Abstract
Background
Limited data exist comparing intensity-modulated photon (IMRT) and proton (IMPT) radiation therapy when treating the prostate bed and pelvic lymph nodes in the postoperative setting for prostate cancer. The aim of this study was to evaluate dosimetric differences between IMRT and IMPT when treating with whole pelvis radiation therapy (WPRT) postoperatively.
Materials and methods
IMRT and IMPT plans were generated for 10 post-prostatectomy patients treated between July and August 2020. The prescription was 50 Gy radiobiologic equivalent (GyE) (proton radiobiological effective dose 1.1) to the pelvis and 70 GyE to the prostate bed in 2 GyE per fraction. Paired 2-sided Wilcoxon signed-rank tests were used to compare clinical target volume (CTV) coverage and dose to organs at risk (OARs).
Results
CTV coverage was met for all plans with 99% of CTVs receiving ≥99% of prescription doses. Dose to OARs was significantly higher with IMRT than IMPT for the following endpoints: bladder V5-V65; bowel V5-V45; sigmoid V5-V50; rectum V5-V70; femoral head V40 and maximum dose; bone V5-V65. Select endpoints with significant differences included bladder V30 (63.5 vs. 44.4%, p < .001), bowel V15 (949 vs. 191 cc, p = .001) and V30 (386 vs. 121 cc, p < .001), rectum V40 (81.8 vs. 32.1%, p < .001) and V50 (47.6 vs. 24.9%, p < .001), femoral head maximum doses (46.4–47.1 vs. 38.3–38.6GyE, p < .001), and bone V10 (93.3 vs. 85.4%, p < . 001). Mean doses for all OARs were significantly higher with IMRT, including bladder (41.9 vs. 29.7GyE, p < .001), bowel (21.2 vs. 5.5GyE, p < .001), and rectum (50.8 vs. 27.3GyE, p < .001). Integral dose to ‘Body – CTV’ was significantly higher with IMRT (32.8 vs. 18.4 J, p < .001).
Conclusion
IMPT provides comparable target coverage to IMRT when treating prostate cancer with WPRT in the postoperative setting while significantly reducing dose to OARs. These data can inform the future clinical management and delivery of post-prostatectomy irradiation for prostate cancer.
Keywords:
Background
Prostate cancer (PCa) is the most common malignancy diagnosed in males in the United States [Citation1,Citation2]. The majority of patients with adverse pathologic features who undergo radical prostatectomy experience biochemical failure [Citation3]. The proportion of men with high-risk PCa undergoing prostatectomy rose from 22.8% in 2004 to 40.5% in 2016, suggesting that the number of patients requiring postoperative radiation therapy (RT) will correspondingly increase [Citation4]. The additive acute and late treatment morbidities of radiation in this setting are generally of concern, given this patient population’s increased underlying urinary dysfunction post-prostatectomy.
Postoperative whole pelvis RT (WPRT) decreases risk of progression but comes at the expense of increased acute and late toxicity [Citation5,Citation6]. Thus, investigation of methods to improve the therapeutic ratio and reduce integral dose exposure, which may carry significant lifetime risks, becomes paramount.
Due to its unique depth-dose distribution with rapid fall off beyond the Bragg peak [Citation7], proton therapy may provide benefit in the sparing of surrounding organs at risk (OARs) in comparison with intensity-modulated photon RT (IMRT), particularly when treating the large volumes included in WPRT [Citation8,Citation9].
While proton therapy’s dosimetric benefits have been demonstrated in the setting of intact prostate irradiation [Citation10–13] and in the postoperative setting when treating the prostate bed only [Citation14], there have been no studies to our knowledge to date evaluating differences between proton therapy and IMRT in the postoperative setting with the inclusion of WPRT. Given the differences between WPRT when treating definitively versus postoperatively in terms of radiation dose and volumes, this is an avenue worthy of exploration. The aim of this study was to evaluate dosimetric differences between IMRT and modern scanning beam intensity-modulated proton therapy (IMPT) in the postoperative treatment of PCa when treating with WPRT.
