Abstract
At global level, the pandemic coronavirus disease 2019 (COVID-19) is known to be caused by an etiologic agent severe acute respiratory syndrome coronavirus 2 (SARS-CoV-2). Numerous evidence and propositions have emerged on the molecular and cellular attributes that cause COVID-19. Notwithstanding, still several key questions with reference to molecular aspects of severity of infection by SARS-CoV-2 need to be answered. In the same line, the role of healthy mitochondria to maintain intracellular temperature and their association with the severity of SARS-CoV-2 is completely missing. In this direction, preclinical and clinical data on the comorbidities in the case of mitochondrial defective disease and COVID-19 are not available. The authors propose that patients harboring primary mitochondrial disease and secondary mitochondrial dysfunction will display a higher severity and death rate compared to healthy mitochondria harboring patients.
Introduction
In every sphere of life, coronavirus disease 2019 (COVID-19) as a respiratory disease has created health disasters. Several clinical studies have suggested that COVID-19 is led by a novel coronavirus that shares structural features with the virus that causes severe acute respiratory syndrome (SARS)Citation1–7. Several models have proposed the infection phase starting from binding of SARS-CoV-2 to ACE-2 expressing nasal epithelial cells in the upper respiratory tract that is referred to as initial infection as presymptomatic/asymptomatic stageCitation5–10. Further, SARS-CoV-2 infects ACE-2 expressing alveolar epithelial cells as an early phase symptomatic stage. In spite of emerging etiologies of SARS-CoV-2, there is a lack of information on the role of functional mitochondria that maintains the intracellular temperature and severity of infections in the case of COVID-19 patients.
In the current scenario, there is progress in the preclinical and clinical aspects of COVID-19. However, at intracellular and intercellular levels, understanding is limited that may predispose to the severity and fatality by SARS-CoV-2. Organization of various cellular organelles including mitochondria and their link with the replication and assembly of SARS-CoV-2 in the infected epithelial cells are not well studied.
To have a proof of concept on the role of mitochondrial functionality that maintains the intracellular temperature, a proposition is warranted to collect data on the preclinical and clinical cases with severity and comorbidities in view of co-occurrence of COVID-19 and primary mitochondrial disease/secondary mitochondrial dysfunction. The key highlights of this paper is bringing awareness and sensitization among basic scientists, preclinical, clinical, epidemiologists, and health service provides including National and International agencies including WHO to realize the need to create a platform so that better diagnostics and therapeutics can be delivered to patients having the co-occurrence of mitochondrial related diseases and COVID-19. The importance of this paper should be limited only to COVID-19, but potentially link with any respiratory virus diseases to vulnerable populations specifically harboring mitochondrial diseases of primary and secondary in nature.
Mitochondrial dysfunction and human diseases
Mitochondria is known as a power of cell and a hub for an intracellular signaling pathway that controls various cellular processes including metabolic and death processesCitation11–13. Functional mitochondria are a key to normal physiological processes. Conversely, dysfunctional and defective mitochondria are linked to various human diseases including viral infections, cancer, and metabolic disordersCitation13–17.
Among the various human disease conditions, primary mitochondrial disorders (PMDs) are considered as inherited and genetic defects due to germline mutations in mitochondrial DNA (mtDNA) and/or nuclear DNA (nDNA) genes that encode proteins involved in oxidative phosphorylationCitation13–20. In the context of PMDs, notable ones are mitochondrial myopathy, Leber’s hereditary optic neuropathy (LHON), Leigh syndrome, and MERRF syndrome and other disorders. Among many PMDs, mitochondrial myopathies affect the debilitation of skeletal muscle and also respiratory muscle of lung airway and quadriceps. Such PMDs in the form of mitochondrial dysfunction in the respiratory muscle in the lung may have relevance that may explain the respiratory failure in the COVID-19 patients as an outcome and may serve as indirect evidence of mitochondrial dysfunction and COVID-19 outcomeCitation21–25.
