 years. Long-term disease control can be achieved in a fraction of patients, with 5-year PFS and OS of 0–39% and 24.7–63%, respectively. The rate of severe adverse events was highly variable with photons, <15% in proton and carbon ion therapy, whereas 25 to 50% of patients treated with neutrons and pions presented severe AE. While a dose higher or equal 64 Gy seem to improve the prognosis, delivering a dose higher or equal 68 Gy dramatically increases severe adverse events.
Conclusion
Definitive EBRT with dose 64–66 Gy seems to be a safe and efficient treatment for unresectable STS. Future clinical trials should assess the potential of biomarkers of response, thus identifying patients that could benefit from local treatment.
Introduction
Soft tissue sarcomas (STS) are rare and heterogeneous malignancies. Although the annual incidence is not precisely known, it has been suggested that it represents 1% of newly diagnosed adult malignant tumors [Citation1], with a yearly crude incidence in Europe of 4.7 per 100,000 person [Citation2]. Surgery remains the only mean of cure, improving both local control and overall survival [Citation3]. Unfortunately, adequate excision can be limited by the presence of major neurovascular structures, leading to 15 to 60% inoperable or incompletely resected sarcoma [Citation4–7]. Other factors such as co-morbidities or patients’ refusal may limit the decision whether to perform major surgery. Co-morbidities are common as 42% of STS are diagnosed in elderly patients [Citation8]. Due to sarcoma radioresistance, requiring high dose to achieve local control [Citation9,Citation10], external beam radiotherapy (EBRT) is uncommonly considered as curative option.
In past decades, technical innovations such as image-guided radiotherapy (IGRT) and intensity-modulated radiotherapy (IMRT) have enabled better target coverage while lowering the dose delivered to organs at risk. The use of particle therapies, such as proton therapy (PT) or carbon ion radiotherapy (CIRT), seems to be promising. Indeed, they better spare adjacent organs due to the absence of exit dose beyond the Bragg peak, thus allowing dose escalation. Moreover, particles such as carbon ions with high linear energy transfer (LET) have greater relative biological effectiveness (RBE) than that of photons and protons. The higher biologically effective dose could thus overcome tumor radioresistance.
Nevertheless, recent guidelines do not consider definitive RT as a first line therapeutic option in unresectable STS [Citation11]. The purpose of the present work was to identify publications evaluating efficacy and safety of definitive photon- or particle-based EBRT used in this indication.
Material and methods
Data sources and study selection
We performed a systematic review following the Preferred Reporting Items for Systematic Reviews and Meta-Analysis (PRISMA) statement guidelines. PubMed database was searched with the following search string: ("soft tissue neoplasms"[MeSH Terms] OR "sarcoma"[MeSH Terms] OR sarcom* OR fibrosarcom* OR neurofibrosarcom* OR leiomyosarcom* OR liposarcom* OR myxoid liposarcom* OR lymphangiosarcom* OR rhabdomyosarcom*) AND (“soft tissue”) AND (("antineoplastic protocols"[MeSH Terms]) OR (chemoradiotherap*) OR (chemo-radiotherap*) OR (radiochemotherap*) OR (radio-chemotherap*) OR (radiotherap*) OR ("radiotherapy"[MeSH Terms]) OR ("radiation therapy") OR (radiation therap*) OR (hadron*) OR ("Protons/therapeutic use"[MeSH]) OR ("Neutrons/therapeutic use"[MeSH]) OR pions OR pion OR borons OR boron OR ("neutron beam") OR ("proton beam") OR ("proton beams") OR ("carbon ion") OR ("carbon ions") OR ("heavy ion") OR ("heavy ions") OR ("light ion") OR ("light ions")) AND (("english"[Language]) OR "french"[Language]).
Then, all titles or abstracts containing “definitive radiation therapy”, “definitive radiotherapy”, “exclusive”, “unresectable”, “unresected”, “non-resectable”, “without surgery”, or “gross residual disease” were identified. The references of included studies were screened for eligibility. The last search was run on October 6th, 2021. Each study was screened and reviewed for eligibility by two independent authors (BA, WW) and review by a third in case of disagreement (MPS).
Inclusion criteria were publications in French or English language, in unresected or grossly resected STS adult patients, with available information on radiotherapy modalities and clinical monitoring whatever the type of particle. Exclusion criteria were publications including less than 5 unresected or grossly resected STS patients, pediatric series, articles reporting global clinical results without separate analysis between bone sarcomas/STS, or between R0/R1 resected and R2/unresected patients, conference abstracts and articles with no full text available.
