Abstract
Background
In patients with cancer, sarcopenia is associated with treatment related complications, treatment cessation, poor quality of life and reduced overall survival. Despite this, there is limited knowledge about changes in skeletal muscle mass during chemotherapy. The aim of this systematic review and meta-analysis was to investigate the change of skeletal muscle mass and sarcopenia during chemotherapy treatment among patients with lung cancer.
Methods
A systematic literature search was conducted in three databases, PubMed, EMBASE and Web of Science. Observational studies with patients with lung cancer were eligible for inclusion if skeletal muscle mass was measured before and after receiving chemotherapy treatment.
Results
Ten cohort studies with a total of 867 participants met the inclusion criteria. During 5.2 ± 2.9 months of chemotherapy treatment, patients with lung cancer experienced a significant loss of skeletal muscle mass with a standardized mean difference (SMD) of: -0.25 (95% CI -0.47 to -0.03). The pretreatment prevalence of sarcopenia varied across studies from 35% to 74%. Only one study reported prevalence of sarcopenia both before and after chemotherapy treatment with an increase from 35% to 59%.
Conclusion
The present data demonstrate a marked loss of skeletal muscle mass in patients with lung cancer undergoing chemotherapy treatment, as well as a high prevalence of sarcopenia. As sarcopenia is associated with poor clinical outcomes, it seems important to include and use assessments of skeletal muscle mass in clinical practice to identify patients in need for interventions. Moreover, interventional studies to hinder development of sarcopenia are needed.
Introduction
Lung cancer is the most common cancer diagnosis with an estimated annual incidence of 2.1 million, equivalent to 12% of all cancers among both sexes globally. Further, due to its inferior prognosis, lung cancer is the leading cause of cancer deaths worldwide with 1.8 million deaths yearly [Citation1,Citation2].
Lung cancer can be divided into non-small cell lung cancer (NSCLC) and small cell lung cancer (SCLC); where NSCLC is the most frequent and accounts for around 80–85% of cases. According to the international tumor-node-metastasis staging system (TNM), NSCLC can be classified into five stages, 0-IV, with higher level indicating more severe disease [Citation3,Citation4].
Independent predictors for mortality in patients with lung cancer are predominately higher cancer stage and histological type (SCLC). Other predictors include high age (>70 years), being male, and poor performance status at the time of diagnosis [Citation5]. Importantly, survival rates are increasing due to the introduction of targeted therapies [Citation6]. From 2000–2004 to 2010–2014 the average lung cancer-specific 5-year survival improved from 11% to 15% among patients in Europe [Citation7]. The improved survival is leading to an increasing interest in improving the quality of life and physical function among patients during and after treatment [Citation2].
Sarcopenia is a progressive and generalized skeletal muscle disorder that involves accelerated loss of muscle mass and function [Citation8,Citation9]. In the general non-cancer population, the prevalence of sarcopenia has been estimated to 5–13% among people between 60–70 years, and 11–50% among people aged 80 years or older [Citation10]. Longitudinal studies have shown that the yearly loss of muscle mass in the general population is 0.5–1% from around the age of 40 and accelerate slightly to 0.5–2% by the age of 70 [Citation11–13]. The prevalence of sarcopenia is highly variable in patients with cancer, ranging from 5% to 89% among patients with respiratory tract and pancreas cancer; dependent on the stage, type of cancer, and assessment method used [Citation14,Citation15].
In patients with cancer, age-related muscle loss can be accelerated by many factors including an increased inflammatory state and a wide range of treatment-related factors, with chemotherapy treatment being an important element [Citation9,Citation11,Citation16]. The four main causes for the development of sarcopenia during chemotherapy include one or a combination of; (i) reduced food intake with a reduction in vitamin D, omega-3 fatty acids, and protein, (ii) reduced physical activity, (iii) direct effect of chemotherapy on muscles, (iv) malabsorption secondary to mucositis or treatment-related pancreatic insufficiency [Citation17].
Moreover, the presence of multi-morbid conditions and/or inactive lifestyle may contribute to low physical function and low skeletal muscle mass already at the time of diagnosis [Citation18–20].
Notably, low skeletal muscle mass has been demonstrated to be an independent risk factor for postoperative complications and overall survival in surgically treated patients with lung cancer [Citation21]. In addition, sarcopenia has been associated with a wide range of negative outcomes including reduced physical function, disability, frailty, morbidity, inferior quality of life, and depression in patients with cancer [Citation11,Citation22,Citation23]. It therefore seems important to identify patients with accelerated loss of skeletal muscle mass or with sarcopenia before and after chemotherapy treatment. Yet, assessment of muscle mass is still not routinely used in clinical practice.
At present, the majority of studies have retrospectively investigated the prevalence of sarcopenia at the time of diagnosis and the association with postoperative complications, chemotherapy-induced toxicity, disease-free, and overall survival [Citation24,Citation25]. However, the potential loss of skeletal muscle mass during chemotherapy has not yet been systematically evaluated. The aim of this systematic review and meta-analysis was therefore to investigate the change of skeletal muscle mass and sarcopenia in patients with lung cancer undergoing chemotherapy treatment.