Secondary mitochondrial dysfunction in human diseases are known to refer to a number of different genetic disorders that includes ethylmalonic aciduria (caused by mutation of ETHE1), Friedreich ataxia (FXN), and hereditary spastic paraplegia 7 (SPG7), Wilson disease (ATP7B), aging, cancer, and lung fibrosisCitation15–20,Citation26,Citation27. Additionally, mitochondrial disorders could be developed as acquired or induced by drugs and other stress factors. In fact, aging is also seen as a secondary mitochondrial dysfunction and the link between aging and COVID-19 is evident in the literature. Importantly, the secondary mitochondrial disorders such as lung COPD, idiopathic pulmonary fibrosis (IPF), cancer, or acute respiratory distress syndrome (ARDS) in relation to old age and COVID-19 severity, and this could be seen as indirect clinical evidence of mitochondrial functions and COVID-19 outcomeCitation28–31. Altogether, PMDs and secondary mitochondrial disorders with the mitochondrial dysfunction need molecular, cellular, preclinical, and clinical approaches to understand the effects of altered intracellular and extracellular temperature in the lung tissues in relation to the binding, replication, and assembly of SARS-C0V-2 that is known to influence the COVID-19 outcome.
In such pathophysiological conditions, mitochondria display significant aberrations in the ATP productionCitation13–17. Additionally, secondary mitochondrial diseases are also linked with mitochondrial dysfunctions with a defective electron transport chain and reduced ATP production. In fact, during primary and secondary mitochondrial disease conditions, cellular metabolic profile shifts from mitochondria-based to glycolysis-based energy production. Globally, one in 5,000 individuals have primary mitochondrial disease conditions as inherited disordersCitation11–17. Among the secondary mitochondria disease conditions, various diseases such as diabetes, cancer, Alzheimer’s disease, muscular dystrophy, and Lou Gehrig’s disease. Therefore, disturbances in the tissue homeostasis impact various external factors including virus infection and that may lead to the mitochondrial dysfunction and subsequent metabolic reprogramming of infected cells.
Mitochondrial function and intracellular temperature
The role of mitochondria in cellular functions is well studied and includes generation of ATP, metabolites, calcium homeostasis, retrograde signaling to nucleus and maintenance of intracellular temperature through dissipation of energyCitation32–36. In other ways, mitochondria as a tiny power house is capable of ATP generation and during the generation of ATP, temperature within the mitochondria and in the close vicinity is significantly high at the intracellular levelCitation18–20,Citation26,Citation27,Citation37–39.
An interesting paper made clear experimental observations on the distinctive intracellular temperature in the case of functional mitochondria over depleted mitochondrial DNA or treated with respiratory inhibitors. It is important to note that measurement of intracellular temperature by using a fluorescent probe indicated that human embryonic kidney (HEK) 293 cells and primary skin fibroblasts maintained 10 °C higher, which is close to a 50 °C temperature over defective mitochondria functionCitation18. In literature, there are reports on the intracellular temperature measurement with reference to mitochondrial status. But, significant gaps are observed to substantiate the data on the tissues and organs by using in vivo and ex vivo models. Furthermore, estimation of intracellular and extracellular temperature in cases of tissues and organs of COVID-19 patients is lacking. In fact, these would be interesting experiments to measure the temperature of these tissues and organs with reference to COVID-19 patients and importantly with various classifications of mitochondrial disorders including primary and secondary mitochondrial diseases.
Another key measurement technique by employing upconversion nanoparticles (UCNPs) provided convincing evidence of significantly high intracellular temperature in HeLa cells during in situ temperature measurementCitation37. These promising temperature measurements by fluorescent probe and nanoparticle based tools will facilitate the need of direct measurement of temperature in vitro, in situ, in vivo, and ex vivo for various human disease models including COVID-19 so that a clear correlation can be made regarding the status of mitochondria in affected cells of tissues/organs with a clear demarcation of intracellular and extracellular temperature. In future, measurement of intracellular and extracellular temperature of affected tissues and organs of COVID-19 patients with a category of mitochondrial disorders and healthy mitochondria will shed light on the molecular pathogenesis by SARS-CoV-2 in terms of molecular mechanism of cellular machinery that is hijacked by SARS-CoV-2.
Literature is limited in the case of in vivo and ex vivo that clearly measured the temperature of living cells, tissues, and organs of COVID-19 patients in various stages of pathogenesis. However, limited data emerged on the measurement of body temperature in the case of COVID-19 patients. Data suggested that among all COVID-19 patients, variable body temperature was recorded and around 31% of patients showed >100.4 °F/38 °C body temperature. There is a clear indication on the effects of temperature on the SARS-CoV-2 binding to ACE2 and also the replication and assembly of viruses. Therefore, an indirect relation between differential temperature and SARS-CoV-2 pathogenesis is being perceived. Such study proposed that all COVID-19 patients do not present the high temperature and the reasons could be linked with many aspects, including the status of mitochondrial functionality.
