Abstract
Background
Neuroendocrine neoplasms represent a diverse group of malignancies. Anatomic origin, histology and aggressiveness vary extensively, from low-grade tumours with an indolent prognosis to highly aggressive conditions with poor outcome. Surgery, with a curative intent, is the standard of treatment when possible. Other treatment regimens include local treatment, or systemic therapy. The role of radiotherapy in treating neuroendocrine neoplasms is not yet established, but studies indicate that a high rate of local control can be achieved by high-dose radiotherapy. Stereotactic body radiotherapy (SBRT) is high dose of radiation delivered to a small volume. We aimed to investigate the one-year local control rate of SBRT in patients with neuroendocrine neoplasms.
Material and Methods
Patients with neuroendocrine neoplasms treated with SBRT between 2003 and 2021 were retrospectively identified. Patient characteristics and SBRT-details were collected by review of patient records and the radiotherapy planning charts. All types except for small cell lung cancer and brain metastases were allowed. The prescribed dose was 45–67.8 Gy in three fractions. Progression, both within the target-site and in other sites, was determined based on existing imaging reports. One-year local control rate and systemic control rate was calculated. Descriptive analyses of local response duration, progression-free survival and overall survival were performed.
Results
Twenty-one patients were included. The one-year local control rate was 94%. Four of the patients had local progression. All patients receiving SBRT towards their primary tumour (n = 11) had a bronchopulmonary neuroendocrine neoplasm, and a one-year local control rate of 100%. In patients treated at a metastatic target, 80% developed systemic progression but the local control remained high.
Conclusion
Our study suggests that SBRT may offer a feasible and effective treatment of neuroendocrine neoplasms in selected cases. SBRT provides long-term local stability and may be useful in treating patients with localised disease not fit for surgery.
Background
Neuroendocrine neoplasms (NEN) represent a diverse group of malignancies with an increasing incidence worldwide. Anatomic origin, histology and aggressiveness vary extensively, from low-grade tumours with an indolent prognosis to highly aggressive conditions with poor outcome. The most frequent primary anatomical site of NEN is the gastroenteropancreatic system (GEP), preferably the small intestine and pancreas. GEP-NEN account for approximately 60% of all NEN [Citation1]. The second largest group of NEN (20–30%) are located in the bronchopulmonary system (BP-NEN) [Citation2]. In 2019 and 2021 respectively, ‘The World Health Organisation’ (WHO) presented new classification systems for GEP-NEN and BP-NEN [Citation3]. The tumours are classified according to morphology and proliferation (mitotic count and/or Ki67 index) into well-differentiated (WD) neuroendocrine tumours (NET) and poorly differentiated (PD) neuroendocrine carcinomas (NEC). The classification is demonstrated in . Grade 1 and 2 GEP-NET are usually characterised by slow growth patterns and favourable prognosis, while grade 3 neuroendocrine tumours (G3 NET) and especially the high-malignant neuroendocrine carcinomas (G3 NEC) may appear with aggressive behaviour. WD G3 NET often demonstrate a more uncertain course of disease and outcome [Citation4]. BP-NEN are categorised as typical (TC) or atypical carcinoids (AC) and as large cell neuroendocrine carcinomas (LCNEC) and small cell lung cancer (SCLC) () [Citation5,Citation6]. The carcinoids are considered more indolent as opposed to the LCNEC and SCLC. Treatment choice and prognosis are distinctly guided by these classifications [Citation4,Citation7–9].
Table 1. WHO 2019 classification of GEP-NEN and WHO 2021 classification of BP-NEN.
Treatment of NEN has different purposes, from cure to disease control and reduction of symptoms. Different treatment modalities are available, with the final choice depending on the stage of the disease, symptoms and the patient’s performance status. Surgery remains the first choice of treatment of localised disease, but other local treatment modalities may be feasible options in selected cases. Systemic treatments such as somatostatin receptor analogues (SSA), targeted therapy, chemotherapy, and peptide receptor radionuclide therapy (PRRT) are frequently used to manage metastatic disease and reduce symptoms [Citation5,Citation6]. Locoregional treatments include several different modalities, such as radiofrequency ablation (RFA), transarterial chemoembolisation (TACE) and selective internal radiation therapy (SIRT) [Citation10,Citation11]. In situations where surgery is not possible (non-resectable tumours, patients not fit for surgery), or in symptomatic disease with the aim of symptom reduction, these treatments may offer a feasible alternative [Citation6]. Treatment decisions are preferably made by a multidisciplinary team, keeping both available options, the characteristics of the disease and the patient’s condition in mind. Since NEN often have a fairly good prognosis, the treatment must provide high tumour control and take side effects and quality of life into account.
