Abstract
Anemia is a common complication in patients with hemodialysis-dependent chronic kidney disease (HDD-CKD). Anemia is principally the result of erythropoietin deficiency, inflammation, and iron deficiency. High molecular weight iron oxide nanoparticles (IONP) are routinely administered intravenously to replace iron losses and, although effective, there are lingering concerns about possible safety issues. Ferric pyrophosphate citrate (FPC, Triferic, Triferic AVNU [Triferic and Triferic AVNU are the proprietary name for ferric pyrophosphate citrate. Triferic and Triferic AVNU are registered trademarks of Rockwell medical Inc.]) is a complex iron salt that donates iron directly to plasma transferrin. FPC is devoid of any carbohydrate moiety and is administered via the dialysate or intravenously during each hemodialysis session to replace iron and maintain hemoglobin. Controlled clinical trials of up to 48 weeks in duration have demonstrated the efficacy of regular administration of dialysate FPC for maintaining hemoglobin levels and iron balance in HDD-CKD patients. Clinical data also suggest that dialysate FPC may reduce the dose requirements for and use of erythropoiesis-stimulating agents and IONPs in HDD-CKD patients. Safety data from clinical studies and post-marketing surveillance show that FPC is well tolerated and not associated with an increased risk of infection, inflammation, iron overload, or serious hypersensitivity reactions. FPC represents an effective and well-tolerated choice for iron replacement and maintenance of hemoglobin in the long-term management of HDD-CKD patients.
Introduction
Iron replacement therapy is needed to manage anemia in patients with hemodialysis-dependent chronic kidney disease (HDD-CKD). Anemia in these patients is principally caused by a relative deficiency in erythropoietin, iron-restricted erythropoiesis (IRE) and uremic inflammation. IRE occurs as a result of gastrointestinal- and hemodialysis-associated blood losses and iron sequestration secondary to uremic inflammation. It is estimated that approximately 5–7 mg of iron needs to be administered to the majority of patients with each hemodialysis session to replace the average annual iron losses of 1–2 grams in HDD-CKD patientsCitation1,Citation2.
The current practice is to replace iron losses in HDD-CKD patients through repeated intravenous administration of iron oxide nanoparticles (IONP)Citation3. This is associated with safety issues, including risk of anaphylaxis/hypersensitivity reactionsCitation4, as well as a possible risk of tissue iron overloadCitation5. Other potential concerns include cardiovascular events, oxidative stress-induced atherosclerosis, and infectionCitation5,Citation6. Thus, there is a need for a more physiologic iron replacement compound that can maintain iron homeostasis in HDD-CKD patients by replacing ongoing iron losses.
Iron and anemia in CKD
Iron is the most conserved element in the human body and there are no significant iron-excretory pathways. Thus, body iron content is controlled by dietary absorptive mechanisms. In healthy humans, iron balance is maintained by recycling iron from senescent red blood cells. Only small quantities of iron are lost through sloughing of cells in the gastro-intestinal tract and these losses are balanced by absorption of iron from the duodenumCitation7. Iron deficiency in HD occurs with great frequency and is due to ongoing blood losses because of blood trapping in the dialyzer and bloodlines, frequent phlebotomy for laboratory testing and enhanced gastrointestinal lossesCitation8,Citation9. The average annual iron loss includes an annual loss of 500–600 mg of iron due to gastrointestinal bleeding exacerbated by uremic platelet dysfunctionCitation10,Citation11; an annual loss of approximately 200 mg of iron due to monthly blood draws (50 mL/month or approximately 0.6 liters annually) for routine biochemical analysesCitation12; and an annual loss of 300–1000 mg of iron due to retention of blood in the tubing and dialyzersCitation1,Citation12.