Materials and methods
Following institutional review board approval, our institutional database was queried to identify 10 consecutive patients with adenocarcinoma of the prostate who were treated with postoperative IMPT from July to August 2020. Patients underwent CT simulation in the supine position with a full bladder and empty rectum using immobilization and rectal balloon.
Clinical (CTVs) and planning target volumes (PTVs) were identical for IMRT and IMPT plans. CTVs included the regional pelvic lymph nodes (CTV_50) and the surgical bed (CTV_70). All volumes were delineated in accordance with the RTOG 0534 protocol [Citation5] and using the 2021 NRG Oncology Consensus Pelvic Lymph Node Atlas [Citation15].
PTVs were created from CTV expansions based on our institutional protocol. PTV_50 included a 5 mm isometric expansion from CTV_50. PTV_70 included a 5 mm expansion from CTV_70 in lateral, anterior, and posterior directions, and a 6 mm expansion in inferior and superior directions. PTV_70_Eval included a 5 mm expansion from PTV_70 laterally. This structure was used to facilitate the evaluation of robust optimization. The goal was to cover PTV_70_Eval with 95% of the prescription isodose to evaluate for robustness.
‘Rectum’ was limited to 10 mm cranial and caudal to the CTV. ‘Bone’ included all bones 10 mm cranial and caudal to the CTV and was created as a surrogate for dose to bone marrow. ‘Body – CTV’ included dose to the entire patient excluding any overlapping CTV.
The prescription was 50 Gy radiobiologic equivalent (GyE) to CTV_50 and 70GyE to CTV_70 in 2GyE per fraction, with proton doses calculated using radiobiological effective doses of 1.1. IMRT and IMPT plans were created for each patient with predefined CTV and OAR objectives as outlined in , with target coverage prioritized over OAR constraints. IMRT plans were generated using volumetric-modulated arc therapy, consisting of two 360-degree coplanar arcs. IMPT plans originally created for the treatment of all 10 patients were used as a baseline but reevaluated by appropriate personnel for the purposes of this study. IMPT plans were created using 4 fields, including opposed laterals inferiorly matched to 2 posterior oblique (PO) beams superiorly, optimized with a single-field optimization (SFO) technique. An example of the dose-color-wash distribution of each plan can be visualized in and Supplemental Figure 1.
Figure 1. Axial dose-color-wash comparing IMRT and IMPT plans. Representative dose-color-wash images in axial planes comparing intensity-modulated photon radiation therapy (IMRT) (left) and intensity-modulated proton therapy IMPT (right) plans, with the dose increasing as the color ranges from blue to green to red. Axial images are divided into superior (top), middle (middle), and inferior (bottom) portions of the target. As shown, low to intermediate doses to surrounding organs at risk were higher with IMRT plans.
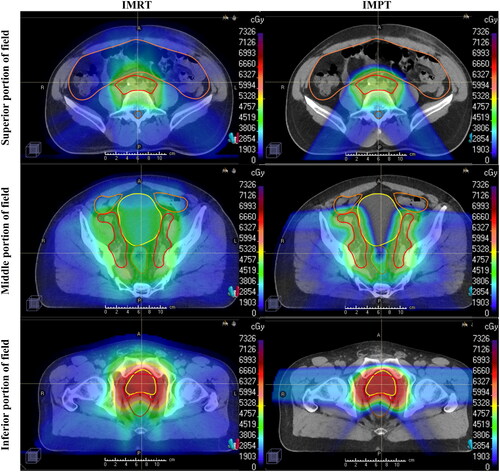
Table 1. Comparative dosimetric outcomes for IMRT and IMPT plans.
All plans were generated using the RayStation treatment planning system (RaySearch Laboratories, Stockholm, Sweden). Proton plans consisted of IMPT using pencil beam scanning with discrete spot scanning. Inverse optimization was used to generate appropriate dose distribution with pre-specified weighting of target coverage and OAR sparing using modulation of beam spot location, energy, and weight. All proton plans utilized 3.5% range uncertainty and 3 mm setup uncertainty from CTV for robust optimization.
