Abstract
Background: Delivered radiotherapy doses do not exactly match those planned for a course of treatment, largely due to inter-fraction changes in anatomy. In this study, accumulated delivered dose was calculated for a sample of cervical cancer patients, by deformably registering daily cone beam computed tomography (CBCT) images to the planning computed tomography (CT) scan. Planned and accumulated doses were compared for the clinical target volume (CTV), bladder, and rectum.
Material and Methods: For 10 patients receiving 45 Gy in 25 fractions of external beam radiotherapy, daily dose distributions were calculated on CBCT. These images were deformed onto the planning CT and the dose was accumulated using Velocity 4.1 (Varian Medical Systems, Palo Alto, USA). The quality of deformable image registration was evaluated visually and by calculating Dice similarity coefficients and mean distance to agreement.
Results: V95%>99% was achieved for the primary CTV in 9/10 patients for the planned dose distribution and 7/10 patients for the accumulated dose distribution. Primary CTV coverage by 95% of the prescription dose was reduced in one patient, due to an increase in anterior-posterior separation. Comparison of planned and accumulated dose volume histograms (DVHs) for the bladder and rectum found agreement within 5% at low and intermediate doses, but differences exceeded 20% at higher doses. Direct addition of CBCT DVHs was seen to be a poor estimate for the accumulated DVH at higher doses.
Conclusion: Computation of delivered radiotherapy dose that accounts for inter-fraction anatomical changes is important for establishing dose-effect relationships. Updating delivered dose distributions after each fraction would support informed clinical decision making on any potential treatment interventions.
Background
The standard of care for locally advanced cervical cancer is external beam radiotherapy (EBRT) with concurrent weekly cisplatin, followed by brachytherapy (BT) [Citation1]. Use of intensity modulated radiotherapy (IMRT) or volumetric modulated arc therapy (VMAT) with daily image guidance is recommended [Citation2] in order to reduce the treated volume [Citation3] and the rate of toxicity [Citation4–8]. Image-guided adaptive BT with multiple magnetic resonance imaging (MRI) scans are currently the gold standard [Citation9]. Adaption of EBRT, while less common, has been implemented by several centers [Citation10]. Online daily replanning can be performed using a magnetic resonance linear accelerator or a computed tomography (CT) guided system such as the Ethos (Varian Medical Systems, Palo Alto, USA), but these are very resource intensive. Alternative solutions include a plan of the day (POTD) selection from a plan library [Citation11,Citation12] or frequent rescanning and replanning [Citation13,Citation14]. Plan adaption is particularly advantageous in the case of cervical cancer, due to significant inter-fraction tumour motion [Citation15]. As treatments become more adaptive and complex, approaches for accurately calculating the overall delivered dose are needed. This will enable smaller margins, lower doses to organs at risk (OARs), a better understanding of dose-response relationships and can be used to inform decisions around pelvic reirradiation in the event of local or regional recurrence [Citation16].
Many studies have investigated dose accumulation for gynaecological radiotherapy [Citation17,Citation18]. Deformable image registration (DIR) is required to model the complex movement of anatomy in the pelvis and changes in bladder and rectal filling. Quantification of the errors associated with DIR is challenging [Citation19], since synthetic phantoms do not represent the complexities of anatomical deformations [Citation20] and the ground truth deformation vector field is not known when using patient data. Errors are typically evaluated using similarity metrics to compare deformed and manually generated contours. Virtual phantoms created by applying known anatomically relevant deformations to real patient images have been used to validate commercially available DIR algorithms for head and neck, thorax and male pelvis [Citation21–23].
The majority of dose accumulation studies for cervical cancer either focus entirely on BT or accumulate the dose from BT with the planned EBRT dose [Citation17]. The latter is especially challenging since the images for BT contain applicators and packing material unlike those for EBRT. Several authors [Citation24–28] have compared OAR dose-volume histogram (DVH) parameters from accumulated BT dose and planned EBRT dose to those obtained by direct parameter addition, which calculates the worst-case scenario of overlapping high dose regions [Citation29]. Van Heerden et al. [Citation30] calculated cumulative D2cc from EBRT and BT to the bladder and rectum based on daily imaging and found <4% difference to the assumption of a uniform EBRT dose. Patients in our centre are treated using an adaptive POTD approach with plans selected from a library. The goal of this study is to determine the delivered dose from a course of EBRT, by deformably accumulating doses calculated on daily cone beam computed tomography (CBCT) scans and to compare this to the planned dose for the clinical target volume (CTV), bladder and rectum.