Molecular propositions to link and mitochondria and COVID-19
The role of mitochondria has emerged in various cellular processes including energy demands, death signals, and pathological stress. Hence, mitochondria are seen at the center of tissue homeostasis during normal and disease conditionsCitation11–13. Based on the above understanding, we make a proposition that mitochondrial defects and dysfunction in primary and secondary mitochondrial disease conditions may achieve intracellular environment that may support the replication and assembly of SARS-CoV-2 in specific cells such as nasal epithelial cells and alveolar epithelial cells. In this way, the severity of SARS-CoV-2 among COVID-19 patients with primary and secondary mitochondrial disease conditions is potentially linked to high replication and assembly of SARS-CoV-2.
Energy generated by mitochondria is used up to 40% for ATP production and 60% of energy is dissipated as heat to serve as the thermostatic radiators to maintain the required intracellular and extracellular temperature gradientCitation18–20,Citation26,Citation27. There is a well-accepted view on the dynamic and gradient based maintenance of intracellular temperature including a compartment close to ERCitation40–44. Therefore, it is logical to propose the role of healthy and functional mitochondria in the maintenance of intracellular temperature gradients across from the nucleus to the close vicinity of ER and most importantly dynamic in nature.
A possible explanation is that mitochondria is well-connected to the endoplasmic reticulum for various intracellular signaling cascades including calcium signalingCitation40–44. Such intracellular compartmentalization of cells may be a well-articulated cellular landscape to achieve various cellular processes including translation and assembly of organelle structure. Therefore, inter-organelle communication is key for the normal cellular landscape.
Furthermore, a pertinent question is asked on the number of mitochondria in a nasal epithelial cell that are targets of SARS-CoV-2. Based on the existing understanding, there are a large number of functional mitochondria in epithelial cells, including nasal, alveolar, and intestinal tissuesCitation14–20,Citation26. Besides the number of mitochondria, an insight on the ratio between mitochondrial ATP production and glycolytic ATP production is also important. Existing data support that glycolytic ATP production increases during physiological stress, including mitochondrial dysfunctionCitation14–17.
In view of the pivotal role of mitochondria generated heat and temperature gradient at intracellular and extracellular levels, a proposition is logical that changes in the mitochondrial function may compromise the temperature gradient. Such cellular physiology may be linked to the physiological stress, including the replication and assembly of SARS-CoV-2 in specific cells such as nasal epithelial cells and alveolar epithelial cells that are primary targets of SARS-CoV-2. Furthermore, there is evidence that temperature is one of the potential factors behind the stability and assembly of SARS-CoV-2 at the intracellular and extracellular levels. Hence, changes in the temperature at intracellular and extracellular levels in the case of nasal epithelial cells and alveolar epithelial cells can contribute towards the entry and intracellular stability and assembly of SARS-CoV-2. Besides the role of dysfunctional mitochondria in bringing the intracellular temperature to more favorable for growth and assembly of SARS-CoV-2, deregulated cellular energetics may help the severity of SARS-CoV-2 in other intracellular signaling pathways, including calcium, chaperone, and ER stress.
Furthermore, highly recent papers have suggested that, among highly severe and fatal cases due to SARS-CoV-2, 15% of such cases show the inborn error of interferon immunityCitation45,Citation46. In fact, such findings are novel and the first of their kind to link SARS-CoV-2 infection and inborn error of immunity among the exposed human population. In this line of evidence, our proposition is warranted to explore the contribution of mitochondrial dysfunction including both inherited/inborn genetic mitochondrial diseases and secondary mitochondrial dysfunction as predisposition factors behind the severe and fatal SARS-CoV-2 infected patients. An interesting paper presented views on the contributions of intra and extracellular function of mitochondrial function in the pathogenicity of COVID-19Citation47.
Taken together, the outcome of COVID-19 is suggested to be multifactorial in nature, including intracellular (mitochondrial dysfunction), intercellular (levels of platelets, immune cells), tissue heterogeneity (gut microbiome), dietary factors (Vitamin C, Vitamin D, Calcium), and various other environmental factors including emotional and social factorsCitation32–36,Citation42–49. This paper discussed the link among cytokine storm, mitochondrial oxidative stress, and also microbiota dysbiosis that aggravate the severity of COVID-19. Our proposition is well supported by the views presented by Saleh et al.Citation47 A proposed model is illustrated in and .