Outcomes
The primary outcome was local control (LC). Secondary outcomes included local recurrence-free survival (LRFS), progression-free survival (PFS), overall survival (OS) and adverse events (AE). Most studies considered dRT as a pseudo-curative treatment and thus used the name disease- instead of progression-free survival, but their definition of this outcome was the usual one of PFS. To generate comparative curves, for studies which did not specify clinical outcomes at 6 months, 1, 2, 3, 4 and/or 5 years, data were extracted from published plots using Web Plot Digitizer (https://automeris.io/WebPlotDigitizer) to convert datapoints into numerical values.
Data extraction
Data were extracted independently and verified by two authors (BA, WW) using a predefined data extraction form. For each study, we extracted the following data: authors’ name, year of publication, study design, median follow-up, age, gender, total number of patients, number of unresected or grossly resected patients, location of the tumor, histological diagnosis and FNCLCC grade, severe adverse events, radiation therapy schedule information and type of particle used.
Results
Identified studies
PubMed database searches yielded 6521 unique citations. All abstracts from PubMed were retrieved, and using pubmed.mineR package we selected 431. After full-text review and evaluation of references, 29 unique citations, with a total of 1409 patients (pts), from 1983 to 2020 were included in this review ( and ). The characteristics of eligible studies, their dose/fractionation data, and their main clinical results are reported in . After data extraction from text and/or published curves, we generated comparative curves of LC (), PFS () and OS () according to the type of particle used.
Figure 3. Local control (LC) in the included studies according to the type of particle used and the number of unresected patients.
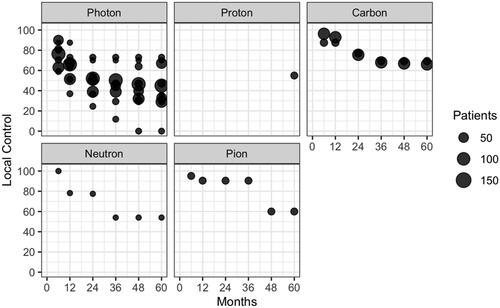
Figure 4. Progression-Free Survival (PFS) in the included studies according to the type of particle used and the number of unresected patients.
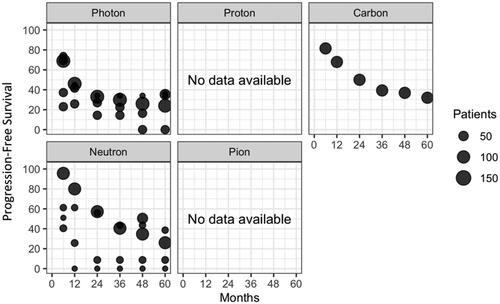
Figure 5. Overall Survival (OS) in the included studies according to the type of particle used and the number of unresected patients.
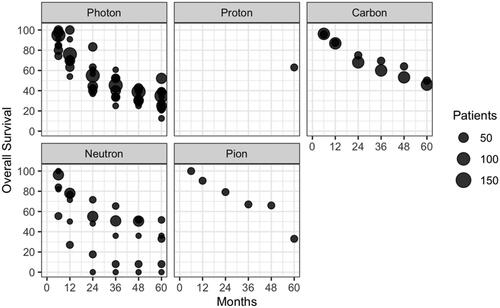
Table 1. Characteristics of eligible studies.
Efficacy of EBRT for unresected or R2 STS
Our analysis included 18 (956 pts), 1 (21 pts), 2 (152 pts), 7 (259 pts) and 1 (21 pts) publications assessing photons, protons, carbon ions, neutrons and negative pi-mesons based radiotherapy respectively ().
Because of the limited number and the heterogeneity of publications, we did not perform post-hoc comparison of clinical outcomes but reported them, as initially planned, according to the type of particle (). When considering the most used techniques worldwide, photons, protons and carbonions achieved 5-year LC of 28–73%, 55% and 65–69%, respectively.
Kepka et al. published the largest series with 112 patients treated with photon-based radiotherapy with a median dose of 64 Gy, and reported 5-year LC, DFS and OS of 45%, 24% and 35%, respectively [Citation9]. The largest series of carbon ions reported 128 patients treated with a median dose of 70.4 GyE [Citation12], and reported 5-year LC, DFS and OS of 65%, 32% and 46%, respectively. In this series, no factor was significantly correlated with LC. In contrast, OS was significantly related to LC and tumor size ≥500cc (p = 0.012 and 0.002, respectively).