Materials and methods
Patient characteristics
Ten consecutive patients treated for carcinoma of the cervix between 2017 and 2019 were selected from a total population of 82 using the following exclusion criteria: stage 1 or 4 disease; radical hysterectomy; previous pelvic or abdominal radiotherapy; radiotherapy to para-aortic lymph nodes; 60 Gy nodal boost(s); less than 4 courses of weekly cisplatin; less than 18 months follow up data available. Clinical and treatment-related parameters are given in . All patients received 45 Gy in 25 fractions of EBRT, with 4 or 5 courses of concurrent weekly cisplatin (40 mg/m2), followed by high dose rate (HDR) BT delivering 28 Gy in 4 fractions. Written consent for their data to be used for research purposes was obtained in all cases.
Table 1. Patient characteristics.
Pre-treatment imaging, radiotherapy planning, and treatment
External beam radiotherapy
CT scans were taken on either an Aquilion LB (Toshiba Medical Systems Corp, Ōtawara, Japan) or a Somatom Definition AS (Siemens Healthineers, Erlangen, Germany). MRI scans were taken on a Magnetom C! or a Magnetom Aera (Siemens Healthineers, Erlangen, Germany). Prior to scanning, patients were asked to empty their bladders and bowels. Bowel preparation with micro enemas was used when necessary. An empty bladder CT scan was acquired, after which the patient was asked to empty their bladder again and drink 300 ml of water. A T2 weighted MRI was then acquired straight after completion of drinking, followed by a second full bladder CT scan 1 h from the start of drinking. The empty bladder CT and MRI were registered using bony anatomy to the full bladder CT, which was used for planning (see in Supplementary Material). The uterus is expected to be more ante-verted (AV) in the case of an empty bladder and more retro-verted (RV) for a full bladder. Low-risk CTVs for the primary tumour were drawn according to the EMBRACE II protocol using the empty bladder CT (ctv_TLR_45_AV), full bladder CT (ctv_TLR_45_RV) and MRI (ctv_TLR_45_MR). An elective nodal CTV, ctv_E_45, was drawn on the full bladder scan and a nodal planning target volume (PTV) was created using a 5 mm margin (ptv_E_45). Little internal motion is expected at the level of the nodes. Uterine motion between the empty and full bladder scans was assessed and each patient was designated as a ‘mover’ or ‘non-mover’ for the adaptive POTD approach. In all cases a standard plan was made, combining ctv_TLR_45_RV and ctv_TLR_45_MR and expanding by 15 mm to generate a standard primary tumour PTV, ptv_T_45_SD. This includes an additional 5 mm on top of our standard pelvis primary CTV to PTV margin of 10 mm, to account for inter-fraction uterine motion not captured by the pre-treatment imaging. For non-movers, a second plan was created, with all 3 CTVs combined and expanded by 10 mm to obtain the primary tumour PTV, ptv_T_45_1. For movers, there were 2 additional plans: ptv_T_45_AV was the 10 mm expansion of ctv_T_45_MR and ctv_T_45_AV, and ptv_T_45_RV was the 10 mm expansion of ctv_T_45_MR and ctv_T_45_RV. All treatment plans were created in the Eclipse (Varian Medical Systems, Palo Alto, USA) treatment planning system (TPS) version 11.0.47 or 15.6.6 using VMAT technique with 2 or 3 full arcs and 6 MV photon energy, see Table in Supplementary Material for planning goals. The final dose calculation was performed using the Anisotropic Analytical Algorithm. Patients were treated on Clinac 2100CD and TrueBeam linear accelerators (Varian Medical Systems, Palo Alto, USA). Image-guided radiotherapy including POTD selection was performed using daily CBCT.