Figure 1. A proposed model on the link between defective mitochondria regulated intracellular temperature and high severity of COVID-19. In the case of alveolar epithelial cells, defective mitochondria potentially are not able to contribute towards intracellular temperature. In fact, intracellular temperature is proposed to be lower compared to the alveolar epithelial cells with healthy and functional mitochondria.
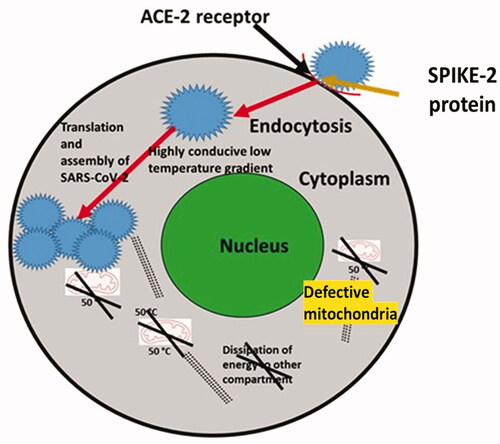
Figure 2. A proposed model on the link between healthy mitochondria regulated intracellular temperature and less severity of COVID-19. Alveolar epithelial cells with healthy and functional mitochondria maintain slightly higher temperature than the alveolar epithelial cells with defective mitochondria. Here, the proposed model suggests that an optimal intracellular temperature of alveolar epithelial cells with good mitochondrial may not be a better intracellular environment for the replication and assembly of SARS-CoV-2 compared to alveolar epithelial cells harboring defective mitochondria.
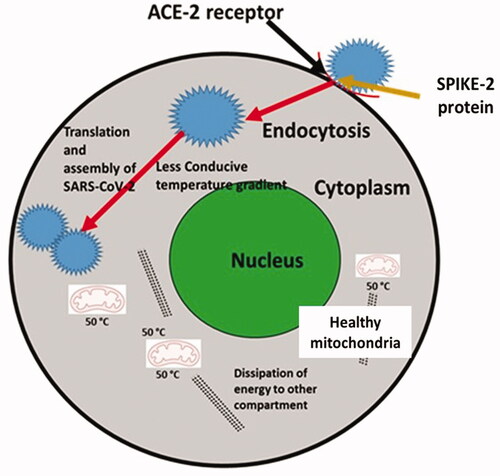
Mitochondrial functionality and cytokine storm (inflammation)
Mitochondrial function is a key factor that contributes to the metabolic reprogramming in health and various disease conditions including cancer, metabolic disorders, and aging and virus diseasesCitation12–18. Cytokine storm, one of the hallmarks of COVID-19, is known for creating heightened inflammation in COVID-19 patients, specifically higher implications in the old age populationCitation5–8. It is interesting to note that cytokine storms may contribute to inflammation during aging and this may be potentially mediated due to the compromised mitochondrial vitality due to various factors including oxidative stress, environmental chemicals, and biological agents including viruses. However, data is limited in terms of other than aging, the implications of primary and secondary mitochondrial disorders in the exposed populations, because the severity of COVID-19 among the old age population is differential. Therefore, direct and indirect evidence on the intracellular and extracellular temperature of affected cells due to SARS-CoV-2 could be correlated with three important factors: cytokine storm mediated inflammation, aging, and the status of mitochondria. Other views are presented in terms of effects of good mitochondria due to physical activity and more resistance to the cytokine storm associated SARS-CoV-2 implicationsCitation21,Citation37–39,Citation50. This is well discussed in the case of bats that show resistance and their association with better environmental adaptations with high physical activities that help them to maintain a higher function of mitochondria.
Indirect clinical association between mitochondrial disorders and COVID-19 outcome
A small study group projected the association of COVID-19 related outcomes in the case of PMDs in terms of hospitalization and respiratory dysfunctionCitation25. This study opened up discussion on the involvement of functional mitochondria in the molecular pathogenesis of SARS-CoV-2 including the intracellular and extracellular environment such as temperature that can modulate the SARS-CoV-2 associated molecular interactions. Another viewpoint is relevant with regard to the metabolic disorders in lung and COVID-19 outcomes. There is an understanding that metabolic syndrome and lung diseases share common molecular pathways that may predispose to respiratory symptoms, greater lung function impairment, pulmonary hypertension, and asthma. On the other hand, COVID-19 is known to cause respiratory symptoms and greater lung function impairment. Interestingly, growing views have surfaced to connect the metabolic syndrome and abnormalities with the pathogenic mitochondrial dysfunction. Therefore, looking at the molecular pathogenesis of COVID-19, the involvement of mitochondria controlled intracellular and extracellular temperature environment needs more attention and debate with suitable in vitro, in vivo, and ex vivo studies that will help to establish better understating in these growing interests.