Paired 2-sided Wilcoxon signed-rank tests were used to compare plans. Given the large number of endpoints and the multiple comparisons problem, Bonferroni corrections were applied to minimize the likelihood of false-positive results. All statistical analyses were conducted using Matlab (MathWorks, Natick, MA) and Excel (Microsoft, Redmond, WA).
Results
provides comparative median values for target coverage and OAR doses. OAR DVHs are shown in and Supplemental Figure 2(A,B).
Figure 2. (A–D) Comparison of IMRT and IMPT for each organ at risk. Doses to organs at risk shown over the entire dose-volume histogram comparing intensity-modulated photon radiation therapy (IMRT) (blue) and intensity-modulated proton therapy (IMPT) (red) plans, including bladder (2A), bowel cavity (2B), rectum (2C), and ‘Body – CTV’ (2D).
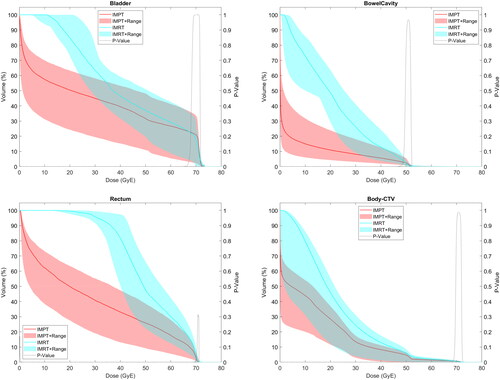
All CTV planning goals were met with IMRT and IMPT plans. CTV_50 D100% was significantly lower with IMRT than IMPT (48.5 vs. 49.5%, p = .001).
Nearly all dosimetric OAR endpoints were significantly higher with IMRT than IMPT plans, including: bladder V5-V65; bowel V5-V45; sigmoid V5-V50, maximum dose; rectum V5-V70; femoral head V37, V40, maximum doses; bone V5-V65; body maximum dose. While all values can be found in , , and Supplemental Figure 2(A,B), select endpoints with significant differences included bladder V30 (63.5 vs. 44.4%, p < .001), bowel V15 (949 vs. 191 cc, p = .001) and V30 (386 vs. 121 cc, p < .001), rectum V40 (81.8 vs. 32.1%, p < .001) and V50 (47.6 vs. 24.9%, p < .001), femoral head maximum doses (46.4–47.1 vs. 38.3–38.6GyE, p < .001), and bone V10 (93.3 vs. 85.4%, p < .001) and V40 (31.8 vs. 21.8%, p < .001).
Mean doses for all analyzed OARs were significantly higher with IMRT than IMPT, including bladder (41.9 vs. 29.7GyE, p < .001), bowel (21.2 vs. 5.5GyE, p < .001), rectum (50.8 vs. 27.3GyE, p < .001), and bone (32.3 vs. 27.0GyE, p < .001). Integral dose to ‘Body – CTV’ was significantly higher with IMRT than IMPT (32.8 vs. 18.4 J, p < .001).
The following OAR constraints were met only for IMPT, with median values amongst the 10 IMRT plans failing to meet these constraints: bladder V30 < 50%, V45 < 40%, V50 < 35%, V65 < 20%; bowel V15 < 830cc, V30 < 300cc; rectum V40 < 40%, V50 < 30%, V60 < 20%; bone V10 < 90%. The only constraint not met by not the median values of IMPT plans was bladder V70 < 20%.
Discussion
The results from this study show that IMPT provides comparable target coverage to IMRT in the postoperative treatment of PCa when treating with WPRT while significantly reducing OAR dose.