Brachytherapy
Plastic or titanium intra-uterine and ring applicators with interstitial needles (Varian Medical Systems, Palo Alto, USA) were inserted using ultrasound guidance under general anaesthetic in a single theatre session. MRI and CT scans were subsequently performed, which were registered according to applicator positioning. A high-risk CTV was drawn using the MRI. The bladder, rectum and bowel were drawn on the CT image set, which was used for visualising the applicators and planning. Plans were created in Brachyvision (Varian Medical Systems, Palo Alto, USA), maximising CTV coverage by the prescription isodose within the limits of OAR dose constraints, calculated for the whole EBRT and BT treatment. An image-guided adaptive approach was used. Fraction 1 was delivered on the same day as the theatre session. The same plan was delivered for fraction 2 the following morning, after which the patient had a CT scan, enabling the delivered dose distribution for fraction 2 to be reconstructed and a new plan to be created for fraction 3. Fraction 3 was delivered on the same day as fraction 2 with a minimum gap of 6 h. Fraction 4 was delivered the following day after the patient had another CT scan and (in the majority of cases) a new plan. All treatments were delivered using a GammaMed HDR afterloader (Varian Medical Systems, Palo Alto, USA).
Dose accumulation using deformable image registration
Dose distributions were calculated in the Eclipse TPS (Varian Medical Systems, Palo Alto, USA) on each CBCT except for 5 out of 250 fractions that were delivered on a Clinac. Since we do not have a sufficiently accurate CT number to electron density calibration curve for Clinac CBCTs, these 5 fractions were calculated on an image from an alternative fraction delivered on a TrueBeam with similar patient geometry. The most similar image was chosen by a visual comparison of uterus orientation, as well as bladder and rectum filling and positioning. CBCT dose calculation was performed using a copy of the calibration curve from our Toshiba Aquilion CT scanner. This is used clinically for pelvis CBCT dose calculations, as it was found to be more accurate than either a calibration curve generated from an electron density phantom scanned on CBCT, or a patient-specific curve. Gamma analysis comparing dose distributions calculated on CT to CBCT using the CT scanner curve found an average pass rate of 95.1% (1%, 1 mm) and 99.9% (2%, 2 mm) for a sample of 10 patients. Although the bladder, rectum, and primary CTV were completely within the imaged length, additional tissue was required to replicate scatter conditions for accurate dose calculation in these regions. Hence each CBCT was extended 3-5 cm superiorly and inferiorly by repeatedly copying the end slices. This is also done for clinical dose assessment if the scan does not extend sufficiently beyond the region of interest. The couch was created manually and overridden as in treatment planning, since the CBCT field of view does not contain the full lateral extent of the couch. For 2 patients the field diameter of 46.5 cm was not large enough to image their full lateral extent, so the missing parts were estimated by copying the body contour from the planning CT to the CBCT, subtracting the CBCT body contour from the planning CT body contour to create the missing tissue and overriding this to unit density, see Supplementary Fig. 2. The bladder and rectum were contoured on each CBCT manually by a medical physicist. An alternative approach to dose calculation on extended CBCT, is to use a synthetic CT created by deforming the planning CT to match the geometry of the CBCT and utilising the Hounsfield Unit values from the planning CT.
Dose accumulation was performed in Velocity 4.1 (Varian Medical Systems, Palo Alto, USA) using the Accumulation: ACTIVE Dosimetry navigator. Velocity utilises a modified B-spline deformable algorithm with mutual information-based matching [Citation31]. Each CBCT was rigidly and then deformably registered to the planning CT scan. The software applies the same deformation vector field to the associated dose file and then sums the deformed doses from all fractions on the planning CT. Deformable image registration is particularly challenging in the pelvis where bladder and rectal filling can vary significantly, so quality assurance checks are required [Citation19]. Some initial attempts at creating DIRs resulted in non-anatomical warping of bone or muscle or deformed CBCT images that looked very different to the planning CT being matched to, for example, different bladder volume or orientation of uterus, see Supplementary Fig. 3.