Methods
This systematic review and meta-analysis was reported according to Preferred Reporting Items for Systematic reviews and Meta-Analyses (PRISMA) guidelines [Citation26]. PRISMA checklist is presented in Appendix 1.
The protocol was preregistered at the international prospective register of systematic reviews (PROSPERO) ID number: CRD42021241804.
Search strategy
We performed a comprehensive systematic search for relevant articles using three databases: PubMed, Web of Science, and EMBASE published until March 29, 2021. Search terms were cancer survivor AND sarcopenia AND cancer. Reference lists and citations of relevant articles were hand-searched to identify additional studies. The complete search strategy for all databases is presented in Appendix 2.
Eligibility criteria
Studies were considered eligible if they included a lung cancer population treated with chemotherapy. They had to be published in a peer-reviewed journal and include an assessment of skeletal muscle mass before and after chemotherapy treatment using an objective measurement tool; Computed tomography (CT), Magnetic resonance imaging (MRI), Dual-energy X-ray absorptiometry (DXA) or Bioelectrical impedance analysis (BIA). Studies were ineligible if they were not published in English due to the inability to translate or included an intervention to impact muscle mass (e.g. exercise). Full list of selection criteria are presented in Appendix 3.
Selection of studies
Covidence systematic review software; Veritas Health Innovation, Melbourne, Australia, available at www.covidence.org, was used to merge references from the three different electronic databases and to remove duplicate studies. Two reviewers (SJ, ZB) independently assessed the titles and abstracts of all studies. Full texts of the relevant articles were uploaded to Covidence, and the same two reviewers individually evaluated full-text articles based on the eligibility criteria. If disagreement occurred, a third reviewer (BSR) was consulted. If missing information prevented clarity, the study authors were contacted by email throughout the process of study selection, including title-abstract screening and full-text screening. The interrater reliability between the two reviewers was calculated using Cohen’s kappa coefficient. Cohen’s kappa coefficient ranges from −1 to 1, where ≤0 indicates no agreement, 0.01–0.20 none to slight, 0.21–0.40 fair, 0.41–0.60 moderate, 0.61–0.80 substantial and 0.81–1 indicates almost perfect agreement [Citation27].
Data extraction
We used a Microsoft© excel spreadsheet for data extraction designed specifically for this study. The researchers independently extracted data on study characteristics and if disagreement occurred, a third reviewer (BSR) was consulted. Extracted data were as follows: author, publication year, study design, funding, country, patient setting, data source, number of participants, sex proportion, age, body mass index (BMI), Eastern Cooperative Oncology Group Performance Status (ECOG PS), type of lung cancer, stage, type of chemotherapy, number of cycles, muscle mass assessment tool, assessment location, the time between assessments, type of outcome, outcome cutoff value, sarcopenia prevalence, skeletal muscle mass pre-and post-chemotherapy, change of skeletal muscle mass, p-value, and confidence intervals.
Assessment of quality
To access the risk of bias in the included studies, the quality assessment tool for observational cohort and cross-sectional studies published by the National Heart Lung and Blood Institute [Citation28] was applied independently by two reviewers (SJ, ZB). Studies were rated good, fair, or poor quality [Citation28].
Statistical analysis
Change in skeletal muscle mass was pooled across studies and analyzed by a random-effects model using the restricted maximum-likelihood estimator with Hartung-Knapp adjustment to quantitatively synthesize data. Changes in muscle mass from baseline to follow-up assessments are reported as standardized mean differences (SMD) with 95% confidence intervals. If no correlation coefficient was provided, the study authors were contacted by email. In case of no response, 0.5 was chosen as the correlation coefficient between the baseline and follow-up assessments. This did not affect the SMD but had a small impact on the standard error. Statistical heterogeneity was evaluated using the I2 statistics and was considered low, moderate, and high at I2 values of 25%, 50%, and 75% respectively [Citation29]. Visual inspection of funnel plots and the Egger test were used to assess publication bias. SMD was interpreted using ‘Cohen’s effect sizes’; 0.2 represents a small effect, 0.5 a moderate effect, and 0.8 is a large effect [Citation30]. The meta-analyses were performed using R version 4.1.3 and the ‘meta’ package [Citation31]. If the included study did not report both a pretreatment mean skeletal muscle mass index with pretreatment SD, and posttreatment mean skeletal muscle mass index, or if the study reported data as skeletal muscle mass in cm2 or cm2/m2, meta-analyses were considered inappropriate and the results were synthesized narratively. If possible, the within-study percentage changes in skeletal muscle mass from pretreatment to posttreatment were calculated.
Results
Study selection
The comprehensive search yielded a total of 7,903 studies (). After duplicates were removed, 4,790 references remained. 4,235 were removed after the screening of title and abstract, leaving 555 eligible for retrieving full text. 545 were excluded, as they did not meet the inclusion criteria, leaving 10 studies for inclusion in this review. The interrater agreement reliability regarding title-abstract screening was fair with a Cohen’s kappa value of 0.39. Regarding full-text screening, the reliability indicated almost perfect agreement with a Cohen’s kappa value of 0.88.