There are no prospective studies to date proving that the dosimetric benefit seen with proton therapy will lead to a reduction in toxicity and improved quality of life in the treatment of PCa. However, the volumes of irradiated bowel and rectum have been shown to be highly predictive for GI toxicity [Citation16–19]. Our study shows a significant reduction in the bowel V5-45 and rectum V5-70 with IMPT. Several of these endpoints have been shown to predict GI toxicity, including bowel V15 and V40 and rectum V10, V20, V60, and V70, all of which were significantly lower with IMPT plans in our analysis [Citation18–20].
Our study shows a significant reduction in bladder V5-65 with IMPT. Bryant et al. found a statistically significant correlation between G3 GU toxicity and bladder V30 on multivariate analysis (HR:0.5, p = .0208) [Citation21]. Bladder V30 was significantly higher with IMRT than IMPT in our study.
Several femoral head dosimetric endpoints were significantly lower with IMPT in our study, including V40 and maximum point dose. A dose-response study published by Rasmusson et al. suggests a correlation between femoral head V40 and osteoarthritis risk, highlighting this may be an important metric to consider [Citation22].
Bone V5-65 was significantly lower with IMPT in our study. This is particularly noteworthy given the increased risk of lymphopenia with the addition of WPRT [Citation23]. Certain bone marrow dosimetric endpoints have been shown to correlate with hematologic toxicity, including V10 < 90%, which was only achieved with IMPT plans in our study, while IMRT plans exceeded this constraint [Citation24,Citation25]. While its effect on outcomes for PCa remains uncertain, lymphopenia has been shown to correlate with overall survival for cancer patients in several studies, suggesting that this is a metric worth highlighting when discussing the potential benefit of IMPT [Citation26,Citation27].
A limitation of this study was that all IMRT plans were generated retrospectively for comparative purposes. However, all plans were reviewed by appropriate personnel and were felt to be worthy of approval. While all plans were created >2 years before publication, there have been no changes to our institutional approaches to planning or treatment strategy since that time. CTV coverage was similar between IMRT and IMPT, however PTV coverage was not compared, given that its relevance is conceptually different between the two modalities. All patients were also treated using rectal balloons, which may influence dose deposition. Thus, dosimetric rectal outcomes must be interpreted with caution when treating without rectal balloon.
Another limitation was the lack of correlative toxicity and patient reported/quality of life outcomes to pair with our dosimetric analysis. A future direction worthy of exploration involves assessing associations between DVH parameters and patient reported outcomes using big data, artificial intelligence, deep machine learning, and other advanced investigational methods. We are currently in the process of conducting additional normal tissue complication probability analyses to assess whether the dosimetric outcomes shown here have the potential to lead to differences in predicted rates of toxicity.
Results from ongoing prospective trials such as COMPPARE (NCT03561220), PARTIQoL (NCT01617161), PAROS (NCT04083937), and PRO-proton (NCT05350475) will likely provide more definitive answers on any clinical benefits such as reduced toxicity and improved quality of life with the use of proton irradiation [Citation28].
Conclusion
This study is the first to provide a dosimetric comparison of intensity-modulated photon and proton radiation therapy in the postoperative setting when treating the prostate bed and pelvic lymph nodes. Nearly all dosimetric OAR endpoints were lower and better spared with proton plans than with IMRT. These data can inform the future clinical management and delivery of post-prostatectomy irradiation while awaiting prospective comparative investigations.
Supplemental Material
Download MS Word (13.6 KB)Supplemental Material
Download MS Word (242.8 KB)Supplemental Material
Download MS Word (891.3 KB)Disclosure statement
No potential conflict of interest was reported by the author(s).
Data availability statement
The data that support the findings of this study are available from the corresponding author, EG, upon reasonable request.
Additional information
Funding
References
- Rawla P. Epidemiology of prostate cancer. World J Oncol. 2019;10(2):63–89. doi: 10.14740/wjon1191.
- American Cancer Society. Cancer Facts and Figures. 2022. https://www.cancer.org/content/dam/cancer-org/research/cancer-facts-and-statistics/annual-cancer-facts-and-figures/2021/cancer-facts-and-figures-2021.pdf.