Deformable image registration was performed manually using the deformable multi-pass (10.2% of cases), extended deformable multi-pass (36.3% of cases) or structure guided deformable (53.5% of cases) algorithms. The deformable multi-pass algorithm was typically tried first, followed by the extended deformable multi-pass algorithm, which has a finer resolution, and lastly the structure-guided deformable algorithm. The structure-guided deformable algorithm was generally required when there were large changes in bladder and/or rectal volume or positioning. The user outlines one or more structures in both images and the algorithm applies more weight to the voxels within these structures. For similar images, the deformable multi-pass or extended deformable multi-pass algorithms were sufficient. All DIRs were verified visually by a physicist to check for non-anatomical deformations and ensure that the bladder, rectum, and tissue corresponding to the low-risk CTV were moving and warping as expected. In addition to blending the deformed CBCT and planning CT, a map of deformation vectors indicating the direction and magnitude of voxel movement was examined together with a map of the Jacobian determinant to highlight regions of expansion and contraction. Dice similarity coefficients (DSCs) and mean distance to agreement (MDA) were calculated between the propagated bladder and rectum contours and those on the planning CT for both the rigid registrations (RRs) and DIRs. demonstrates the DIR for a case where the bladder was much fuller during treatment compared to the planning CT. shows the deformed CBCT where the superior part of the bladder has been pushed inferiorly to match the planning CT, as indicated by the arrows. The superior part of the rectum has also been moved posteriorly, as expected. The deformed OAR contours are displayed and are an acceptable match for those outlined on the planning CT.
Results
The mean DSC for the bladder was 0.68 for RR and 0.78 for DIR (p = 0.0001, two-tailed paired T-test). For the rectum the mean DSC was 0.57 for RR and 0.66 for DIR (p < 0.0001, two-tailed paired T-test). These values are higher than other DSCs reported for CBCT to CT DIR for gynaecological patients [Citation32]. The mean MDA for bladder was 6.6 mm and 4.2 mm for RR and DIR (p < 0.0002, two-tailed paired T-test). This is consistent with values published for CT to CT DIR in Velocity [Citation33]. For the rectum, the mean MDA was 5.1 mm and 3.8 mm for RR and DIR (p < 0.0001, two-tailed paired T-test).
shows V95% for the low-risk primary tumour CTV as contoured on the full bladder scan (ctv_TLR_45_RV) for the planned and accumulated dose distributions. The planned dose distribution refers to the sum of the dose distributions of the available plans, weighted according to the number of fractions the plan was delivered. V95%>99% is satisfied in 9/10 patients for the planned dose distributions and 7/10 patients for the accumulated dose distributions. For patient 3, V95%=37.3% for the accumulated dose to ctv_TLR_45_RV and D98%=93.1%. Examination of the daily CBCTs showed that the superior part of ctv_TLR_45_RV was not covered by 95% of the prescribed dose, due to different bowel positioning resulting in the patient having a consistently >2 cm larger anterior-posterior separation in this region when treated compared to her planning CT. No evidence of recurrence has been seen in the patient’s follow-up scans since completion of treatment over 4 years ago.
shows the difference in bladder and rectum DVHs between the planned and accumulated dose distributions for each patient. For both OARs the accumulated dose may be above or below the planned dose for an individual patient, although for the bladder the overall tendency is for the planned dose to be higher. Much of this is attributed to differences in bladder filling; the average bladder size during treatment was 23% higher than that during the planning scan. Ordering the patients according to the increasing height of the peak in and the percentage difference in bladder size on treatment relative to planning gives a Spearman’s correlation coefficient of 0.6 (p = 0.067). The dashed lines indicate the mean of the absolute differences. This remains below 5% until 40.6 Gy for the bladder and 40.0 Gy for the rectum, suggesting there is little difference between planned and accumulated DVHs at lower and intermediate doses. Larger differences are seen at higher doses with the mean in the absolute value of the difference peaking at 28.9% for the bladder and 24.7% for the rectum. shows that D2cc was higher for planned than accumulated dose distributions for the bladder in 9/10 patients and the rectum in 7/10 patients. Median values of the differences in D2cc are shown in . Lower average D2cc for accumulated dose distributions is due to differences in organ positioning between fractions, resulting in inter-fractional changes in the position of hotspots.