Study characteristics
Study characteristics are presented in and Appendix 4. All data included were obtained in hospital-based medical records. Eight out of ten studies [Citation32–39] were retrospective cohorts and two [Citation40,Citation41] were prospective. The studies were published between 2015 and 2020. Eight studies [Citation33–40] did not report funding, one study [Citation41] received funding and one study [Citation32] reported not receiving funding. Across the ten studies, the total sample size was n = 867 patients and n = 530 (61%) were males. The average age of participants was 66 years with a range from 35–90 years. The majority of patients were diagnosed with NSCLC, only one study [Citation32] included n = 25 of 200 (12.5%) patients diagnosed with SCLC. Across studies, most patients n = 685 (79%) had stage IV disease. All patients received between 1–12 cycles of chemotherapy and most patients had a performance status of zero or one indicating expected tolerability to chemotherapy.
Table 1. Study characteristics.
Risk of bias in the included studies is shown in . For this research question, it was particularly important to consider selection bias, measurement bias, and adjustment for confounders. All ten studies clearly described the study population, used clearly defined, valid, reliable outcome measurements, and had a sufficient timeframe to see an association between exposure and outcome. However, nine of the studies did not justify a sample size, eight of the studies did not examine different levels of chemotherapy as related to loss of skeletal muscle mass, and none of the studies adjusted for confounding variables. The overall quality was rated as good in two studies and [Citation32,Citation40] and fair in eight [Citation33–38].
Definitions and assessment of muscle mass and sarcopenia
Assessment methods for muscle mass and definitions for sarcopenia are presented in .
Table 2. Sarcopenia prevalence and development.
All studies used CT scans to access muscle mass. Most studies [Citation33–35,Citation37,Citation39–41] used the location of the 3rd lumbar spine L3, while two studies [Citation32,Citation36] used L2–L3 and one study [Citation38] used T10 and L1. Only two studies [Citation33,Citation40] reported the use of blinded assessors for the analysis. Five studies [Citation32,Citation33,Citation37,Citation39,Citation41] reported skeletal muscle index as cm2/m2, four studies [Citation34–36,Citation40] reported the skeletal muscle area as cm2 and one study [Citation38] reported the area in cm3. The cutoff values for assessing sarcopenia varied between studies. Five studies [Citation33,Citation34,Citation37,Citation39,Citation41] used different cutoff criteria for men and women with SMI ranging from 41 to 53 cm2/m2 for men and 31 to 41 cm2/m2 for women. Two studies [Citation35,Citation36] reported a cutoff value for sarcopenia as a loss of ≥10% of the psoas major muscle area. Two studies [Citation36,Citation37] used their own calculations within their data to define a cutoff value for sarcopenia, while five studies used previously sourced cutoff values [Citation33–35,Citation39,Citation41].
Meta-analysis of change of muscle mass
Changes of skeletal muscle mass are presented in and .
Figure 3. Weighted standardized mean difference in skeletal muscle change. TE: Standardized mean difference; TEse: Standard error of standardized mean difference.
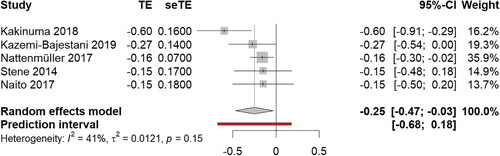
A total of five studies could be summarized using meta-analysis [Citation32,Citation34,Citation39–41] compromising n = 359 patients with lung cancer. Of the included patients, n = 296 (82%) were diagnosed NSCLC and n = 25 (7%) with SCLC. The mean time between the two assessments of skeletal muscle mass was 5.2 ± 2.9 months where patients received palliative- [Citation34] or first-line chemotherapy [Citation32,Citation39–41]. The meta-analysis revealed a small to moderate loss of skeletal muscle mass of SMD: −0.25 (95% CI -0.47 to -0.03) during chemotherapy treatment. Statistical heterogeneity was low to moderate (I2 = 41%) and asymmetry was not detected in the funnel plot or from Egger’s test (p-value = 0.45), indication low possibility of publication bias or systematic differences between studies. Five studies [Citation33,Citation35–38] were not included in the meta-analysis.
Change of muscle mass and prevalence of sarcopenia across all studies
Changes of skeletal muscle mass and the prevalence of sarcopenia are presented in .