There was a large heterogeneity of dose prescription, particularly in photon-based irradiation, partly due to the technical evolution in radiation techniques. As an example, the recent publication of Paik et al investigated the role of 1 to 5 fractions stereotactic body radiotherapy (SBRT) for unresectable STS of the trunk. For the 18 patients included, authors reported 5-year LC and OS of 47% and 28%, respectively [Citation13].
Dose-response relationship
Radiation dose higher or equal to 63 Gy was associated with better prognosis in unresectable STS (at 5 years: LC 60% versus 22%, p = 0.02; DFS 36% versus 10%, p = 0.007; OS 52% versus 14%, p = 0.002) [Citation9]. DeLaney et al. reported that patients resected with positive margins and receiving more than 64 Gy had higher LC, DFS and OS (p < 0.04) [Citation10]. This dose threshold was not confirmed by Slater et al. [Citation14] nor for protons by Willers et al. (at 5 years: LRC 73 versus 65%, p = 0.72; OS 76 versus 73%, p = 0.72) [Citation15]. Imai et al. failed to demonstrate local or survival benefit of escalating the dose above 70.4 GyE with carbon ions (5-year 46 versus 50%, p = 0.45) [Citation12]. Nevertheless, While Serizawa et al. reported that more than 90% of CTV was covered by prescribed isodose [Citation16], other studies did not report their respect of dose constraint.Safety of EBRT
The rate of grade ≥3 AE was highly variable with photons, protons, and carbon-ion therapies alone. Severe acute and late skin toxicities were 6–18% [Citation9,Citation10,Citation15] and 1–5% [Citation9,Citation10,Citation13–15,Citation17]. Chronic visceral complications such as stenosis, fistula or bleeding were 1–9% [Citation9,Citation14,Citation16,Citation18].
Neutrons and negative pi-meson therapy induced severe late complications, mainly represented by 3-44% skin toxicity [Citation19–23] and 1–14% visceral obstruction [Citation19,Citation21,Citation23–25], with a grade 5 pneumonitis [Citation24].
Major toxicities dramatically increased when the delivered dose exceeded 66 Gy. Dose thresholds of 68 Gy and 70 Gy were respectively reported by Kepka et al. (26% versus 8% severe AE) [Citation9] and Slater et al. (28% versus 2% severe AE) [Citation14].
EBRT and systemic treatment combinations
Seven of the included studies evaluated a combination of photon EBRT with radiosensitizer or chemotherapy [Citation26–32]. In a small sample (n = 11), promising 5-year DFS and OS of 34% were reported after EBRT and concomitant ifosfamide [Citation26]. Rhomberg et al. [Citation27] evaluated a combination of razoxane and RT in unresectable STS in a randomized phase II study. This schedule improved LC from 30.5% to 64% at 4 years (p < 0.05). The combination of vindesine and RT led to a median OS of 17.5 to 29 months [Citation28,Citation29]. Concomitant recombinant interleukine-2 achieved a median LC of 11.1 months in head and neck angiosarcomas [Citation30].
These combinations induced important acute grade ≥3 adverse events, with 9–40% acute leucopenia [Citation26,Citation28,Citation29], 3.5–35% acute skin toxicity [Citation26,Citation30,Citation32], 60% (n = 3/5) dysphagia for angiosarcoma of the thyroid [Citation29], and a toxic death by pulmonary embolism [Citation28].
Discussion
While surgery remains the standard of care of local STS, this review shows that definitive RT is still a relevant treatment option for unresectable cases. Since gross R2 resected and unresected patients are both consider locally advanced, have far worse prognosis than R0/R1 resected patients [Citation33], and undergo the same treatment with same challenges, they are usually pooled together [Citation14,Citation27,Citation34,Citation35]. To our knowledge, no direct prognostic comparison was reported between these two gross tumor situations. We thus decided to include both. On the one hand, controlling gross tumor locally is essential in STS to prevent local complications such as bleeding and pain, and to preserve patient’s quality of life. On the other hand, keeping in mind LRFS, PFS and OS data is important since long-term disease control can be achieved and are frequently used in studies evaluating systemic treatments.