Preclinical and clinical perspectives
The proposed views and experimental approaches suggest a new dimension on the relevance of mitochondrial function and intracellular temperature within the nasal and alveolar epithelial cells of patients exposed to SARS-CoV-2. Such cellular understanding paves the way for taking into consideration such heterogeneity at mitochondria and cellular levels during any pharmaceutical interventions. The proposed views may bring in future biomarkers related to the COVID-19 and mitochondrial disease dysfunctions. Additionally, proposed experimental approaches may help to explore pharmaceutical interventions in the form of dietary mimetic to enhance the mitochondrial function and intracellular temperature in the case of COVID-19 exposed patients. One of the limitations in clinical studies centered on mitochondrial dysfunction is lack of sufficient patients with mitochondrial dysfunction and SARS-CoV-2 infected patients. Besides clinical studies, the established animal model on mitochondrial dysfunction and COVID-19 is a limitation. In summary, research communities focusing on molecular, preclinical, and clinical aspects on mitochondrial dysfunction including genetic mitochondrial diseases and secondary mitochondrial dysfunction need to be strengthened so a better connection between the scientific world and social world may be achieved. Besides some developed countries, awareness on the mitochondrial related genetic diseases is not appreciable in developing countries including India. Therefore, genetic and molecular based testing on mitochondrial disorders and their potential link with the severity of COVID-19 should be one of the focuses of healthcare services run by the National and International agencies.
Futuristic experiments
Epidemiological data is proposed to show the co-occurrence of COVID-19 and mitochondrial disease that may bring the severity and comorbidities. Data will establish the correlation between two pathophysiological conditions; COVID-19 and patients with mitochondrial disease. An experiment may be proposed at in vitro level with the help of nasal and alveolar epithelial cells with functional mitochondria and depleted mitochondria to see the effects of infection by SARS-CoV-2. Furthermore, use of fluorescence thermometer probes may be used to measure the intracellular temperature in the case of nasal and alveolar epithelial cells infected by SARS-CoV-2 in both settings with functional mitochondria and depleted mitochondrial DNACitation32–36. During the in vitro experiment, we propose to study the replication and assembly efficiency of SARS-CoV-2 in the case of nasal and alveolar epithelial cells infected by SARS-CoV-2. Furthermore, evaluation of mitochondrial functionality and SARS-CoV-2 replication and assembly is proposed by the use of mitochondria depleted nasal and alveolar epithelial cells. Besides the above proposed experiments, understanding on the mitochondrial functionality and COVID-19 pathogenesis may be translated in the form of drugs that can strengthen the biogenesis of healthy mitochondria in the case of patients with bad/unhealthy mitochondria.
Transparency
Declaration of financial/other relationships
The authors declare that they have no conflict of interest. Peer reviewers on this manuscript have no relevant financial or other relationships to disclose.
Acknowledgements
The authors acknowledge financial support from Dr. D.Y. Patil Vidyapeeth, Pune, India (DPU/05/01/2016).
Additional information
Funding
References
- Arvin AM, Fink K, Schmid MA, et al. A perspective on potential antibody-dependent enhancement of SARS-CoV-2. Nature. 2020;584(7821):353–363. https://doi.org/10.1038/s41586-020-2538-8.
- Gupta A, Madhavan MV, Sehgal K, et al. Extrapulmonary manifestations of COVID-19. Nat Med. 2020;26(7):1017–1032.
- Harmooshi NN, Shirbandi K, Rahim F. Environmental concern regarding the effect of humidity and temperature on 2019-nCoV survival: fact or fiction. Environ Sci Pollut Res Int. 2020;27(29):36027–36010.
- Le Bert N, Tan AT, Kunasegaran K, et al. SARS-CoV-2-specific T cell immunity in cases of COVID-19 and SARS, and uninfected controls. Nature. 2020;584(7821):457–462. https://doi.org/10.1038/s41586-020-2550-z.