Across all ten studies, five studies [Citation32,Citation34,Citation37–39] reported a loss of skeletal muscle mass during chemotherapy of ∼3% to 10% in 3 to 10 months. Two studies [Citation37,Citation41] assessed skeletal muscle mass at several time points. Kimura 2015 et al. reported a loss in skeletal muscle mass of 3.7% from pretreatment to three months after chemotherapy initiation, which remained at six and 12 months after chemotherapy initiation. Similarly, Naito 2017 et al. reported a loss in muscle mass of 4.4% from pretreatment to six weeks after chemotherapy initiation which also remained at 12 weeks after chemotherapy initiation. Four studies reported a pretreatment sarcopenia prevalence of 35% [Citation33], 74% [Citation34], 67% [Citation41], and 46% [Citation39], respectively, and three studies reported posttreatment sarcopenia prevalence of 59% after 4–6 months [Citation33], 55% after 10.2 months [Citation36], and 30% with no timeframe specified [Citation35]. Only one of the above studies reported both pre- and posttreatment sarcopenia which changed from 35% to 59% [Citation33]. Two studies [Citation35,Citation36] reported the prevalence of sarcopenia defined as a loss of ≥10% of the psoas major muscle area in 30% and 55% of the patients posttreatment.
Discussion
To our knowledge, this is the first systematic review to describe the loss of muscle mass in patients with lung cancer undergoing chemotherapy treatment. The results demonstrated a significant loss of skeletal muscle mass of SMD: 0.25 (95% CI 0.47 to 0.03) during chemotherapy treatment. Across studies, the observed loss in skeletal muscle mass was ∼3% to 10% following 3 to 10 months of chemotherapy measured by CT scans. This is markedly higher than the expected age-related loss of muscle mass of 0.5–2% per year observed among the general healthy population measured by DXA [Citation11–13]. However, caution in comparison must be taken due to the different types of measurements used. In the present review, the pretreatment prevalence of sarcopenia varied across studies from 35% to 74%. Only one study reported the prevalence of sarcopenia before and after chemotherapy treatment with an increase from 35% to 59% [Citation33]. The observed accelerated loss of muscle mass during chemotherapy treatment and the prevalence of sarcopenia is consistent with results found in other cancer sites [Citation42–45]. This has a potential clinical and prognostic impact, as maintaining and gaining skeletal muscle mass is significantly related to reduced toxicity to chemotherapy and longer overall patient survival [Citation34].
Of note, the studies by Kazemi-Bajestani 2019 et al. and Kakinuma 2018 et al. stand out with a 7.4% and 9.8% loss in muscle mass following about four months of chemotherapy treatment. Both studies were rated as fair-good quality with a low risk of bias. The large loss of muscle mass could be due to the disease stage, as all the patients in the two cohorts were diagnosed with incurable stage IV NSCLC, whereas most other studies also included patients with earlier stages of lung cancer (). However, the greater loss is not explained by differences in performance status, as most studies included in this review enrolled patients with good performance status (Appendix 4).
Kimura 2015 et al. and Naito 2017 et al. both report a change in SMI at several time points and observed a loss of muscle mass of 3.7% and 4.4% during the first six weeks and three months after chemotherapy initiation, which was maintained and thus not further exacerbated at the 12 weeks and 12 months follow up. As such, these findings indicate that greater loss of skeletal muscle mass occurs within the first months of chemotherapy initiation, and is not regained once the chemotherapy regimen has ended. Studies suggest that the efficacy benefits of chemotherapy are reached after 3–4 cycles of chemotherapy [Citation46,Citation47]. After this point, stable disease or symptom relief of malaise, pain, cough, and dyspnea after chemotherapy may lead to more physical activity and thereby no further exacerbating in loss of muscle mass.
The key question when observing this accelerated loss in muscle mass is what causes this: the disease; the treatment; or the patient (e.g. lifestyle and sociocultural environment). Likely all three, but in what proportion is still unanswered. The loss is most likely multifactorial as patients with lung cancer often have comorbidities, cachexia, and a sedentary lifestyle or experience adverse effects while undergoing chemotherapy treatment [Citation17–20]. Cachexia and sarcopenia are distinct conditions. Cachexia is a disorder characterized by systemic inflammation and loss of body weight (at least 5%) because of extensive wasting of the skeletal muscle and adipose tissue [Citation48,Citation49]. Cachexia is often present already at diagnosis and according to a cohort study with 708 patients with lung cancer, 60% stated they experienced weight loss even before beginning chemotherapy treatment [Citation50]. Thereby, loss in skeletal muscle mass most likely starts before chemotherapy initiation. Moreover, loss of skeletal muscle mass may also be accelerated by a direct effect of chemotherapy, side effects of receiving chemotherapy, or a combination [Citation11]. A previous study has reported that different types of chemotherapy, such as cisplatin, irinotecan, doxorubicin, and etoposide cause direct muscle loss [Citation51]. This is by upregulating proteasome activity, activation on mitogen-activated protein kinase and extracellular regulated kinase signaling, and by inducing mitochondrial dysfunction [Citation51]. Patients with sarcopenia are also more likely to have inferior tolerance to chemotherapy and suffer from chemotherapy toxicity than their non-sarcopenic counterparts [Citation34,Citation52]. In addition, side effects from chemotherapy, such as fatigue, nausea, and vomiting, which in turn may lead to physical inactivity, have also been associated with loss of skeletal muscle mass in patients with cancer [Citation53,Citation54]. Finally, living with a terminal disease like advanced lung cancer can bring with it shame, guilt, feeling like a burden, distress, anxiety, and depression [Citation55–59]. Additionally, it can be difficult to engage in exercise at the end of life, if the motivation is external and in discrepancy with the perception of living a good life [Citation60]. Therefore, maintenance of nutrition and exercise during treatment can be a challenge, and most patients lose significant amounts of weight and skeletal muscle mass during chemotherapy treatment [Citation61].