EBRT achieves good results on long-term local control, with a 5-year rate of 28–73% with photons and 52–70% with particle therapies (proton 55%, carbon 65–69%, neutron 52%, pion 60%). Due to the size and the heterogeneity of the studies, direct comparisons and pooled analyses could not be performed. Local recurrences usually occur within 3 years regardless of the radiotherapy technique [Citation9,Citation13] or the type of particle used [Citation12,Citation16,Citation20,Citation25], and is possibly related to OS [Citation12,Citation15,Citation36]. Long-term disease control can be achieved, with 5-year PFS of 0–36%, 32% and 0–39% with photons, carbon ions and neutrons, respectively (no data available for protons and pions). Five-year OS was 24.7–52.2% in photon-based therapy, 63% using protons, 46–50% using carbons, 0–33% using neutron and 33% using pion therapy. In contrast, in post- and pre-operative radiotherapy, O’Sullivan et al. reported far better prognosis, with 5-year LC, recurrence-free survival and OS of 92–93%, 58–59%, 67–73% [Citation37]. Yang et al. reported 10-year local failure rates of 0 and 22% in patients with high grade resected tumors who beneficiated or not of adjuvant RT (p = 0.0001) [Citation38].
The major issue remains the optimal total dose and fractionation to achieve high local control rates. A dose higher or equal 64 Gy was associated with a better prognosis [Citation9,Citation10], while there was no benefit escalating dose higher than 70.4 GyE [Citation12]. In unresectable bone or STS treated with carbon ions, Kamada et al. also reported higher LC in patients receiving at least 64 GyE (p = 0.035) [Citation39]. Nevertheless, only one study provided dosimetric data [Citation16], making conclusions about dose-effect relationship more difficult to interpret. Furthermore, most treatments were performed in 2 D or 3 D-CRT and delivered without IGRT, which likely led to actual CTV undercoverage.
Proximity of critical organs is a major limitation for both surgical excision and radiotherapy. Technological innovations such as IGRT, IMRT or SBRT have been continuously improving the precision of dose delivery, thus allowing to deliver either a higher dose to the tumor and to better spare OAR [Citation40]. In a study published by Folkert et al. in STS treated with limb-sparing surgery and adjuvant RT, IMRT reduced both local recurrence (HR = 0.46; p = 0.02) and acute grade ≥2 radiation dermatitis (48,7% versus 31.5%; p = 0.002) compared with conventional RT [Citation41].
For charged particles, the deposited dose increases with depth to a maximum at the end of their path, called the Bragg Peak. Compared to photon therapy, this ballistic advantage may be useful to efficiently treat unresectable sarcoma by limiting dose exposure to surrounding OAR. In addition, high LET particle therapies have several advantages compared with protons through their relative biological effectiveness (RBE) and oxygen enhancement ratio (OER). Despite variations according to the fractionation, LET and energy of the beam, RBE of carbon, neutron and negative pi-meson are around 3 [Citation42], 3-4 [Citation42] and 1.5 [Citation43], respectively. Photon OER is approximately of 2.5-3.0 [Citation44] whereas OER of carbon ions, neutrons and pions are around 1-2 [Citation45], 1.6 [Citation46] and 2.2 [Citation43], respectively.
To date, no prospective study has been published comparing photon and particle therapies, and particle therapies between themselves for unresectable STS. ETOILE (NCT02838602) is a randomized controlled trial still recruiting. Its aim is to compare carbon ion therapy versus conventional radiotherapy in the treatment of gross radioresistant tumors, including STS (source www.clinicaltrials.gov).
More recently, development of spatially fractionated radiotherapy (SFRT) allows to efficiently spare normal tissues while treating bulky tumors [Citation47]. This type of treatment consists of alternating tumor sub-volumes treated at very high or very low doses, typically in a single fraction. This highly non-uniform dose could increase response rate by inducing bystander effect [Citation48,Citation49] and vascular remodeling that improves tumor oxygenation [Citation50]. On the other hand, the steep gradient in the high dose regions could better spare OAR. Grams et al. [Citation47] recently reported preliminary results of this technique for two palliative patients. One had a 3680 cm3 abdominal leiomyosarcoma treated with18 Gy on 32 high dose regions within the GTV and presented a partial response one week after treatment [Citation47].
Systemic treatments alone achieve similar survival results in this poor prognosis indication. Median PFS of non-metastatic locally advanced sarcomas treated with Doxorubicin with or without Ifosfamide was 7.3 and 5.4 months, respectively [Citation51]. Publications evaluating first-line anthracycline-based treatment reported a median OS between 12.8 and 19 months [Citation51–53], but less than 20% of the patients included in these series were non-metastatic.