Radiotherapy (RT) is a well-recognised treatment modality in most common cancer types, but its role in NEN management remains to be fully established. In G3 NEC, conventional RT, often concomitant with chemotherapy (CRT), may be used in a neoadjuvant or definitive setting [Citation12–15]. In WD NEN, there are some concerns regarding possible radioresistance, although the mechanisms of such an intrinsic resistency are not well understood. Factors such as tumour developing from rather mature cells, slow proliferation rate, small number of mitoses and intra-tumour heterogeneity has been discussed as possible reasons for a lower sensitivity to radiation [Citation16]. Despite this, radiotherapy may be useful in both palliative and more definite treatment approaches.
Stereotactic body radiotherapy (SBRT) is the common term for a specific technique of radiotherapy, where high doses of radiation per fraction are precisely delivered to a small target volume. This approach increases the effect and chances of long-term local control (LC) SBRT is well established in the treatment of early stages of non-small cell lung cancer (NSCLC), some brain tumours, as well as oligometastatic colorectal cancer [Citation17–19].
The role of SBRT in NEN remains to be determined, as the experience and evidence are sparse. To date, most data on SBRT in NEN consists of case reports [Citation20].
Recently published studies of SBRT in BP-NEN and GEP-NEN allow the assumption that high ablative SBRT doses can overcome the intrinsic radioresistance of NEN. It may thus be a useful strategy to obtain LC and achieve better disease control in both localised and metastatic disease, especially in NEN unsuitable for other local treatment modalities.
With this study, we aimed to evaluate the effect of SBRT on local disease control in patients with NEN.
Material and methods
The study is a retrospective, descriptive cohort study. Patients with NEN, treated with SBRT at the Department of Oncology, Aarhus University hospital (AUH), from 2003 to 2021 were identified. The Department of Oncology, AUH, is part of an ENETS certified centre of excellence, vouching for a multidisciplinary approach to treating NEN. Data regarding patient and disease characteristics, treatment details, outcome and follow-up were retrospectively collected from the patient records. When available, treatment plans from the radiotherapy planning system were obtained, and details of the radiotherapy treatment plans were collected. Data on radiation dose, fraction size and treatment period was available for all patients.
All patients with histologically confirmed NEN treated with SBRT were identified, regardless of grade and primary site of origin. Due to the remarkable aggressiveness and short life expectancy, patients with SCLC were excluded. Previously, concomitant and subsequent systemic treatment was allowed. Additional local treatment, such as RFA, was also allowed, but not on the same target as SBRT. The SBRT was either planned as a definitive or oligometastatic treatment strategy. All anatomic treatment sites (except for brain metastases) were included.
The SBRT was planned and administered according to the department’s standards. Pre-treatment imaging always included a CT scan of the region of interest. Before 2010 a 3D-CT was used. The patients were positioned with both arms above the head in an individualised immobilisation device, further supported by an in-house developed frame for the liver SBRT. To control for target motions, a time-resolved 4D-CT scan was performed and the mid-ventilation (mv) phase was used for delineation of the GTV of thoracic tumours. Liver targets were controlled by abdominal compression and guided by exhale breath-hold CT. Prior to the scan, 2–3 gold-seed fiducial markers were implanted near the liver lesions. Target delineation was performed in cooperation with an experienced radiologist. The GTV was equal to the clinical target volume (CTV). PTV for the lung SBRT was generated by adding a margin of 5 mm transversal and 10 mm longitudinal (before 2015) or by including the respiratory motion into the GTV. For the exhale breath-hold planning the PTV was defined by adding 5 mm and 7 mm margins in the transversal and cranio-caudal directions, respectively. The majority of the patients were treated with 7–8 beams using 3D conformal static beams. Few patients with lung tumours were treated with intensity-modulated radiation therapy (IMRT). Volumetric-modulated arc therapy (VMAT), was preferred for liver SBRT. The photon energy was 6 MV. Prescription dose was 45 or 67,5Gy in 3 fractions, 2 fractions/week. For centrally located lung lesions the prescribed dose was 56 Gy in 8 fractions, 4 fractions/week. Patients were usually planned using inhomogeneous dose distribution where 95%-98% of the GTV-mv was covered by 90% of the prescribed dose and 98% of the PTV was covered by a 67% isodose surface. After 2009, daily cone-beam CT with soft tissue match on the GTV was performed. The maximum diameter of the lesions was 5 cm. All constraints of organs at risk were kept.