- Tendulkar RD, Agrawal S, Gao T, et al. Contemporary update of a multi-institutional predictive nomogram for salvage radiotherapy after radical prostatectomy. J Clin Oncol. 2016;34(30):3648–3654. doi: 10.1200/JCO.2016.67.9647.
- Agrawal V, Ma X, Hu JC, et al. Trends in diagnosis and disparities in initial management of high-risk prostate cancer in the US. JAMA Netw Open. 2020;3(8):e2014674. 08 03 doi: 10.1001/jamanetworkopen.2020.14674.
- Pollack A, Karrison TG, Balogh AG, et al. The addition of androgen deprivation therapy and pelvic lymph node treatment to prostate bed salvage radiotherapy (NRG oncology/RTOG 0534 SPPORT): an international, multicentre, randomised phase 3 trial. Lancet. 2022;399(10338):1886–1901. doi: 10.1016/S0140-6736(21)01790-6.
- Dearnaley D, Griffin CL, Lewis R, et al. Toxicity and patient-reported outcomes of a phase 2 randomized trial of prostate and pelvic lymph node versus prostate only radiotherapy in advanced localised prostate cancer (PIVOTAL). Int J Radiat Oncol Biol Phys. 2019;103(3):605–617. doi: 10.1016/j.ijrobp.2018.10.003.
- Wilson RR. Radiological use of fast protons. Radiology. 1946;47(5):487–491. doi: 10.1148/47.5.487.
- Trofimov A, Nguyen PL, Coen JJ, et al. Radiotherapy treatment of early-stage prostate cancer with IMRT and protons: a treatment planning comparison. Int J Radiat Oncol Biol Phys. 2007;69(2):444–453. Oct 01 doi: 10.1016/j.ijrobp.2007.03.018.
- Vargas C, Fryer A, Mahajan C, et al. Dose-volume comparison of proton therapy and intensity-modulated radiotherapy for prostate cancer. Int J Radiat Oncol Biol Phys. 2008;70(3):744–751. doi: 10.1016/j.ijrobp.2007.07.2335.
- Whitaker TJ, Routman DM, Schultz H, et al. IMPT versus VMAT for pelvic nodal irradiation in prostate cancer: a dosimetric comparison. Int J Part Ther. 2019;5(3):11–23. doi: 10.14338/IJPT-18-00048.1.
- Vees H, Dipasquale G, Nouet P, et al. Pelvic lymph node irradiation including pararectal sentinel nodes for prostate cancer patients: treatment optimization comparing intensity modulated X-rays, volumetric modulated arc therapy, and intensity modulated proton therapy. Technol Cancer Res Treat. 2015;14(2):181–189. doi: 10.7785/tcrt.2012.500405.
- Widesott L, Pierelli A, Fiorino C, et al. Helical tomotherapy vs. intensity-modulated proton therapy for whole pelvis irradiation in high-risk prostate cancer patients: dosimetric, normal tissue complication probability, and generalized equivalent uniform dose analysis. Int J Radiat Oncol Biol Phys. 2011;80(5):1589–1600. doi: 10.1016/j.ijrobp.2010.10.005.
- Chera BS, Vargas C, Morris CG, et al. Dosimetric study of pelvic proton radiotherapy for high-risk prostate cancer. Int J Radiat Oncol Biol Phys. 2009;75(4):994–1002. doi: 10.1016/j.ijrobp.2009.01.044.
- Santos PMG, Barsky AR, Hwang WT, et al. Comparative toxicity outcomes of proton-beam therapy versus intensity-modulated radiotherapy for prostate cancer in the postoperative setting. Cancer. 2019;125(23):4278–4293. doi: 10.1002/cncr.32457.
- Hall WA, Paulson E, Davis BJ, et al. NRG oncology updated international consensus atlas on pelvic lymph node volumes for intact and postoperative prostate cancer. Int J Radiat Oncol Biol Phys. 2021;109(1):174–185. doi: 10.1016/j.ijrobp.2020.08.034.