From a radiobiological point of view, combining systemic treatment and EBRT could be a valuable option to improve the therapeutic ratio. While combining RT and systemic treatment increased response rate [Citation27], severe acute adverse events could reach 35 to 60% [Citation28–30] and should thus be performed in selected patients. Jakob et al. performed concomitant chemoradiotherapy with temozolomide in 15 locally advanced unresectable non-metastatic STS. After this induction treatment, nine out of 15 tumors (60%) were amenable to surgery, with complete resection achieved in 7 patients, and R1 resection in 2 patients [Citation54]. Trabectedin, another conventional chemotherapy agent, has already proven its efficacy in advanced STS [Citation55] and could be another candidate. Its combination with RT in unresectable STS is currently evaluated in a phase I-II trial (TRASTS; NCT02275286; source www.clinicaltrials.gov). A multicentric phase I trial published in early 2022 reported a median PFS of 6.5 months in 10 inoperable STS treated with EBRT and concomitant Sunitinib [Citation56]. Grade 3 adverse events were mainly hematological and reached 58% [Citation56]. Poly-(ADP-ribose)-polymerase inhibitors (PARPi) are other candidates. Indeed, these molecules inactivate and trap PARP1 onto DNA damaged site, converting single-strand breaks into potentially lethal DNA double-strand breaks. In a preclinical study, Lee et al. reported that the addition of Olapararib (PARPi) to RT increased DNA damage, apoptosis and cell death in Ewing sarcoma cell lines [Citation57]. In STS, a phase Ib (RADIOSARP; NCT02787642) is evaluating the combination of Olaparib with concomitant radiotherapy (source www.clinicaltrials.gov).
A potential limitation of this systematic review is the absence of pooled analysis. This is due to heterogeneity in survival data reporting, to the more or less stringent selection of histological subtype and tumor location, and to the potential use of various concomitant treatments in many studies. Nevertheless, our work provides a detailed state of the art of treatment regimens according to distinct clinical situations, as well as their expected results.
Conclusion
Definitive photon, proton and carbon ion therapies are safe options of treatment of unresectable STS and can achieve long-term disease control in almost a third of patients. Delivering dose higher than or equal to 64 Gy is required, without exceeding 66 Gy. The combination of systemic treatment and RT induces severe adverse events, should currently be restricted to fit patients. Future trials should assess the potential of biomarkers of response to identify patients or histological subtypes that could benefit most from definitive radiotherapy.
Author contributions
Benoît ALLIGNET: Conceptualization, Methodology, Investigation, Data curation,
Visualization, Writing- Original draft preparation. Marie-Pierre SUNYACH: Conceptualization, Writing- Reviewing and Editing. Xavier GEETS: Writing- Reviewing and Editing. Waisse WAISSI: Conceptualization, Supervision, Methodology, Investigation, Writing-Reviewing and Editing.
Disclosure statement
The author declare that they have no competing interest.
Data availability statement
Data can be made available upon requested with appropriate approval by the investigator team and research administrative offices. Data sharing will be subject to appropriate data transfer agreements. Data requests can be made to the corresponding author after manuscript publication.
Additional information
Funding
References
- Howlader N, Noone A, Krapcho M, et al. SEER cancer statistics review, 1975-2018. Bethesda (MD): National Cancer Institute; 2018.
- Stiller CA, Trama A, Serraino D, RARECARE Working Group, et al. Descriptive epidemiology of sarcomas in Europe: Report from the RARECARE project. Eur J Cancer. 2013;49(3):684–695.
- Novais EN, Demiralp B, Alderete J, et al. Do surgical margin and local recurrence influence survival in soft tissue sarcomas? Clin Orthop Relat Res. 2010;468(11):3003–3011.
- Catton CN, O'Sullivan B, Kotwall C, et al. Outcome and prognosis in retroperitoneal soft tissue sarcoma. Int J Radiat Oncol Biol Phys. 1994;29(5):1005–1010.
- Lewis JJ, Leung D, Woodruff JM, et al. Retroperitoneal soft-tissue sarcoma: analysis of 500 patients treated and followed at a single institution. Ann Surg. 1998;228(3):355–365.
- Kim JK, Verma N, McBride S, et al. Patterns of radiotherapy use and outcomes in head and neck soft-tissue sarcoma in a National Cohort. Laryngoscope. 2020;130(1):120–127.