Treatment response and duration were evaluated by review of existing imaging evaluation performed routinely (CT of thorax and abdomen performed every third/fourth month) as part of the general control program. The in-site response was characterised as stable disease (SD) or progression. Systemic progression was determined as the appearance of new lesions, or significant growth of existing lesions requiring a reassessment of the current treatment strategy. One-year LC rate was determined as the percentage of patients with in-site SD one year after treatment start. One-year systemic control was determined as the percentage of patients with no evidence of systemic progression after one year. Furthermore, the three-year LC rate was described in patients where follow-up beyond three years was available. LC rates were described for the entire cohort, and for metastastic or primary site respectively. Descriptive analyses of local response duration, progression free survival (PFS) and overall survival (OS) were performed. PFS was defined as time from treatment beginning (first fraction delivered) until either local or systemic progression. OS was defined as time from treatment beginning until death of any cause. Survival data was assessed by the Kaplan Meier method and demonstrated as survival plots. All data was collected by permission of the Danish Patient Safety Authority (3-3013-3276/1). Statistical analyses were performed in IBM SPPS Statistics v.20.
Results
Twenty-six patients were identified according to the criteria previously listed. Two patients were treated for brain metastases, one had no patient record available, one never finished the SBRT due to co-morbidity, and one had a synchronous metastatic rectum cancer and died shortly after the SBRT. These five patients were excluded from further analyses. Thus, 21 patients were included in the final study. Only one lesion per patient was treated. Patient characteristics are presented in . Thirteen women and eight men were included, with a mean age of 69 years (range 39–86). Anatomic origin and grade are described in . Most patients (67%) had a primary lung tumour, 24% originated from the gastro-intestinal system, and 9% were classified as unknown primary tumours. Both WD and PD tumours were represented, although a significant part (81%) of the pathology reports did not describe this in detail. The grade was, therefore, primarily determined by the KI-67 proliferation index, according to the WHO NEN classification system. The Ki67 proliferation index was between 1 and 80% (). Based on this, 57% of the patients had grade 1–2 disease, 33% had grade 3 NET or NEC, and 10% were not available for further categorisation.
Table 2. Pt. characteristics.
47% of the patients received additional treatment before SBRT (). These treatments included up-front surgery, SSA, chemotherapy, and in two cases SSA or chemotherapy followed by PRRT. In 53% SBRT was the primary treatment.
53% of the patients were treated at the primary tumour site, while 47% of the treated lesions were metastatic (). All of the metastases but one (lung) were located in the liver. All patients receiving primary tumour site treatment had a primary BP-NEN. The GTV volume ranged from 1,5 to 25,7 cm3 and 15,3 to 74,8 cm3 of the lung and liver respectively. The prescribed median biological equivalent dose (BED10) of radiation was 189,11 Gy (range 95,2–219,68 Gy). The dose prescription varied as described in .
Table 3. SBRT characteristics (BED10, prescribed dose and treatment site).
As illustrated in , four patients had local failure during the follow-up period. All of them also developed systemic progression. Nine patients died before experiencing local failure, four of them within the first year. The one-year local control rate was thus 94%. The one-year systemic control rate was 63%. At the end of the study, eight patients had not yet experienced local progression, and ten were still alive.