Systemic iron metabolism is normally a tightly regulated process primarily driven by the control of iron absorptive mechanisms and the distribution of iron among organs and tissues. Hepcidin decreases the absorption of iron across the duodenal mucosa and delivery of iron to transferrin from body stores by inactivating the cellular iron exporter ferroportinCitation13. Elevated hepcidin limits the iron export from the recycling of aged erythrocytes in macrophages, and the release of stored iron from hepatocytes. Circulating hepcidin is also increased by inflammation as well as kidney failure through reduced clearanceCitation14,Citation15.
Iron deficiency can be categorized, although somewhat arbitrarily, as absolute, or functional. Absolute iron deficiency is the consequence of diminished or absent iron stores and results in anemia due to a lack of available iron for incorporation into hemoglobin (IDA). In contrast, functional iron deficiency (FID), is a form of iron-restricted erythropoiesis in the presence of adequate amounts of stored iron that is resistant to mobilization for erythropoiesis. FID is a consequence of chronically elevated levels of serum hepcidin that reduce iron release from stores and decreased intestinal iron absorptionCitation16,Citation17. FID is also caused when erythropoietin increases the demand for iron and the rate at which iron can be mobilized is insufficient to meet the demandCitation18. When erythropoiesis-stimulating agents, inflammation and iron restriction coexist in HDD-CKD patients, IRE ensues followed by anemiaCitation19,Citation20.
Treatment of anemia in HD with iron
IONP utilize the red blood cell iron-recycling pathway to allow for large repletion doses of iron to be administered for correction of iron deficiency anemia. Due to the dysregulation of iron metabolism in CKD-HD patients, more iron than is required to maintain balance must be administered to improve hemoglobin (Hgb). This has led to potential iron overload as measured by increasing ferritin concentrations and evidence of increased iron storesCitation21,Citation22. While the clinical consequence of increased body iron is unclear, a more physiologic form of iron replacement would be desirable to maintain iron stores and hemoglobin while avoiding the possibility of excess iron due to the use of IONPsCitation22. Such a replacement compound should have the following characteristics. First, it should directly and effectively deliver iron to transferrin and thereby to the bone marrow for erythropoiesis, to maintain hemoglobin. Second, it should not require processing by macrophages or be subject to sequestration within the reticuloendothelial system. Unlike IONP’s, which independently elevate hepcidin, replacement of iron losses without increasing serum ferritin or hepcidin would effectively treat functional iron deficiencyCitation23. Third, it should facilitate the action of erythropoietic agents, including ESAs. Fourth, it should be safe for long-term use in HDD-CKD patients including home hemodialysis patients, with no contraindications for use and no risk of anaphylaxis, inflammation, or release of redox-active, plasma non-transferrin-bound iron (NTBI). Finally, it should be easy to administer by providers in the outpatient clinics and by patients in home settings.
FPC: a novel iron drug for hemoglobin maintenance
Soluble ferric pyrophosphate-citrate complexes, generally referred to as soluble ferric pyrophosphate (SFP) was first described in the mid-1800s by Robiquet and ChapmanCitation24,Citation25. This class of food-grade iron salts is available as an oral iron supplement and for fortification of food. Gupta et al. first demonstrated that food-grade SFP could be administered to hemodialysis patients via the dialysateCitation26. Pharmaceutical-grade ferric pyrophosphate citrate (FPC) was developed for parenteral administration as an iron supplement and approved in 2015 by the US Food and Drug Administration for the maintenance of hemoglobin in patients receiving chromic hemodialysis.
Chemical structure and mechanism of action
FPC is a highly water soluble (>1000 mg per milliliter of water), the protected iron compound in which citrate and pyrophosphate are tightly complexed to the iron (). It has a molecular formula of Fe4(C6H4O7)3(H2P2O7)2(P2O7) and relative molecular weight of approximately 1313 DaltonsCitation27. The molecular weight is similar to that of vitamin B12 and thus, FPC can diffuse across the hemodialyzer membrane. Both theoretical calculations and in vitro studies confirm tight chelation of the iron atoms in the solution. Ferric ion forms a tighter complex with pyrophosphate (PPi) than the competing cations that are present in the dialysate, such as sodium, potassium, calcium, and magnesium. The calculated Log Kstab for ferric pyrophosphate is 9.30, more than a million-fold more stable than other iron salts (i.e. ferric fumarate, sulfate, gluconate, and citrate)Citation28.