- Matheson NJ, Lehner PJ. How does SARS-CoV-2 cause COVID-19? Science. 2020;369(6503):510–511.
- Shang J, Wan Y, Luo C, et al. Cell entry mechanisms of SARS-CoV-2. Proc Natl Acad Sci U S A. 2020;117(21):11727–11734.
- Wiersinga WJ, Rhodes A, Cheng AC, et al. Pathophysiology, transmission, diagnosis, and treatment of Coronavirus Disease 2019 (COVID-19). A Review JAMA. 2020;324(8):782–793. https://doi.org/10.1001/jama.2020.12839.
- Li W, Moore MJ, Vasilieva N, et al. Angiotensin-converting enzyme 2 is a functional receptor for the SARS coronavirus. Nature. 2003;426(6965):450–454.
- Hoffmann M, Kleine-Weber H, Schroeder S, et al. SARS-CoV-2 cell entry depends on ACE2 and TMPRSS2 and is blocked by a clinically proven protease inhibitor. Cell. 2020;181(2):271–280.e8.
- Sungnak W, Huang N, Bécavin C, et al. SARS-CoV-2 entry factors are highly expressed in nasal epithelial cells together with innate immune genes. Nat Med. 2020;26(5):681–687.
- Niyazov DM, Kahler SG, Frye RE. Primary mitochondrial disease and secondary mitochondrial dysfunction: importance of distinction for diagnosis and treatment. Mol Syndromol. 2016;7(3):122–137.
- Costa R, Peruzzo R, Bachmann M, et al. Impaired mitochondrial ATP production downregulates wnt signaling via ER stress induction. Cell Rep. 2019;28(8):1949–1960.
- Picard M, Sandi C. The social nature of mitochondria: implications for human health. Neurosci Biobehav Rev. 2021;120:595–610.
- Nakagawa K, Lokugamage KG, Makino S. Viral and cellular mRNA translation in Coronavirus-Infected cells. Adv Virus Res. 2016;96:165–192.
- Picard M, McEwen BS, Epel ES, et al. An energetic view of stress: focus on mitochondria. Front Neuroendocrinol. 2018;49:72–85.
- Kosmider B, Lin CR, Karim L, et al. Mitochondrial dysfunction in human primary alveolar type II cells in emphysema. EBioMedicine. 2019;46:305–316.
- Yoon YH, Yeon SH, Choi MR, et al. Altered mitochondrial functions and morphologies in epithelial cells are associated with pathogenesis of chronic rhinosinusitis with Nasal polyps. Allergy Asthma Immunol Res. 2020;12(4):653–668.
- Birceanu O. Mitochondria are too hot to handle!. J Exp Biol. 2018;221(9):jeb170027.
- Chrétien D, Bénit P, Ha HH, et al. Mitochondria are physiologically maintained at close to 50 °C. PLoS Biol. 2018;16(1):e2003992.
- Howard R, Scheiner A, Cunningham J, et al. Cytoplasmic convection currents and intracellular temperature gradients. PLoS Comput Biol. 2019;15(11):e1007372.
- Richardson S, Hirsch JS, Narasimhan M, et al. Presenting characteristics, comorbidities, and outcomes among 5700 patients hospitalized with COVID-19 in the New York City Area. JAMA. 2020;323(20):2052–2059.
- Nunn AVW, Guy GW, Brysch W, et al. SARS-CoV-2 and mitochondrial health: implications of lifestyle and ageing. Immun Ageing. 2020;17(1):33.
- Stefan N, Birkenfeld AL, Schulze MB, et al. Obesity and impaired metabolic health in patients with COVID-19. Nat Rev Endocrinol. 2020;16(7):341–342.
- Wang M, Baker JS, Quan W, et al. A preventive role of exercise across the coronavirus 2 (SARS-CoV-2) pandemic. Front Physiol. 2020;11(1139):572718.
- Pizzamiglio C, Machado PM, Thomas RH, et al. COVID-19-Related outcomes in primary mitochondrial diseases: an international study. Neurology. 2022;98(14):576–582. https://doi.org/10.1212/WNL.0000000000200240.
- Kang JS. Theoretical model and characteristics of mitochondrial thermogenesis. Biophys Rep. 2018;4(2):63–67.