Patients treated with definitive intent at the primary tumour site (n = 11) had a one-year local control rate of 100%. The median follow-up time was 21 months (range 4–73). At the end of follow-up, none of these patients had local progression. One had systemic progression, and eight were still alive. Among the patients treated at a metastatic target, 80% had systemic progression during the follow-up, but the local control remained high.
For patients with a follow-up of three years and beyond (n = 13), the three-year LC rate was determined. Six patients had been treated at their primary tumour and seven at a metastatic target. Four patients had local progression during the three-year follow-up. All of them had been treated at a metastatic target, and thus the LC-rate for patients treated at their primary tumour remained 100%.
The median PFS and the median OS for the entire group were 12 (1-73) and 21 (4-218) months, respectively. The three-year survival curves of LC, PFS and OS are demonstrated as Kaplan-Meier curves in .
Figure 1. The swimmerplot illustrates follow-up time and events (local failure, systemic failure and death) of each patient respectively.
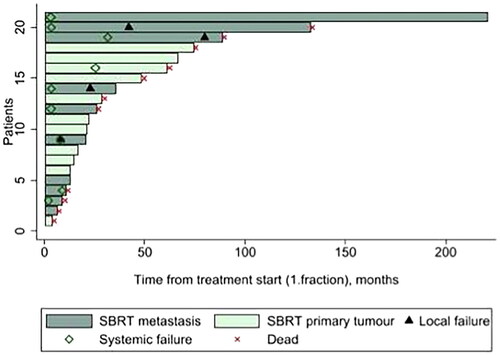
Figure 2. Survival curves. The Kaplan-Meier curves demonstrates the three-year local control (A), PFS (B) and OS (C) for all of the patients included and available at follow-up after three years or more.
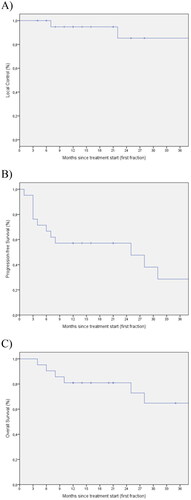
Patients who received SBRT towards the primary tumour, had a median OS of 21 months (4–73). Since only one patient had distant progression and none had local progression, PFS was not described.
Discussion
The clinical management of NEN has improved over recent years but remains challenging. Our study demonstrated a high local control rate in both primary and metastatic NEN treated with SBRT. Overall, the one-year LC rate was 94%, and the systemic control rate was 63%. We included different types of NEN and anatomical sites and observed a high LC across the subtypes. The evidence of radiotherapy in NEN is sparse, but our data correlate with a review from 2018 where Chan D L et al. described the effect of external beam radiotherapy in patients with GEP-NEN of any histological grade. The material was heterogeneous in respect of tumour characteristics and type of radiotherapy, but they demonstrated relatively high LC-rates and symptomatic relief [Citation21].
In our study, we exclusively included patients treated with SBRT, which allows for higher doses of radiotherapy compared to a more conventional approach of RT, thus implying even higher possibilities of achieving LC. This is further supported by a study performed by Chen et al. They retrospectively analysed data of 45 patients with NEN of different grade end origin treated with high doses of radiotherapy. The patient composition was similar to ours, but the doses of radiotherapy were lower, with a median BED of 72. Both primary and metastatic lesions were treated. The 2-year local response rate was 98%, but 45% developed systemic progression with new metastases later on [Citation15].
Patients treated with SBRT at their primary tumour site, had a one-year LC-rate of 100%. All of these patients had a BP-NEN. Only one patient experienced systemic progression during follow-up, and all remained under local control. These findings support that SBRT is a feasible and effective treatment of localised BP-NEN. In a recent study, Lo H and colleagues investigated the OS in patients with early-stage BP-NEC receiving either SBRT or surgery. The median OS was 57,2 months after surgery compared to 34,6 months in the SBRT group. Hence, surgery is superior, but SBRT might be a viable alternative to surgery in non-resectable patients [Citation22]. Wegner R E et al. demonstrated similar mOS and superiority in favour of SBRT, comparing SBRT with conventional chemo-radiotherapy in patients with early-stage BP-NEC (mOS 34.7 months vs 23.7 months respectively) [Citation14]. In our study, low-grade BP-NEN was also included, indicating a beneficial role of SBRT in both high-and low-grade diseases. Since SBRT is well established in managing lung cell adenocarcinomas, the practical experiences and techniques will often be easily applicable to treating BP-NEN [Citation23]. Thus, our findings strongly indicate that SBRT may be a valid alternative to surgery in patients who are not fit for resection because of co-morbidity, tumour location or unwilling to undergo invasive procedures.