To date, one of the most used treatments to prevent and manage sarcopenia in patients with cancer is resistance training [Citation11,Citation62,Citation63]. Several studies have demonstrated that resistance training prevents the loss of skeletal muscle mass and increases muscular strength for patients undergoing chemotherapy [Citation64,Citation65]. Exercise interventions during chemotherapy usually consist of supervised progressive resistance training combined with aerobic training twice weekly for 9–12 weeks. Notably, it has been shown, that patients with inoperable lung cancer and a high functional capacity, measured by the functional test, live significantly longer compared to patients with a low functional capacity [Citation66]. However, more knowledge is needed about the effect of exercise, especially in the more severe stages of sarcopenia and in patients with advanced stages of lung cancer.
In addition to resistance training, nutrition supplements, specifically high protein intake, are also used to hinder loss of muscle mass during treatment for lung cancer [Citation67]. However, to date, there is no evidence to suggest that diet alone can counteract the loss of muscle mass [Citation11,Citation68]. In the contrary, oral nutritional supplements have been shown to increase fat mass and body weight with no significant impact on skeletal muscle mass [Citation11,Citation68]. Resistance training, when combined with nutrition interventions can preserve or even increase muscle mass during chemotherapy [Citation63,Citation69]. Therefore, more randomized clinical trials of good quality investigating multimodal interventions that target sarcopenia in cancer patients are highly needed [Citation61,Citation70].
The strengths of this study include our strict compliance with the PRISMA guidelines and a comprehensive search string that increases the probability of capturing all available literature published on the topic. However, while this review aimed to describe changes in skeletal muscle mass during chemotherapy, the cause of these changes remains unanswered.
Additionally, most included studies were retrospective with a high risk of confounding factors. While all the included studies used CT scans to access muscle mass, differences in definitions of sarcopenia between the studies directly affect the estimates and made it difficult to compare prevalence rates between studies in this review. In general, cutoff values for low skeletal muscle mass are not yet well defined and should be investigated to guide both clinical practice and future research [Citation15,Citation71]. Most included studies used first-line chemotherapy, but differences in chemotherapy regimen and line (e.g. first, second, or palliative chemotherapy) and even inclusion of targeted therapies in smaller subgroups may contribute dissimilar to the loss of muscle mass. Finally, as this is an emerging topic in oncology, we included patients that had different disease stages and NSCLC as well as SCLC patients, even though the prognosis of those two types of cancers are largely different and could blur the change in sarcopenia over time. However, it should be noted that despite the heterogeneity of the included studies, all studies reported a significant loss of muscle mass following treatment with chemotherapy.
Implication for practice
As low levels of muscle mass are an independent risk factor for overall survival in patients with lung cancer, it seems important to identify patients with accelerated loss of muscle mass or sarcopenia before, during, and after chemotherapy treatment, to initiate preventive interventions and hinder the progression of the condition. This could be done by introducing a screening of important diagnostic criteria such as muscular strength or physical functioning which could trigger a further assessment of muscle mass using CT or BIA measurement. The present study also underlines the need for more evidence-based and uniform assessments of muscle mass in cancer patients. In general, there is a lack of knowledge surrounding the prevalence and determinants of sarcopenia in patients with lung cancer and the potential effects of targeted exercise and nutritional interventions translating into improved treatment outcomes and increased quality of life. However, there is some evidence that resistance training can attenuate the loss of muscle mass. Therefore, future research should focus on the type and timing of nutrition intake in combination with resistance training, to maintain skeletal muscle mass during cancer treatment. Moreover, studies investigating changes in muscle mass among patients receiving the best supportive care vs. chemotherapy could contribute to clarify to what extent the disease vs. chemotherapy treatment impacts skeletal muscle mass. This is important knowledge for clinicians when addressing the accelerated loss of muscle mass and sarcopenia in patients with cancer.
Conclusion
This systematic review and meta-analysis demonstrate a marked loss in muscle mass and a high prevalence of sarcopenia in patients with lung cancer undergoing chemotherapy. Sarcopenia is associated with several negative prognostic implications for patients with lung cancer. In time, assessment of muscle mass and other diagnostic criteria should be implemented in clinical practice leading to effective interventions to maintain skeletal muscle mass during chemotherapy treatment. However, more research is needed to investigate the complexity of treating muscle loss.
Supplemental Material
Download MS Word (15.9 KB)Supplemental Material
Download MS Word (12.2 KB)Supplemental Material
Download MS Word (15 KB)Supplemental Material
Download MS Word (20.4 KB)Disclosure statement
The authors declare no conflicts of interest.
