Background
Radiotherapy of Hodgkin lymphoma has undergone a significant development with shrinkage of irradiated volumes and lowering of prescribed dose while maintaining a high cure rate [Citation1]. More advanced techniques such as conformal IMRT planning and delivery in deep inspiration breath hold have been applied with an associated reduction of dose to organs at risk [Citation2,Citation3]. Several studies have shown a dose-response relationship between dose to the heart, lungs and breast and late effects such as ischemic heart disease and secondary induced cancer for Hodgkin lymphoma patients [Citation4–6]. Especially due to a young age profile [Citation7] and favorable prognosis [Citation8], it is crucial to keep dose to organs at risk (OAR) at lowest possible level. A possible next step in the management of Hodgkin lymphoma is applying particle therapy for obtaining even lower OAR doses than possible with photon therapy [Citation9]. Studies suggest that proton therapy provides dosimetric advantages [Citation10–12], but the advantages are dependent on individual patient anatomy, target volume and location, and motion management (i.e., deep inspiration breath hold) [Citation13–15]. In addition, particle therapy is more susceptible to motion and anatomical changes necessitating caution and adaptive strategies to ensure sufficient target coverage and maintain disease control [Citation16,Citation17]. The clinical benefit of using particle therapy remains to be proven in clinical trials.
In Denmark, about 100 Hodgkin lymphoma patients per year receive radiotherapy. It has been decided by the national lymphoma group that referral to proton therapy should be based on an individual proton-photon plan comparison performed at the referring radiotherapy center [Citation18]. The specific criteria for proton referral have not yet been decided but will be a combination of dosimetric advantages and patient specifics, i.e., age, sex, etc. Regardless of the final criteria, it is important that the plan quality across the country is of a high and homogenous standard to ensure accuracy and equity of referral to proton therapy. Towards this aim, three planning workshops were arranged by the Danish National Center for Particle Therapy. This study evaluates the outcome of the workshops by comparing dose distributions and dose to organs at risk (OAR) between proton and photon plans prepared independently at each center, before the third and final workshop.
Materials & methods
The four participating centers created at least one photon and one proton plan for each case, except the proton center that only created proton plans. There were three cases labelled A, B and C, with a prescribed dose of 36 Gy in 18 fractions for case A and B, and 30 Gy in 15 fractions for case C. Patient A and C were males and patient B female.
For each case, there were 5–6 proton plans, and 4–5 photon plans in total (more than one participating dose planner allowed at each center). Patient A was simulated in free breathing while patient B and C were simulated in deep inspiration breath hold. One center used Pinnacle (Philips) treatment planning system for dose plan generation while the rest used Eclipse (Varian Medical Systems). All centers used the same delineation for targets and OARs and optimized according to the ALARA principle with ‘ideal’ constraints from the International Lymphoma Radiation Oncology Group (ILROG) proton guidelines shown in [Citation9]. Sufficient target coverage was prioritized above the dose constraints for OARs. Treatment technique (IMRT or VMAT) and target dose coverage for the photon plans were according to local guidelines at each center, while a common CTV-to-PTV margin of 5 mm was used for photon plans. Proton plans used multi-field optimization with mainly anterior-oblique fields and in addition a posterior field when the target extended cranially to the neck, posteriorly of the heart or in case of female patient where breast tissue is to be spared as much as possible (case B). All proton plans were robustly optimized with a range uncertainty of ±4.5% in combination with an isocenter shift of 0 and 5 mm in all directions resulting in all together 14 uncertainty scenarios. Combined range uncertainty and translations are not possible when using Pinnacle and therefore 8 scenarios were evaluated in this case. CTV coverage of D98%>95% for the nominal plan, D98%>90% for all scenarios, D98%>95% for 12/14 (Eclipse) or 7/8 scenarios (Pinnacle), and V110%<1 cm3 for the nominal plan, was required. A Mann-Whitney U test was used to compare dosimetric metrics between photon and proton plans. Calculations were performed using Microsoft Excel and a statistical significance level of p < 0.05 was used. The project and the use of data was approved in the Region of Southern Denmark (project No. 23/28721).