Among the patients treated at a metastatic site (primarily liver metastases), the LC-rate was also high, but a significant part of the patients experienced systemic progression during their course of disease. This is consistent with previous findings by Hudson J M et al. [Citation24]. They described the role of SBRT in managing liver metastases from WD GEP-NEN. In a retrospective study, 25 patients were treated with SBRT. A total of 53 metastases were treated, and a high one-year LC-rate of 92% was demonstrated. Local progression was only seen in patients with grade 2 disease. The treatment was well tolerated with little toxicity. In our study, any grade of NEN, including grade 3-NEN and NEC were included. Especially patients with grade 3-NEN and NEC were prone to develop systemic progression, but it was also seen in patients with grade 1 NEN. Our results thus indicate that SBRT in metastatic NEN might be useful to achieve local control, but systemic treatment is still necessary to minimise systemic progression. SBRT of liver metastases is a feasible option in the armamentarium of treating localised disease. We suggest that it may be useful when patients are unfit for invasive procedures, when tumour size exceeds the boundaries for RFA (usually 3 cm), or the tumour location is unsuitable for percutaneous RFA due to complication risks.
There are several limitations to our study. The number of patients was small, and the population was heterogeneous. This is an inherent problem in the study of NEN, as the disease is rare, but nonetheless, it affects the possibility of achieving a good fundament for conclusions. Furthermore, the time span of inclusion was long, which may affect the treatment standard and the clinical practice concerning patients offered the specific treatment. Radiotherapy, including SBRT, has experienced significant developments over the passing years, and thus there may be differences in treatment planning and execution between patients included at the beginning of the period and the end. In counterbalance to this, the fact that all patients received their treatment at the same centre may to some extent compensate for this. Regarding the image-evaluation, The RECIST-criteria were not consistently used, making further characterisation of response and response rates impossible. Finally, the retrospective design impairs the consistent collection of data.
In conclusion, our study supports the use of SBRT, especially in the treatment of non-metastatic BP-NEN. SBRT may be a viable alternative to surgery in non-resectable patients. In metastatic NEN, SBRT might be useful to achieve local control, but systemic treatment is still necessary to minimise systemic progression in G3 NET and NEC. Further, prospective studies are highly warranted.
Supplemental Material
Download MS Word (23 KB)Disclosure statement
No potential conflict of interest was reported by the author(s).
Data availability statement
The data that support the findings of this study is available upon reasonable request from the corresponding author (ADN). The data are not publicly available as they contain information that could compromise the privacy of research participations.
References
- Singh S, Asa SA, Kennecke H, et al. Diagnosis and management of gastrointestinal neuroendocrine tumors: an evidence-based Canadian consensus. Cancer Treat Rev. 2016;47:32–45.
- Dasari A, Shen C, Halperin D, et al. Trends in the incidence, prevalence, and survival outcomes in patients with neuroendocrine tumors in the United States. JAMA Oncol. 2017;3(10):1335–1342.
- The 2019 WHO classification of tumours of the digestive system. Histopathology. 2020;76:182–188.
- Sorbye H, Welin S, Langer SW, et al. Predictive and prognostic factors for treatment and survival in 305 patients with advanced gastrointestinal neuroendocrine carcinoma (WHO G3): the NORDIC NEC study. Ann Oncol. 2013;24(1):152–160.
- Tabaksblat E, Langer SW, Knigge U, et al. Diagnosis and treatment of bronchopulmonary neuroendocrine tumours: state of the art. Acta Oncol. 2016;55(1):3–14.
- Kasajima A, Klöppel G. Neuroendocrine tumor G3 of bronchopulmonary origin and its classification. Pathol Int. 2022;72(10):488–495.