- Martin E, Coert JH, Flucke UE, et al. A nationwide cohort study on treatment and survival in patients with malignant peripheral nerve sheath tumours. Eur J Cancer. 2020;124:77–87.
- Howlader N, Noone A, Krapcho M, et al. SEER cancer statistics review, 1975-2017. Bethesda, MD: National Cancer Institute. 2020. Available from: https://seer.cancer.gov/csr/1975_2017/
- Kepka L, DeLaney TF, Suit HD, et al. Results of radiation therapy for unresected soft-tissue sarcomas. Int J Radiat Oncol Biol Phys. 2005;63(3):852–859.
- DeLaney TF, Kepka L, Goldberg SI, et al. Radiation therapy for control of Soft-Tissue sarcomas resected with positive margins. Int J Radiat Oncol Biol Phys. 2007;67(5):1460–1469.
- Gronchi A, Miah AB, Dei Tos AP, ESMO Guidelines Committee, EURACAN and GENTURIS. Electronic address: [email protected], et al. Soft tissue and visceral sarcomas: ESMO-EURACAN-GENTURIS Clinical Practice Guidelines for diagnosis, treatment and follow-up⋆ ⋆. Ann Oncol. 2021;32(11):1348–1365.
- Imai R, Kamada T, Araki N, Working Group for Carbon Ion Radiotherapy for Bone and Soft Tissue Sarcomas. Carbon ion radiotherapy for unresectable localized axial soft tissue sarcoma. Cancer Med. 2018;7(9):4308–4314.
- Paik EK, Kim MS, Jang WI, et al. Benefits of stereotactic ablative radiotherapy combined with incomplete transcatheter arterial chemoembolization in hepatocellular carcinoma. Radiat Oncol. 2016;11(1):8.
- Slater JD, McNeese MD, Peters LJ. Radiation therapy for unresectable soft tissue sarcomas. Int J Radiat Oncol Biol Phys. 1986;12(10):1729–1734.
- Willers H, Hug EB, Spiro IJ, et al. Adult soft tissue sarcomas of the head and neck treated by radiation and surgery or radiation alone: Patterns of failure and prognostic factors. Int J Radiat Oncol Biol Phys. 1995;33(3):585–593.
- Serizawa I, Kagei K, Kamada T, et al. Carbon ion radiotherapy for unresectable retroperitoneal sarcomas. Int J Radiat Oncol Biol Phys. 2009;75(4):1105–1110.
- Matsumoto K, Imai R, Kamada T, the Working Group for Bone and Soft Tissue Sarcomas, et al. Impact of carbon ion radiotherapy for primary spinal sarcoma. Cancer. 2013;119(19):3496–3503.
- Feng M, Murphy J, Griffith KA, et al. Long-term outcomes after radiotherapy for retroperitoneal and deep truncal sarcoma. Int J Radiat Oncol Biol Phys. 2007;69(1):103–110.
- Schmitt G, Schnabel K, Sauerwein W, et al. Neutron and neutron-boost irradiation of soft tissue sarcomas: a 4.5 year analysis of 139 patients. Radiother Oncol. 1983;1(1):23–29.
- Duncan W, Arnott SJ, Jack WJL. The edinburgh experience of treating sarcomas of soft tissues and bone with neutron irradiation. Clin Radiol. 1986;37(4):317–320.
- Pelton JG, del Rowe JD, Bolen JW, et al. Fast neutron radiotherapy for soft tissue sarcomas university of Washington experience and review of the worldʼs literature. Am J Clin Oncol. 1986;9(5):397–400.
- Pickering DG, Stewart JS, Rampling R, et al. Fast neutron therapy for soft tissue sarcoma. Int J Radiat Oncol Biol Phys. 1987;13(10):1489–1495.
- Prott FJ, Haverkamp U, Willich N, et al. Results of fast neutron therapy ten years of fast neutron therapy in munster. Bulletin Du Cancer/Radiotherapie. 1996;83:115s–121s.
- Schwartz DL, Einck J, Bellon J, et al. Fast neutron radiotherapy for soft tissue and cartilaginous sarcomas at high risk for local recurrence. Int J Radiat Oncol Biol Phys. 2001;50(2):449–456.
- Greiner RH, Munkel G, Blattmann H, et al. Conformal radiotherapy for unresectable retroperitoneal soft tissue sarcoma. Int J Radiat Oncol Biol Phys. 1992;22(2):333–341.