Data availability statement
The data that support the findings of this study are available from the corresponding author, upon reasonable request to [email protected].
Additional information
Funding
References
- Bray F, Ferlay J, Soerjomataram I, et al. Global cancer statistics 2018: GLOBOCAN estimates of incidence and mortality worldwide for 36 cancers in 185 countries. CA Cancer J Clin. 2018; 68(6):394–424.
- A Jemal LT, Soerjomataram I, Bray F. The cancer atlas. 3 ed. USA: American Cancer Society, Inc; 2019.
- Detterbeck FC, Boffa DJ, Kim AW, et al. The eighth edition lung cancer stage classification. Chest. 2017; 151(1):193–203.
- Larry Jameson ASF J, Kasper DL, et. al. Harrison’s principles of internal medicine. 20 ed.: New York: Cenveo Publisher Services; 2018.
- Blanchon F, Grivaux M, Asselain B, et al. 4-year mortality in patients with non-small-cell lung cancer: development and validation of a prognostic index. Lancet Oncol. 2006;7(10):829–836.
- Howlader N, Forjaz G, Mooradian MJ, et al. The effect of advances in lung-cancer treatment on population mortality. N Engl J Med. 2020; 383(7):640–649.
- OECDiLibrary. Incidence, survival and mortality for lung cancer [cited 2022. 24. february]. Available from: https://www.oecd-ilibrary.org/sites/547f405e-en/index.html?itemId=/content/component/547f405e-en.
- Santilli V, Bernetti A, Mangone M, et al. Clinical definition of sarcopenia. Clin Cases Miner Bone Metab. 2014; 11(3):177–180.
- Cruz-Jentoft AJ, Sayer AA. Sarcopenia. Lancet. 2019; 393(10191):2636–2646.
- von Haehling S, Morley JE, Anker SD. An overview of sarcopenia: facts and numbers on prevalence and clinical impact. J Cachexia Sarcopenia Muscle. 2010; 1(2):129–133.
- Davis MP, Panikkar R. Sarcopenia associated with chemotherapy and targeted agents for cancer therapy. Ann Palliat Med. 2019; 8(1):86–101.
- Wilson D, Jackson T, Sapey E, et al. Frailty and sarcopenia: the potential role of an aged immune system. Ageing Res Rev. 2017; 36:1–10.
- Goodpaster BH, Park SW, Harris TB, et al. The loss of skeletal muscle strength, mass, and quality in older adults: the health, aging and body composition study. J Gerontol A Biol Sci Med Sci. 2006; 61(10):1059–1064.
- Rier HN, Jager A, Sleijfer S, et al. The prevalence and prognostic value of low muscle mass in cancer patients: a review of the literature. Oncologist. 2016; 21(11):1396–1409.
- Simonsen C, Kristensen TS, Sundberg A, et al. Assessment of sarcopenia in patients with upper gastrointestinal tumors: prevalence and agreement between computed tomography and dual-energy x-ray absorptiometry. Clin Nutr. 2021;40(5):2809–2816.
- Atlan P, Bayar MA, Lanoy E, et al. Factors which modulate the rates of skeletal muscle mass loss in non-small cell lung cancer patients: a pilot study. Support Care Cancer. 2017;25(11):3365–3373.
- Kodera Y. More than 6 months of postoperative adjuvant chemotherapy results in loss of skeletal muscle: a challenge to the current standard of care. Gastric Cancer. 2015;18(2):203–204.
- Young RP, Hopkins RJ, Christmas T, et al. COPD prevalence is increased in lung cancer, independent of age, sex and smoking history. Eur Respir J. 2009; 34(2):380–386.
- Titz C, Hummler S, Schmidt ME, et al. Exercise behavior and physical fitness in patients with advanced lung cancer. Support Care Cancer. 2018; 26(8):2725–2736.
- Coups EJ, Park BJ, Feinstein MB, et al. Physical activity among lung cancer survivors: changes across the cancer trajectory and associations with quality of life. Cancer Epidemiol Biomarkers Prev. 2009; 18(2):664–672.
- Kawaguchi Y, Hanaoka J, Ohshio Y, et al. Does sarcopenia affect postoperative short- and long-term outcomes in patients with lung cancer?-a systematic review and meta-analysis. J Thorac Dis. 2021;13(3):1358–1369.
- Nipp RD, Fuchs G, El-Jawahri A, et al. Sarcopenia is associated with quality of life and depression in patients with advanced cancer. Oncologist. 2018; 23(1):97–104.
- Simonsen C, de Heer P, Bjerre ED, et al. Sarcopenia and postoperative complication risk in gastrointestinal surgical oncology: a meta-analysis. Ann Surg. 2018; 268(1):58–69.
- Pamoukdjian F, Bouillet T, Lévy V, et al. Prevalence and predictive value of pre-therapeutic sarcopenia in cancer patients: a systematic review. Clin Nutr. 2018;37(4):1101–1113.
- Yang M, Shen Y, Tan L, et al. Prognostic value of sarcopenia in lung cancer: a systematic review and meta-analysis. Chest. 2019;156(1):101–111.