Figure 3. Difference in DVH between planned and accumulated dose distributions for each patient for (a) bladder and (b) rectum. Dashed line indicates mean of magnitude of differences.
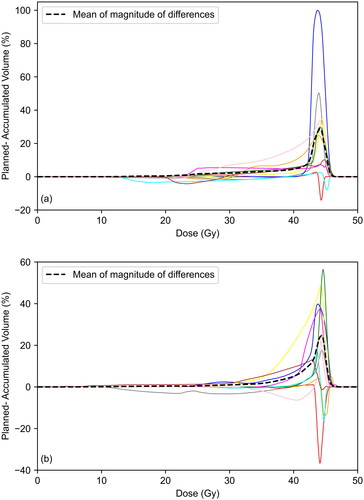
Figure 4. Variations in planned, accumulated and summed CBCT D2cc (Gy) for (a) bladder and (b) rectum.
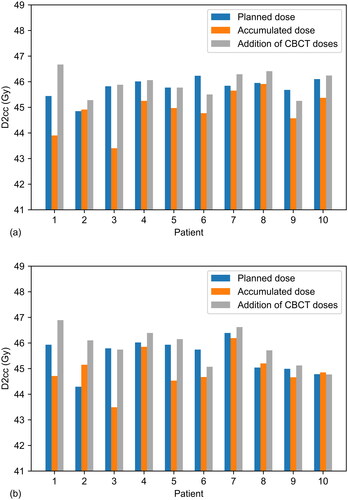
Table 2. Median values of D2cc for the rectum and bladder with range in parentheses.
Exploratory analyses were performed to look for correlations between the difference in planned and accumulated mean doses to the OARs and age, menopausal status, body mass index, CTV volume, and histology. Unfortunately, the sample size is too small to achieve adequate statistical power. However, it was observed that the 4 largest differences in mean rectal dose were observed for the 4 movers. In addition, out of the 5 patients who were pre-menopausal when starting treatment, 4 of these had the largest differences in mean rectal dose.
. shows the difference between the summation of DVHs from each CBCT dose calculation and the DVH from the accumulated dose distribution. The dashed line indicates the mean value of the absolute differences. There is reasonable agreement at low and intermediate doses with the mean value of the absolute difference below 5% for doses below 41.8 Gy for the bladder and 38.6 Gy for the rectum. At higher doses, the summation of CBCT DVHs is a poor estimate for the accumulated DVH. As seen in . and . summing D2cc from all CBCT dose calculations overestimates accumulated D2cc for the bladder in 10/10 patients and the rectum in 9/10 patients, since the former approach does not allow for overall hotspot reduction with interfraction organ movement.
Discussions
This study does not include the effects of intra-fraction motion, since in most cases only one CBCT was acquired per fraction. It is also limited by the small sample size, which is due to resource limitations, notably the amount of time required to complete the dose accumulation. For each patient the following steps had to be performed manually 25 times: CBCT extension and plan creation; bladder and rectum contouring on CBCT; CBCT to CT RR and DIR. Ideally, this process would be fully automated and performed on every patient after each fraction with an alert triggered if certain constraints aren’t met. However, depending on the image quality, auto-contouring algorithms for bladder and rectum may not yet be sufficiently accurate on CBCT and it is essential that each DIR is checked by an experienced member of staff. Developing a more efficient approach could also enable more accurate normal tissue complication probability models to be developed that are based on delivered rather than planned doses.