- Eckert F, Matuschek C, Mueller A, et al. Definitive radiotherapy and Single-Agent radiosensitizing ifosfamide in patients with localized, irresectable soft tissue sarcoma: a retrospective analysis. Radiat Oncol. 2010;5:55.
- Rhomberg W, Hassenstein EOM, Gefeller D. Radiotherapy vs. radiotherapy and razoxane in the treatment of soft tissue sarcomas: Final results of a randomized study. Int J Radiat Oncol Biol Phys. 1996;36(5):1077–1084.
- Rhomberg W, Eiter H, Schmid F, et al. Combined vindesine and razoxane shows antimetastatic activity in advanced soft tissue sarcomas. Clin Exp Metastasis. 2008;25(1):75–80.
- Rhomberg W, Wink A, Pokrajac B, et al. Treatment of vascular soft tissue sarcomas with razoxane, vindesine, and radiation. Int J Radiat Oncol Biol Phys. 2009;74(1):187–191.
- Ohguri T, Imada H, Nomoto S, et al. Angiosarcoma of the scalp treated with curative radiotherapy plus recombinant interleukin-2 immunotherapy. Int J Radiat Oncol Biol Phys. 2005;61(5):1446–1453.
- Goffman T, Tochner Z, Glatstein E. Primary treatment of large and massive adult sarcomas with iododeoxyuridine and aggressive hyperfractionated irradiation. Cancer. 1991;67(3):572–576.
- Kinsella TJ, Glatstein E. Clinical experience with intravenous radiosensitizers in unresectable sarcomas. Cancer. 1987;59(5):908–915.
- Bilgeri A, Klein A, Lindner LH, et al. The effect of resection margin on local recurrence and survival in high grade soft tissue sarcoma of the extremities: How far is far enough? Cancers (Basel. 2020;12(9):2560–2513.
- Svoboda VHJ, Krawczyk J. Management of soft tissue sarcomas in portsmouth 1965–1985. Clin Oncol. 1991;3(3):162–167.
- Eckert F, Matuschek C, Mueller AC, et al. Definitive radiotherapy and Single-Agent radiosensitizing ifosfamide in patients with localized, irresectable soft tissue sarcoma: a retrospective analysis. Radiat Oncol. 2010;5(1):6.
- Fayda M, Aksu G, Yaman Agaoglu F, et al. The role of surgery and radiotherapy in treatment of soft tissue sarcomas of the head and neck region: Review of 30 cases. J Craniomaxillofac Surg. 2009;37(1):42–48.
- O'Sullivan B, Davis A, Turcotte R, et al. Five-year results of a randomized phase III trial of pre-operative vs post-operative radiotherapy in extremity soft tissue sarcoma. J Clin Oncol. 2004;22(14_suppl):9007–9007.
- Yang JC, Chang AE, Baker AR, et al. Randomized Prospective Study of the Benefit of Adjuvant Radiation Therapy in the Treatment of Soft Tissue Sarcomas of the Extremity. Clin Oncol. 1998;16:197–203.
- Kamada T, Tsujii H, Tsuji H, Working Group for the Bone and Soft Tissue Sarcomas, et al. Efficacy and safety of carbon ion radiotherapy in bone and soft tissue sarcomas. J Clin Oncol. 2002;20(22):4466–4471.
- Nutting CM, Morden JP, Harrington KJ, et al. Parotid-sparing intensity modulated versus conventional radiotherapy in head and neck cancer (PARSPORT): a phase 3 multicentre randomised controlled trial. The Lancet Oncology. 2011;12(2):127–136.
- Folkert MR, Singer S, Brennan MF, et al. Comparison of local recurrence with conventional and intensity-modulated radiation therapy for primary soft-tissue sarcomas of the extremity. J Clin Oncol. 2014;32(29):3236–3241.
- Kanai T, Endo M, Minohara S, et al. Biophysical characteristics of HIMAC clinical irradiation system for heavy-ion radiation therapy. Int J Radiat Oncol Biol Phys. 1999;44(1):201–210.
- Skarsgard LD, Palcic B, Douglas BG, et al. Radiobiology of pions at TRIUMF. Int J Radiat Oncol Biol Phys. 1982;8(12):2127–2132.
- Robert Grimes D, Partridge M. A mechanistic investigation of the oxygen fixation hypothesis and oxygen enhancement ratio. Biomed Phys Eng Express. 2015;1(4):045209.