- Liberati A, Altman DG, Tetzlaff J, et al. The PRISMA statement for reporting systematic reviews and meta-analyses of studies that evaluate healthcare interventions: explanation and elaboration. BMJ. 2009; 339:b2700.
- McHugh ML. Interrater reliability: the kappa statistic. Biochem Med. 2012;22(3):276–282.
- NHLBI. NIH. Study quality assessment tools. [Internet]. Available from: https://www.nhlbi.nih.gov/health-topics/study-quality-assessment-tools.
- Higgins JP, Thompson SG, Deeks JJ, et al. Measuring inconsistency in meta-analyses. BMJ. 2003;327(7414):557–560.
- Nakagawa S, Cuthill IC. Effect size, confidence interval and statistical significance: a practical guide for biologists. Biol Rev Camb Philos Soc. 2007;82(4):591–605.
- PmfR2021. Available from: https://cran.r-project.org/web/packages/meta/meta.pdf.
- Nattenmüller J, Wochner R, Muley T, et al. Prognostic impact of CT-Quantified muscle and fat distribution before and after first-line-chemotherapy in lung cancer patients. PLOS One. 2017;12(1):e0169136.
- Cortellini A, Palumbo P, Porzio G, et al. Single-institution study of correlations between skeletal muscle mass, its density, and clinical outcomes in non-small cell lung cancer patients treated with first-line chemotherapy. Thorac Cancer. 2018;9(12):1623–1630.
- Stene GB, Helbostad JL, Amundsen T, et al. Changes in skeletal muscle mass during palliative chemotherapy in patients with advanced lung cancer. Acta Oncol. 2015; 54(3):340–348.
- Petrova MP, Donev IS, Radanova MA, et al. Sarcopenia and high NLR are associated with the development of hyperprogressive disease after second-line pembrolizumab in patients with non-small-cell lung cancer. Clin Exp Immunol. 2020; 202(3):353–362.
- Nishioka N, Uchino J, Hirai S, et al. Association of sarcopenia with and efficacy of anti-PD-1/PD-L1 therapy in non-small-cell lung cancer. J Clin Med. 2019;8(4):450.
- Kimura M, Naito T, Kenmotsu H, et al. Prognostic impact of cancer cachexia in patients with advanced non-small cell lung cancer. Support Care Cancer. 2015;23(6):1699–1708.
- Goncalves MD, Taylor S, Halpenny DF, et al. Imaging skeletal muscle volume, density, and FDG uptake before and after induction therapy for non-small cell lung cancer. Clin Radiol. 2018;73(5):505.e1–505–e8.
- Kakinuma K, Tsuruoka H, Morikawa K, et al. Differences in skeletal muscle loss caused by cytotoxic chemotherapy and molecular targeted therapy in patients with advanced non-small cell lung cancer. Thorac Cancer. 2018; 9(1):99–104.
- Kazemi-Bajestani SMR, Becher H, Butts C, et al. Rapid atrophy of cardiac left ventricular mass in patients with non-small cell carcinoma of the lung. J Cachexia Sarcopenia Muscle. 2019;10(5):1070–1082.
- Naito T, Okayama T, Aoyama T, et al. Skeletal muscle depletion during chemotherapy has a large impact on physical function in elderly Japanese patients with advanced non-small-cell lung cancer. BMC Cancer. 2017;17(1):571.
- Jang MK, Park C, Hong S, et al. Skeletal muscle mass change during chemotherapy: a systematic review and meta-analysis. Anticancer Res. 2020;40(5):2409–2418.
- Hansen TTD, Omland LH, von Heymann A, et al. Development of sarcopenia in patients with bladder cancer: a systematic review. Semin Oncol Nurs. 2021;37(1):151108.
- Daly LE, Nb ÉB, Power DG, et al. Loss of skeletal muscle during systemic chemotherapy is prognostic of poor survival in patients with foregut cancer. J Cachexia Sarcopenia Muscle. 2018;9(2):315–325.
- van der Zanden V, van Soolingen NJ, Viddeleer AR, et al. Loss of skeletal muscle density during neoadjuvant chemotherapy in older women with advanced stage ovarian cancer is associated with postoperative complications. Eur J Surg Oncol. 2022;48(4):896–902.
- Smith IE, O'Brien ME, Talbot DC, et al. Duration of chemotherapy in advanced non-small-cell lung cancer: a randomized trial of three versus six courses of mitomycin, vinblastine, and cisplatin. J Clin Oncol. 2001;19(5):1336–1343.
- Socinski MA, Schell MJ, Peterman A, et al. Phase III trial comparing a defined duration of therapy versus continuous therapy followed by second-line therapy in advanced-stage IIIB/IV non-small-cell lung cancer. J Clin Oncol. 2002;20(5):1335–1343.
- Evans WJ, Morley JE, Argilés J, et al. Cachexia: a new definition. Clin Nutr. 2008;27(6):793–799.