- Nasr MA, Dovbeshko GI, Bearne SL, et al. Heat shock proteins in the “hot” mitochondrion: identity and putative roles. BioEssays. 2019;41(9):1900055.
- Ito K, Barnes PJ. COPD as a disease of accelerated lung aging. Chest. 2009;135(1):173–180.
- Raghu G, Weycker D, Edelsberg J, et al. Incidence and prevalence of idiopathic pulmonary fibrosis. Am J Respir Crit Care Med. 2006;174(7):810–816.
- Rubenfeld GD, Caldwell E, Peabody E, et al. Incidence and outcomes of acute lung injury. N Engl J Med. 2005;353(16):1685–1693.
- Cloonan SM, Choi AM. Mitochondria in lung disease. J Clin Invest. 2016;126(3):809–820.
- Okabe K, Inada N, Gota C, et al. Intracellular temperature mapping with a fluorescent polymeric thermometer and fluorescence lifetime imaging microscopy. Nat Commun. 2012;3:705.
- Kucsko G, Maurer PC, Yao NY, et al. Nanometre-scale thermometry in a living cell. Nature. 2013;500(7460):54–58.
- Hayashi T, Fukuda N, Uchiyama S, et al. A cell-permeable fluorescent polymeric thermometer for intracellular temperature mapping in mammalian cell lines. PLoS One. 2015;10(2):e0117677.
- Inada N, Fukuda N, Hayashi T, et al. Temperature imaging using a cationic linear fluorescent polymeric thermometer and fluorescence lifetime imaging microscopy. Nat Protoc. 2019;14(4):1293–1321.
- Sugimura T, Kajimoto S, Nakabayashi T. Label-Free imaging of intracellular temperature by using the O-H stretching Raman band of water. Angew Chem Int Ed Engl. 2020;59(20):7755–7760.
- Di X, Wang D, Zhou J, et al. Quantitatively monitoring in situ mitochondrial thermal dynamics by upconversion nanoparticles. Nano Lett. 2021;21(4):1651–1658.
- Qiao J, Chen C, Shangguan D, et al. Simultaneous monitoring of mitochondrial temperature and ATP fluctuation using fluorescent probes in living cells. Anal Chem. 2018;90(21):12553–12558.
- Rajagopal MC, Brown JW, Gelda D, et al. Transient heat release during induced mitochondrial proton uncoupling. Commun Biol. 2019;2:279.
- Inoue T, Tsai B. How viruses use the endoplasmic reticulum for entry, replication, and assembly. Cold Spring Harb Perspect Biol. 2013;5(1):a013250..
- Csordás G, Weaver D, Hajnóczky G. Endoplasmic Reticulum-Mitochondrial contactology: structure and signaling functions. Trends Cell Biol. 2018;28(7):523–540.
- Balaban RS. How hot are single cells? J Gen Physiol. 2020;152(8):e202012629.
- Mavin E, Verdon B, Carrie S, et al. Real-time measurement of cellular bioenergetics in fully differentiated human nasal epithelial cells grown at air-liquid-interface. Am J Physiol Lung Cell Mol Physiol. 2020;318(6):L1158–L1164.
- Suzuki M, Plakhotnik T. The challenge of intracellular temperature. Biophys Rev. 2020;14:1–8.
- Bastard P, Rosen LB, Zhang Q, et al. Type I IFNs in patients with life-threatening COVID-19. Science. 2020;370(6515):eabd4585.
- Zhang Q, Bastard P, Liu Z, et al. Inborn errors of type I IFN immunity in patients with life-threatening COVID-19. Science. 2020;370(6515):abd4570.
- Saleh J, Peyssonnaux C, Singh KK, et al. Mitochondria and microbiota dysfunction in COVID-19 pathogenesis. Mitochondrion. 2020;54:1–7.
- Bókkon I, Kapócs G, Vucskits A, et al. COVID-19: the significance of platelets, mitochondria, vitamin D, serotonin and the gut microbiota. Curr Med Chem. 2021;28(37):7634–7657.
- Singh P, Mishra N, Singh N, et al. Credible protein targets and curative strategies for COVID-19: a review. SN Compr Clin Med. 2020;25:1–10.
- Zhou Z, Yang Z, Ou J, et al. Temperature dependence of the SARS-CoV-2 affinity to human ACE2 determines COVID-19 progression and clinical outcome. Comput Struct Biotechnol J. 2021;19:161–167.