Table 1. ‘Ideal’ OAR constraints from the ILROG proton guidelines [Citation9].
Results
The dose distributions and field angles for selected proton and photon plans are shown in . The proton plans are for all cases more conformal, which is mostly evident in the sagittal and coronal planes where the 50% isodoses for the photon plans extends further in the dorsal direction. Furthermore, the transversal plane view suggests a higher level of lung sparing at the 50% isodoses, corresponding to 18 Gy for case A and B, and 15 Gy for case C.
Figure 1. Dose distribution of selected proton and photon plans for each case. The dose is shown as dose color wash from 50% to 110% as indicated by the scales shown to the left side. CTVs are shown as red contours and the beam angles are represented by the yellow lines.
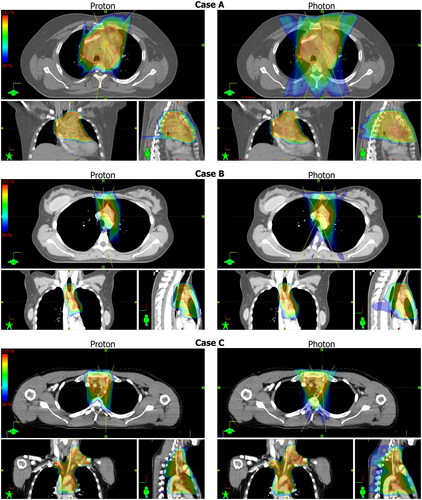
Boxplots of dose to OARs are shown in . In general, the variability and doses to OARs are lower for the proton plans. E.g., the median heart mean dose is reduced from 10.5 Gy (photon) to 5.9 Gy (proton) for case A, from 3.9 Gy to 2.2 Gy for case B and from 3.7 Gy to 2.6 Gy for case C.
Figure 2. Boxplots of dose to OARs for all proton and photon plans. The horizontal line represents the median, the boxes the interquartile range, and the whiskers the min/max values. OAR constraints are shown by the dotted horizontal lines. The breast mean dose (lower left corner) is shown for case B only, while the dose to the other OARs is shown for all cases (labelled A, B, and C). All metrics are statistically significantly different except lung V20Gy for patient B.
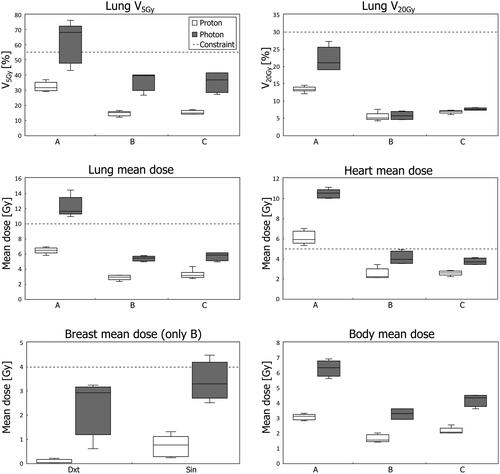
All metrics shown in are statistically significantly different except lung V20Gy for patient B. Maximum doses to the heart presented no significant difference (not shown in ).
Discussion
This dose planning comparison across four Danish centers showed that significantly lower OAR doses can be achieved by proton therapy compared to photon therapy for the three cases. This suggest that some patients might benefit from referral to proton therapy.
Furthermore, a lower variability in OAR doses indicates a high proton plan quality homogeneity which was the goal of the workshops. The higher variability for photon plans can be explained by the utilization of different planning techniques, i.e., IMRT vs. VMAT, but also local differences in choice of field angles and prioritization of target coverage vs. OAR sparing. For the proton plans, a common procedure limiting the degrees of freedom together with a consensus obtained during the workshops could explain the lower variability. A next step could be to arrange similar workshops for photon planning, to see if it is possible to increase the plan homogeneity to the same level as for the proton plans.
Which patients benefit most from proton therapy is not obvious because the dosimetric advantage is highly dependent on the individual anatomy as indicated by the three cases in this study. Furthermore, the clinical benefit depends on different factors like age and sex. Ideally, these factors are incorporated into validated normal tissue complication probability (NTCP) models in order to estimate the reduction in risk for complications [Citation19].