- Janson ET, Knigge U, Dam G, et al. Nordic guidelines 2021 for diagnosis and treatment of gastroenteropancreatic neuroendocrine neoplasms. Acta Oncol. 2021;60(7):931–941.
- Pavel M, Öberg K, Falconi M, et al. Gastroenteropancreatic neuroendocrine neoplasms: ESMO clinical practice guidelines for diagnosis, treatment and follow-up. Ann Oncol. 2020;31(7):844–860.
- Hofland J, Kaltsas G, de Herder WW. Advances in the diagnosis and management of Well-Differentiated neuroendocrine neoplasms. Endocr Rev. 2020;41(2):371–403.
- Strosberg J, El-Haddad G, Wolin E, et al. Phase 3 trial of 177Lu-Dotatate for midgut neuroendocrine tumors. N Engl J Med. 2017;376(2):125–135.
- Camus B, Cottereau AS, Palmieri LJ, et al. Indications of peptide receptor radionuclide therapy (PRRT) in gastroenteropancreatic and pulmonary neuroendocrine tumors: an updated review. J. Clin. Med. 2021;10:1–15.
- Honma Y, Nagashima K, Hirano H, et al. Clinical outcomes of locally advanced esophagal neuroendocrine carcinoma treated with chemoradiotherapy. Cancer Med. 2020;9(2):595–604.
- Iwata T, Ueno H, Itami J, et al. Efficacy of radiotherapy for primary tumor in patients with unresectable pancreatic neuroendocrine tumors. Jpn J Clin Oncol. 2017;47(9):826–831.
- Wegner ER, Abel S, Colonias A. Stereotactic ablative body radiotherapy versus conventionally fractioned radiotherapy for early stage large cell neuroendocrine carcinoma of the lung. Lung Cancer Manag. 2020;9(3):LMT32.
- Chen KS, Lawhn-Heath C, Behr S, et al. Outcomes after high-dose radiation in the management of neuroendocrine neoplasms. PLoS ONE. 2021;16(6):e0252574.
- Waldum HL, Öberg K, Sørdal ØF, et al. Not only stem cells, but also mature cells, particularly neuroendocrine cells, may develop into tumours: time for a paradigm shift. Therap Adv Gastroenterol. 2018;11:175628481877505.
- Mahadevan A, Blanck O, Lanciano R, et al. Stereotactic body radiotherapy (SBRT) for liver metastasis – clinical outcomes from the international multi-institutional RSSearch® patient registry. Radiat Oncol. 2018;13(1):26.
- Soldà F, Lodge M, Ashley S, et al. Stereotactic radiotherapy (SABR) for the treatment of primary non-small cell lung cancer; systematic review and comparison with a surgical cohort. Radiother Oncol. 2013;109(1):1–7.
- Guckenberger M, Andratschke N, Dieckmann K, et al. ESTRO ACROP consensus guideline on implementation and practice of stereotactic body radiotherapy for peripherally located early stage non-small cell lung cancer. Radiother Oncol. 2017;124(1):11–17.
- Myrehaug S, Hallet J, Chu W, et al. Proof of concept for stereotactic body radiation therapy in the treatment of functional neuroendocrine neoplasms. J. Radiosurg SBRT. 2020;6:321–324.
- Chan DL, Thompson R, Lam M, et al. External beam radiotherapy in the treatment of gastroenteropancreatic neuroendocrine tumours: a systematic review. Clin Oncol. 2018;30(7):400–408.
- Lo H, Abel S, Finley G, et al. Surgical resection versus sterotactic body radiation therapy in early stage bronchopulmonary large cell neuroendocrine carcinoma. Thorac Cancer. 2020;11(2):305–310.
- Khalil A, Knap MM, Møller DS, et al. Local control after stereotactic body radiotherapy of centrally located lung tumours. Acta Oncol. 2021;60(8):1069–1073.
- Hudson JM, Chung HTK, Chu W, et al. Stereotactic ablative radiotherapy for the management of liver metastases from neuroendocrine neoplasms: a preliminary study. Neuroendocrinology. 2022;112(2):153–160.