To the best of our knowledge, this is the first study to estimate the delivered EBRT dose to the target using daily imaging and DIR. In ref. [Citation30] D2cc to the bladder and rectum for EBRT and BT combined was reported. The mean differences in D2cc calculated using deformable dose accumulation based on daily imaging and direct addition assuming uniform EBRT dose, with the range given in parentheses, were 1.1 GyEQD2 (−0.2–3.7 GyEQD2) for the bladder and 0.0 GyEQD2 (−1.0–2.8 GyEQD2) for the rectum. We found mean differences in D2cc of −0.2 GyEQD2 (−2.0–1.2 GyEQD2) for the bladder and −0.1 GyEQD2 (−1.9–1.5 GyEQD2) for the rectum. These findings are broadly consistent in magnitude, although for the bladder we found slightly lower D2cc values relative to the prescription dose.
Deformable image registration is a useful tool for estimating delivered EBRT dose for cervical cancer patients, accounting for inter fraction differences in uterus position and bladder and rectal filling. In some cases, substantial differences were observed between planned and accumulated dose distributions, for example the clinical target volume was covered by the 93% isodose instead of the 95% isodose for a patient who was consistently larger during treatment, due to different bowel positioning, compared to planning. For OARs, the largest differences were seen at higher dose levels, with planned doses typically exceeding accumulated doses. This was particularly pronounced for the bladder, where D2cc was higher for planned than accumulated dose distributions in 9/10 patients. CBCT DVH addition was found to be a good estimate for the deformably accumulated DVH for the OARs at low and intermediate doses, but not at higher doses.
Ethics approval and consent to participate
All patients gave written consent for their data to be used for research purposes.
Supplemental Material
Download JPEG Image (31.5 KB)Supplemental Material
Download JPEG Image (34.2 KB)Supplemental Material
Download JPEG Image (47.8 KB)Supplemental Material
Download JPEG Image (33.8 KB)Supplemental Material
Download JPEG Image (32.8 KB)Supplemental Material
Download JPEG Image (32.5 KB)Supplemental Material
Download JPEG Image (51.7 KB)Supplemental Material
Download MS Word (25.8 KB)Acknowledgements
Consultant oncologists and staff of physics and radiotherapy who cared for these patients. Anitta Thomas who performed some of the dose calculations on CBCT.
Disclosure statement
No potential conflict of interest was reported by the author(s).
Data availability statement
The datasets used and/or analysed during the current study are available from the corresponding author on reasonable request.
Additional information
Funding
References
- Colombo N, Carinelli S, Colombo A, et al. Cervical cancer: ESMO clinical practice guidelines for diagnosis, treatment and follow-up. Ann Oncol. 2012;23 (Suppl 7):vii27–32. doi: 10.1093/annonc/mds268.
- Pötter R, Tanderup K, Kirisits C, et al. The EMBRACE II study: the outcome and prospect of two decades of evolution within the GEC-ESTRO GYN working group and the EMBRACE studies. Clin Transl Radiat Oncol. 2018;9:48–60. doi: 10.1016/j.ctro.2018.01.001.
- Berger T, Assenholt M, Seppenwoolde Y, et al. Importance of technique, dose prescription, and contouring in cervix external beam radiation therapy: current and future practice in a large multicenter study (EMBRACE). Int J Radiat Oncol Biol Phys. 2016;96(2):E292. doi: 10.1016/j.ijrobp.2016.06.1359.
- Klopp AH, Yeung AR, Deshmukh S, et al. A phase III randomized trial comparing Patient-Reported toxicity and quality of life (QOL) during pelvic intensity modulated radiation therapy as compared to conventional radiation therapy. Int J Radiat Oncol Biol Phys. 2016;96(2):S3. doi: 10.1016/j.ijrobp.2016.06.024.
- Chopra S, Engineer R, Mahantshetty UM, et al. Phase III RCT of postoperative adjuvant conventional radiation (3DCRT) versus IGIMRT for reducing late bowel toxicity in cervical cancer (PARCER) (NCT01279135/CTRI2012/120349): results of interim analyses. Int J Radiat Oncol Biol Phys. 2015;93(3):S4. doi: 10.1016/j.ijrobp.2015.07.016.
- Gandhi AK, Sharma DN, Rath GK, et al. Early clinical outcomes and toxicity of intensity modulated versus conventional pelvic radiation therapy for locally advanced cervix carcinoma: a prospective randomized study. Int J Radiat Oncol Biol Phys. 2013;87(3):542–548. doi: 10.1016/j.ijrobp.2013.06.2059.