- Fearon K, Strasser F, Anker SD, et al. Definition and classification of cancer cachexia: an international consensus. Lancet Oncol. 2011;12(5):489–495.
- Ross PJ, Ashley S, Norton A, et al. Do patients with weight loss have a worse outcome when undergoing chemotherapy for lung cancers? Br J Cancer. 2004;90(10):1905–1911.
- Van Gammeren D, Damrauer JS, Jackman RW, et al. The IkappaB kinases IKKalpha and IKKbeta are necessary and sufficient for skeletal muscle atrophy. Faseb J. 2009;23(2):362–370.
- Gérard S, Bréchemier D, Lefort A, et al. Body composition and anti-neoplastic treatment in adult and older subjects – A systematic review. J Nutr Health Aging. 2016;20(8):878–888.
- Stasi R, Abriani L, Beccaglia P, et al. Cancer-related fatigue: evolving concepts in evaluation and treatment. Cancer. 2003;98(9):1786–1801.
- Love RR, Leventhal H, Easterling DV, et al. Side effects and emotional distress during cancer chemotherapy. Cancer. 1989;63(3):604–612.
- Carlson LE, Zelinski EL, Toivonen KI, et al. Prevalence of psychosocial distress in cancer patients across 55 North American cancer centers. J Psychosoc Oncol. 2019;37(1):5–21.
- Zhang L, Liu X, Tong F, et al. Lung cancer distress: screening thermometer meta-analysis. BMJ Support Palliat Care. 2022;0:1–9.
- Geerse OP, Brandenbarg D, Kerstjens HAM, et al. The distress thermometer as a prognostic tool for one-year survival among patients with lung cancer. Lung Cancer. 2019;130:101–107.
- Linares-Moya M, Rodríguez-Torres J, Heredia-Ciuró A, et al. Psychological distress prior to surgery is related to symptom burden and health status in lung cancer survivors. Support Care Cancer. 2022;30(2):1579–1586.
- Zhang JM, Zhang MR, Yang CH, et al. The meaning of life according to patients with advanced lung cancer: a qualitative study. Int J Qual Stud Health Well-being. 2022;17(1):2028348.
- Schoenau MN, Hansen M, Ulvestad S, et al. The lived experiences, perceptions, and considerations of patients after operable lung cancer concerning nonparticipation in a randomized clinical rehabilitation trial. Qual Health Res. 2020;30(5):760–771.
- Dunne RF, Loh KP, Williams GR, et al. Cachexia and sarcopenia in older adults with cancer: a comprehensive review. Cancers. 2019;11(12):1861.
- Cao A, Ferrucci LM, Caan BJ, et al. Effect of exercise on sarcopenia among cancer survivors: a systematic review. Cancers. 2022;14(3):786.
- Edvardsen E, Skjønsberg OH, Holme I, et al. High-intensity training following lung cancer surgery: a randomised controlled trial. Thorax. 2015;70(3):244–250.
- Christensen JF, Jones LW, Tolver A, et al. Safety and efficacy of resistance training in germ cell cancer patients undergoing chemotherapy: a randomized controlled trial. Br J Cancer. 2014; 111(1):8–16.
- Quist M, Langer SW, Lillelund C, et al. Effects of an exercise intervention for patients with advanced inoperable lung cancer undergoing chemotherapy: a randomized clinical trial. Lung Cancer. 2020;145:76–82.
- Kasymjanova G, Correa JA, Kreisman H, et al. Prognostic value of the six-minute walk in advanced non-small cell lung cancer. J Thorac Oncol. 2009;4(5):602–607.
- Williams GR, Dunne RF, Giri S, et al. Sarcopenia in the older adult with cancer. J Clin Oncol. 2021; 39(19):2068–2078.
- Kim SH, Lee SM, Jeung HC, et al. The effect of nutrition intervention with oral nutritional supplements on pancreatic and bile duct cancer patients undergoing chemotherapy. Nutrients. 2019;11(5):1145.
- Jang MK, Park C, Tussing-Humphreys L, et al. The effectiveness of sarcopenia interventions for cancer patients receiving chemotherapy: a systematic review and meta-analysis. Cancer Nurs. 2021;46(2):81–90.
- Solheim TS, Laird BJA, Balstad TR, et al. A randomized phase II feasibility trial of a multimodal intervention for the management of cachexia in lung and pancreatic cancer. J Cachexia Sarcopenia Muscle. 2017;8(5):778–788.
- Cruz-Jentoft AJ, Bahat G, Bauer J, et al. Sarcopenia: revised European consensus on definition and diagnosis. Age Ageing. 2019;48(4):601.
- Martin L, Birdsell L, Macdonald N, et al. Cancer cachexia in the age of obesity: skeletal muscle depletion is a powerful prognostic factor, independent of body mass index. J Clin Oncol. 2013;31(12):1539–1547.
- Prado CM, Lieffers JR, McCargar LJ, et al. Prevalence and clinical implications of sarcopenic obesity in patients with solid tumours of the respiratory and gastrointestinal tracts: a population-based study. Lancet Oncol. 2008;9(7):629–635.