In the three cases, we observed a mean heart dose reduction by approximately 1–4 Gy. Based on existing models, irradiation of the heart is linked to a 7% increased risk of ischemic heart disease per Gy [Citation5]. Whether proton therapy facilitates a reduction of significant clinical benefit depends not only on dosimetry, but also on the age and sex of the patient since this determines the background risk [Citation20]. In general, the benefit is higher for younger patients because the accumulated risk of developing heart disease over the remaining duration of their life is higher. The background risk for ischemic heart disease is also higher for men than women therefore favoring men for proton therapy. For example, for a 30-year-old man the background risk of developing ischemic heart disease is around 23%, while for a 60-year-old woman it is about 7% [Citation20]. Assuming case A was a 30-year-old man, the risk would be reduced from 40% to 33% when treating with protons instead of photons. On the other hand, assuming case A was a 60-year-old woman, the risk reduction would be more modest from 13% to 11%. Lung and breast irradiation is associated with an increased risk of secondary induced cancer of 14–15% per Gy [Citation4, Citation6]. Assuming case A was a 30-year-old man, the risk of second lung cancer would be reduced from 15% to 11% when treating with protons instead of photons (background risk: 6%). On the other hand, assuming case A was a 60-year-old woman, the reduction would be from 10% to 7% (background risk: 4%). Correspondingly, the reduction in breast dose translates to a reduction in the risk of developing secondary induced breast cancer. Assuming case B was a 30-year-old woman this would therefore reduce this risk from 15–16% to 11–12% (background risk: 11%). It is worth noting that the models used in the above examples have several uncertainties, such as being based on data from treatments conducted before the common use of 3D dose planning and not incorporating data from proton therapy. Nonetheless, these models suggest that proton therapy has the potential to reduce late effects, but it will require investigation in clinical trials to determine the actual benefit. In addition, clinical trials can be used for validating NTCP models as those mentioned above. However, the latency of late effects necessitates many years of follow up.
This study is purely theoretical and even though proton therapy appears to be advantageous, it should be noted that proton treatments are much more susceptible to anatomical changes, variations in positioning and intrafractional motion. Therefore, proton treatment of mediastinal lymphomas requires not only robust plans, but also internal motion evaluation and adaptive strategies in order not to compromise disease control.
In conclusion, we have shown that it is possible to achieve a high and homogenous proton plan standard across different radiotherapy centers. This is important for consistent referral when the decision is based on a comparative proton-photon plan.
Disclosure statement
No potential conflict of interest was reported by the author(s).
Data availability statement
The data that support the findings of this study are available from the corresponding author, upon reasonable request.
Correction Statement
This article has been corrected with minor changes. These changes do not impact the academic content of the article.
References
- Specht L, Yahalom J, Illidge T, et al. Modern radiation therapy for Hodgkin lymphoma: field and dose guidelines from the international lymphoma radiation oncology group (ILROG). Int J Radiat Oncol Biol Phys. 2014;89(4):854–862. doi:10.1016/j.ijrobp.2013.05.005.
- Buglione M, Guerini AE, Filippi AR, et al. A systematic review on intensity modulated radiation therapy for mediastinal hodgkin’s lymphoma. Crit Rev Oncol Hematol. 2021;167:103437. doi:10.1016/j.critrevonc.2021.103437.
- Aznar MC, Maraldo MV, Schut DA, et al. Minimizing late effects for patients with mediastinal hodgkin lymphoma: deep inspiration breath-hold, IMRT, or both? Int J Radiat Oncol Biol Phys. 2015;92(1):169–174. doi:10.1016/j.ijrobp.2015.01.013.
- Travis LB, Gospodarowicz M, Curtis RE, et al. Lung cancer following chemotherapy and radiotherapy for Hodgkin’s disease. J Natl Cancer Inst. 2002;94(3):182–192. doi:10.1093/jnci/94.3.182.
- van Nimwegen FA, Schaapveld M, Cutter DJ, et al. Radiation dose-response relationship for risk of coronary heart disease in survivors of Hodgkin lymphoma. J Clin Oncol. 2016;34(3):235–243. doi:10.1200/JCO.2015.63.4444.