- Naik A, Gurjar OP, Gupta KL, et al. Comparison of dosimetric parameters and acute toxicity of intensity-modulated and three-dimensional radiotherapy in patients with cervix carcinoma: a randomized prospective study. Cancer Radiother. 2016;20(5):370–376. doi: 10.1016/j.canrad.2016.05.011.
- Mundt AJ, Mell LK, Roeske JC. Preliminary analysis of chronic gastrointestinal toxicity in gynecology patients treated with intensity-modulated whole pelvic radiation therapy. Int J Radiat Oncol Biol Phys. 2003; 56(5):1354–1360. doi: 10.1016/s0360-3016(03)00325-0.
- Dimopoulos JCA, Petrow P, Tanderup K, et al. Recommendations from gynaecological (GYN) GEC-ESTRO working group (IV): basic principles and parameters for MR imaging within the frame of image based adaptive cervix cancer brachytherapy. Radiother Oncol. 2012;103(1):113–122. doi: 10.1016/j.radonc.2011.12.024.
- Shelley CE, Barraclough LH, Nelder CL, et al. Adaptive radiotherapy in the management of cervical cancer: review of strategies and clinical implementation. Clin Oncol (R Coll Radiol). 2021;33(9):579–590. doi: 10.1016/j.clon.2021.06.007.
- Heijkoop ST, Langerak TR, Quint S, et al. Clinical implementation of an online adaptive plan-of-the-day protocol for nonrigid motion management in locally advanced cervical cancer IMRT. Int J Radiat Oncol Biol Phys. 2014;90(3):673–679. doi: 10.1016/j.ijrobp.2014.06.046.
- Buschmann M, Majercakova K, Sturdza A, et al. Image guided adaptive external beam radiation therapy for cervix cancer: Evaluation of a clinically implemented plan-of-the-day technique. Z Med Phys. 2018;28(3):184–195. doi: 10.1016/j.zemedi.2017.09.004.
- Stewart J, Lim K, Kelly V, et al. Automated weekly replanning for intensity-modulated radiotherapy of cervix cancer. Int J Radiat Oncol Biol Phys. 2010;78(2):350–358. Octdoi: 10.1016/j.ijrobp.2009.07.1699.
- Lim K, Stewart J, Kelly V, et al. Dosimetrically triggered adaptive intensity modulated radiation therapy for cervical cancer. Int J Radiat Oncol Biol Phys. 2014;90(1):147–154. doi: 10.1016/j.ijrobp.2014.05.039.
- Li X, Wang L, Cui Z, et al. Online MR evaluation of inter- and intra-fraction uterus motions and bladder volume changes during cervical cancer external beam radiotherapy. Radiat Oncol. 2021;16(1):179. doi: 10.1186/s13014-021-01907-1.
- Mulani J, Jain J, Gupta A, et al. Dose accumulation for multicourse gynecological reirradiation: a methodological narrative and clinical examples. Int J Radiat Oncol Biol Phys. 2022;113(5):1085–1090. doi: 10.1016/j.ijrobp.2022.04.046.
- Swamidas J, Kirisits C, De Brabandere M, et al. Image registration, contour propagation and dose accumulation of external beam and brachytherapy in gynecological radiotherapy. Radiother Oncol. 2020;143:1–11. doi: 10.1016/j.radonc.2019.08.023.
- Kim H, Lee YC, Benedict SH, et al. Dose summation strategies for external beam radiation therapy and brachytherapy in gynecologic malignancy: a review from the NRG oncology and NCTN medical physics subcommittees. Int J Radiat Oncol Biol Phys. 2021;111(4):999–1010. doi: 10.1016/j.ijrobp.2021.06.019.
- Brock KK, Mutic S, McNutt TR, et al. Use of image registration and fusion algorithms and techniques in radiotherapy: report of the AAPM radiation therapy committee task group no. 132. Med Phys. 2017;44(7):e43–76–e76. doi: 10.1002/mp.12256.