- Furusawa Y, Fukutsu K, Aoki M, et al. Inactivation of aerobic and hypoxic cells from three different cell lines by accelerated 3He-, 12C- and 20Ne-Ion beams. Radiat Res. 2000;154(5):485–496.
- Hall EJ, Zaider M, Bird R, et al. Radiobiological studies with therapeutic neutron beams generated by p + leads to Be or d + leads to Be. Br J Radiol. 1982;55(657):640–644.
- Grams MP, Owen D, Park SS, et al. VMAT grid therapy: a widely applicable planning approach. Pract Radiat Oncol. 2021;11(3):e339–e347.
- Asur RS, Sharma S, Chang CW, et al. Spatially fractionated radiation induces cytotoxicity and changes in gene expression in bystander and radiation adjacent murine carcinoma cells. Radiat Res. 2012;177(6):751–765.
- Butterworth KT, Ghita M, McMahon SJ, et al. Modelling responses to spatially fractionated radiation fields using preclinical image-guided radiotherapy. Br J Radiol. 2017;90(1069):20160485.
- Griffin RJ, Koonce NA, Dings RPM, et al. Microbeam radiation therapy alters vascular architecture and tumor oxygenation and is enhanced by a galectin-1 targeted anti-Angiogenic peptide. Radiat Res. 2012;177(6):804–812.
- Tap WD, Papai Z, Van Tine BA, et al. Doxorubicin plus evofosfamide versus doxorubicin alone in locally advanced, unresectable or metastatic soft-tissue sarcoma (TH CR-406/SARC021): an international, multicentre, open-label, randomised phase 3 trial. Lancet Oncol. 2017;18(8):1089–1103.
- Judson I, Verweij J, Gelderblom H, et al. Doxorubicin alone versus intensified doxorubicin plus ifosfamide for first-line treatment of advanced or metastatic soft-tissue sarcoma: a randomised controlled phase 3 trial. Lancet Oncol. 2014;15(4):415–423.
- Seddon B, Strauss SJ, Whelan J, et al. Gemcitabine and docetaxel versus doxorubicin as first-line treatment in previously untreated advanced unresectable or metastatic soft-tissue sarcomas (GeDDiS): a randomised controlled phase 3 trial. Lancet Oncol. 2017;18(10):1397–1410.
- Jakob J, Wenz F, Dinter DJ, et al. Preoperative Intensity-Modulated radiotherapy combined with temozolomide for locally advanced Soft-Tissue sarcoma. Int J Radiat Oncol Biol Phys. 2009;75(3):810–816.
- Demetri GD, Von Mehren M, Jones RL, et al. Efficacy and safety of trabectedin or dacarbazine for metastatic liposarcoma or leiomyosarcoma after failure of conventional chemotherapy: Results of a phase III randomized multicenter clinical trial. J Clin Oncol. 2016;34(8):786–793.
- Sunyach M-P, Penel N, Montané L, et al. Sunitinib with concomitant radiation therapy in inoperable sarcomas: Final results from the dose escalation and expansion parts of a multicenter phase I study. Radiother Oncol. 2022;168:95–103.
- Lee HJ, Yoon C, Schmidt B, et al. Combining PARP-1 inhibition and radiation in ewing sarcoma results in lethal DNA damage. Mol Cancer Ther. 2013;12(11):2591–2600.
- Tepper JE, Suit HD. Radiation therapy alone for sarcoma of soft tissue. Cancer. 1985;56(3):475–479.
- Patrício MB, Trindade MC, Santos F, et al. The role of radiotherapy in soft tissue sarcomas. Retrospective study of 115 cases treated from 1979 to 1988. Acta Médica Portuguesa 1994;7 Suppl 1:25–9.
- Smith KB, Indelicato DJ, Knapik JA, et al. Definitive radiotherapy for unresectable pediatric and young adult nonrhabdomyosarcoma soft tissue sarcoma. Pediatr Blood Cancer. 2011;57(2):247–251.
- Xiao SW, Xu Y-Z, Xiao B-F, et al. Recombinant adenovirus-p53 gene therapy for advanced unresectable soft-tissue sarcomas. Hum Gene Ther. 2018;29(6):699–707.
- Schmitt G, Mills EED, Levin V, et al. The role of neutrons in the treatment of soft tissue sarcomas. Cancer. 1989;64(10):2064–2068.