- Travis LB, Hill DA, Dores GM, et al. Breast cancer following radiotherapy and chemotherapy among young women with Hodgkin disease. JAMA. 2003;290(4):465–475. doi:10.1001/jama.290.4.465.
- Jaglowski SM, Linden E, Termuhlen AM, et al. Lymphoma in adolescents and young adults. Semin Oncol. 2009;36(5):381–418. doi:10.1053/j.seminoncol.2009.07.009.
- National Cancer Institute. Cancer Stat Facts: Hodgkin Lymphoma. Surveillance, Epidemiology, and End Results Program (SEER). 2022. Available from: https://seer.cancer.gov/statfacts/html/hodg.html.
- Dabaja BS, Hoppe BS, Plastaras JP, et al. Proton therapy for adults with mediastinal lymphomas: the International Lymphoma Radiation Oncology Group guidelines. Blood. 2018;132(16):1635–1646. doi:10.1182/blood-2018-03-837633.
- Chera BS, Rodriguez C, Morris CG, et al. Dosimetric comparison of three different involved nodal irradiation techniques for stage II Hodgkin’s lymphoma patients: conventional radiotherapy, intensity-modulated radiotherapy, and three-dimensional proton radiotherapy. Int J Radiat Oncol Biol Phys. 2009;75(4):1173–1180. doi:10.1016/j.ijrobp.2008.12.048.
- Baues C, Marnitz S, Engert A, et al. Proton versus photon deep inspiration breath hold technique in patients with hodgkin lymphoma and mediastinal radiation. Radiat Oncol. 2018;13(1):122. doi:10.1186/s13014-018-1066-2.
- Hoppe BS, Flampouri S, Su Z, et al. Consolidative involved-node proton therapy for stage IA-IIIB mediastinal Hodgkin lymphoma: preliminary dosimetric outcomes from a phase II study. Int J Radiat Oncol Biol Phys. 2012;83(1):260–267. doi:10.1016/j.ijrobp.2011.06.1959.
- Edvardsson A, Kügele M, Alkner S, et al. Comparative treatment planning study for mediastinal Hodgkin’s lymphoma: impact on normal tissue dose using deep inspiration breath hold proton and photon therapy. Acta Oncol. 2019;58(1):95–104. doi:10.1080/0284186X.2018.1512153.
- Rechner LA, Maraldo MV, Vogelius IR, et al. Life years lost attributable to late effects after radiotherapy for early stage Hodgkin lymphoma: the impact of proton therapy and/or deep inspiration breath hold. Radiother Oncol. 2017;125(1):41–47. doi:10.1016/j.radonc.2017.07.033.
- Patel CG, Peterson J, Aznar M, et al. Systematic review for deep inspiration breath hold in proton therapy for mediastinal lymphoma: a PTCOG Lymphoma Subcommittee report and recommendations. Radiother Oncol. 2022;177:21–32. doi:10.1016/j.radonc.2022.10.003.
- Paganetti H, Botas P, Sharp GC, et al. Adaptive proton therapy. Phys Med Biol. 2021;66(22):22TR01. doi:10.1088/1361-6560/ac344f.
- Chang JY, Zhang X, Knopf A, et al. Consensus guidelines for implementing pencil-beam scanning proton therapy for thoracic malignancies on behalf of the PTCOG thoracic and lymphoma subcommittee. Int J Radiat Oncol Biol Phys. 2017;99(1):41–50. doi:10.1016/j.ijrobp.2017.05.014.
- DLG: Kliniske retningslinjer for Hodgkin lymfom, version 1.0. 2022. Available from: https://www.lymphoma.dk/retningslinjer.
- Vega RBM, Mohammadi H, Patel SI, et al. Establishing cost-effective allocation of proton therapy for patients with mediastinal Hodgkin lymphoma. Int J Radiat Oncol Biol Phys. 2022;112(1):158–166. doi:10.1016/j.ijrobp.2021.07.1711.
- Centers for Disease Control and Prevention (CDC ). Prevalence of coronary heart disease–United States, 2006–2010. MMWR Morb Mortal Wkly Rep. 2011;60(40):1377–1381.