- Saenz DL, Kim H, Chen J, et al. The level of detail required in a deformable phantom to accurately perform quality assurance of deformable image registration. Phys Med Biol. 2016;61(17):6269–6280. doi: 10.1088/0031-9155/61/17/6269.
- Loi G, Fusella M, Lanzi E, et al. Performance of commercially available deformable image registration platforms for contour propagation using patient-based computational phantoms: a multi-institutional study. Med Phys. 2018;45(2):748–757. doi: 10.1002/mp.12737.
- Nie K, Chuang C, Kirby N, et al. Site-specific deformable imaging registration algorithm selection using patient-based simulated deformations: site-specific DIR selection using patient-based simulated deformations. Med Phys. 2013;40(4):041911. doi: 10.1118/1.4793723.
- Kubli A, Pukala J, Shah AP, et al. Variability in commercially available deformable image registration: a multi‐institution analysis using virtual head and neck phantoms. J Appl Clin Med Phys. 2021;22(5):89–96. doi: 10.1002/acm2.13242.
- Teo BK, Bonner Millar LP, Ding X, et al. Assessment of cumulative external beam and intracavitary brachytherapy organ doses in gynecologic cancers using deformable dose summation. Radiother Oncol. 2015;115(2):195–202. doi: 10.1016/j.radonc.2015.04.002.
- van Heerden LE, Houweling AC, Koedooder K, et al. Structure-based deformable image registration: added value for dose accumulation of external beam radiotherapy and brachytherapy in cervical cancer. Radiother Oncol. 2017;123(2):319–324. doi: 10.1016/j.radonc.2017.03.015.
- Kim H, Huq MS, Houser C, et al. Mapping of dose distribution from IMRT onto MRI-guided high dose rate brachytherapy using deformable image registration for cervical cancer treatments: preliminary study with commercially available software. J Contemp Brachytherapy. 2014;6(2):178–184. doi: 10.5114/jcb.2014.43240.
- Hayashi K, Isohashi F, Akino Y, et al. Estimation of the total rectal dose of radical external beam and intracavitary radiotherapy for uterine cervical cancer using the deformable image registration method. J Radiat Res. 2015;56(3):546–552. doi: 10.1093/jrr/rru127.
- Kadoya N, Miyasaka Y, Yamamoto T, et al. Evaluation of rectum and bladder dose accumulation from external beam radiotherapy and brachytherapy for cervical cancer using two different deformable image registration techniques. J Radiat Res. 2017;58(5):720–728. doi: 10.1093/jrr/rrx028.
- Pötter R, Haie-Meder C, Limbergen EV, et al. Recommendations from gynaecological (GYN) GEC ESTRO working group (II): concepts and terms in 3D image-based treatment planning in cervix cancer brachytherapy—3D dose volume parameters and aspects of 3D image-based anatomy, radiation physics, radiobiology. Radiother Oncol. 2006;78(1):67–77. doi: 10.1016/j.radonc.2005.11.014.
- van Heerden LE, Visser J, Koedooder K, et al. Role of deformable image registration for delivered dose accumulation of adaptive external beam radiation therapy and brachytherapy in cervical cancer. J Contemp Brachytherapy. 2018;10(6):542–550. doi: 10.5114/jcb.2018.79840.
- Lawson JD, Schreibmann E, Jani AB, et al. Quantitative evaluation of a cone-beam computed tomography-planning computed tomography deformable image registration method for adaptive radiation therapy. J Appl Clin Med Phys. 2007;8(4):96–113. doi: 10.1120/jacmp.v8i4.2432.
- Zambrano V, Furtado H, Fabri D, et al. Performance validation of deformable image registration in the pelvic region. J Radiat Res. 2013;54 Suppl 1(suppl 1):i120–8. doi: 10.1093/jrr/rrt045.
- Shi L, Chen Q, Barley S, et al. Benchmarking of deformable image registration for multiple anatomic sites using digital data sets with ground-truth deformation vector fields. Pract Radiat Oncol. 2021;11(5):404–414. doi: 10.1016/j.prro.2021.02.012.