- Kuban DA, Tucker SL, Dong L, et al. Long-term results of the M. D. Anderson randomized dose-escalation trial for prostate cancer. Int J Radiat Oncol Biol Phys. 2008;70(1):67–74. doi: 10.1016/j.ijrobp.2007.06.054.
- Tucker SL, Cheung R, Dong L, et al. Dose-volume response analyses of late rectal bleeding after radiotherapy for prostate cancer. Int J Radiat Oncol Biol Phys. 2004;59(2):353–365. doi: 10.1016/j.ijrobp.2003.12.033.
- Huang EH, Pollack A, Levy L, et al. Late rectal toxicity: dose-volume effects of conformal radiotherapy for prostate cancer. Int J Radiat Oncol Biol Phys. 2002;54(5):1314–1321. doi: 10.1016/s0360-3016(02)03742-2.
- Deville C, Jain A, Hwang WT, et al. Initial report of the genitourinary and gastrointestinal toxicity of post-prostatectomy proton therapy for prostate cancer patients undergoing adjuvant or salvage radiotherapy. Acta Oncol. 2018;57(11):1506–1514. doi: 10.1080/0284186X.2018.1487583.
- Kavanagh BD, Pan CC, Dawson LA, et al. Radiation dose-volume effects in the stomach and small bowel. Int J Radiat Oncol Biol Phys. 2010;76(3 Suppl):S101–S107. doi: 10.1016/j.ijrobp.2009.05.071.
- Bryant C, Smith TL, Henderson RH, et al. Five-year biochemical results, toxicity, and patient-reported quality of life after delivery of dose-escalated image guided proton therapy for prostate cancer. Int J Radiat Oncol Biol Phys. 2016;95(1):422–434. doi: 10.1016/j.ijrobp.2016.02.038.
- Rasmusson E, Nilsson P, Kjellén E, et al. Long-term risk of hip complications after radiation therapy for prostate cancer: a dose-response study. Adv Radiat Oncol. 2021;6(1):100571. doi: 10.1016/j.adro.2020.09.011.
- Schad MD, Dutta SW, Muller DM, et al. Radiation-related lymphopenia after pelvic nodal irradiation for prostate cancer. Adv Radiat Oncol. 2019;4(2):323–330. doi: 10.1016/j.adro.2019.01.005.
- Mell LK, Kochanski JD, Roeske JC, et al. Dosimetric predictors of acute hematologic toxicity in cervical cancer patients treated with concurrent cisplatin and intensity-modulated pelvic radiotherapy. Int J Radiat Oncol Biol Phys. 2006;66(5):1356–1365. doi: 10.1016/j.ijrobp.2006.03.018.
- Mell LK, Sirák I, Wei L, et al. Bone marrow-sparing intensity modulated radiation therapy with concurrent cisplatin for stage IB-IVA cervical cancer: an international multicenter phase II clinical trial (INTERTECC-2). Int J Radiat Oncol Biol Phys. 2017;97(3):536–545. doi: 10.1016/j.ijrobp.2016.11.027.
- Venkatesulu BP, Mallick S, Lin SH, et al. A systematic review of the influence of radiation-induced lymphopenia on survival outcomes in solid tumors. Crit Rev Oncol Hematol. 2018;123:42–51. doi: 10.1016/j.critrevonc.2018.01.003.
- Grossman SA, Ellsworth S, Campian J, et al. Survival in patients with severe lymphopenia following treatment with radiation and chemotherapy for newly diagnosed solid tumors. J Natl Compr Canc Netw. 2015;13(10):1225–1231. doi: 10.6004/jnccn.2015.0151.
- Koerber SA, Katayama S, Sander A, et al. Prostate bed irradiation with alternative radio-oncological approaches (PAROS) – a prospective, multicenter and randomized phase III trial. Radiat Oncol. 2019;14(1):122. doi: 10.1186/s13014-019-1325-x.