Figure 1. Chemical structure of FPC. FPC is an iron-pyrophosphate chelate. Iron is coordinated to two oxygens of pyrophosphate and four oxygens of citrate. The pyrophosphate moiety confers stability, and the citrate moiety confers water solubility. The molecular weight is estimated to be 1300 Da and the solubility is 1 gm FPC/gm water.
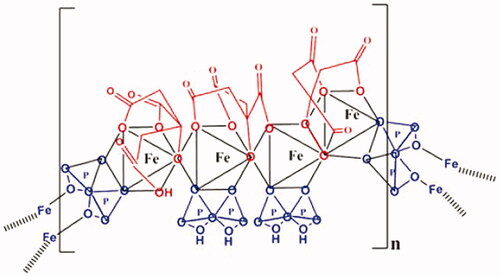
In-vitro kinetic studies of iron transfer from FPC to transferrin have demonstrated that iron loading of transferrin reaches 100% within 10 minCitation29. FPC iron co-crystallized with human apo-transferrin (2:1 molar ratio) shows iron donated to both the N- and C-lobe iron-binding sites. (PDB Structure library 6CTC) Iron in the C-lobe of transferrin is complexed with carbonate as the synergistic anion, while in the N-lobe, iron is complexed with pyrophosphate as the synergistic anionCitation30. The crystal structure of FPC-transferrin is a semi-open structure in the N-lobe (). When compared with fully loaded human iron-transferrin there is no impact on the transferrin receptor binding domain, so FPC-iron transferrin should not have any effect on the plasma clearance of di-ferric transferrinCitation31.
Figure 2. Crystal structure of FPC bound human transferrin. The 2.6 Å crystal structure of human apo-transferrin co-crystalized with FPC (PDB-6CTC). Pratt et al.Citation29 iron in the N-lobe of Tf has pyrophosphate as the synergistic anion. The iron in the C-lobe contains has to carbonate as the synergistic anion. Iron atoms are shown as orange spheres. Pyrophosphate and carbonate are depicted as stick figures.
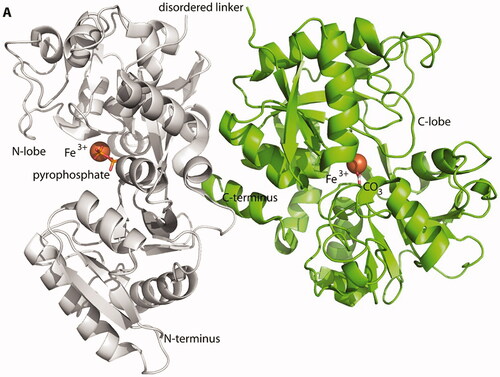
The mechanism of iron delivery by FPC is unique for a parenteral iron compound, and similar to that of native diet-derived iron. When administered to the circulation, FPC donates its iron directly and completely to circulating transferrin which then can deliver iron to erythroid precursors in the bone marrow for incorporation into hemoglobin. All of the administered FPC-iron is donated to transferrin as indicated by the concordance of total serum iron to transferrin-bound iron (TBI)Citation32. Non-transferrin-bound iron (NTBI) is negligible until the transferrin saturation (TSAT) exceeds 100%. This indicates the absence of labile plasma iron, the fraction of NTBI that is redox-active and believed to be a toxic speciesCitation33. We postulate that direct transfer of iron from FPC to transferrin can bypass hepcidin-induced iron sequestration in macrophages becoming 100% bioavailable for hemoglobin incorporation and delivery to other iron-requiring tissues.
Pharmacokinetics and administration of FPC
The pharmacokinetic (PK) profile of intravenous FPC is characterized by rapid linear increases in serum iron concentration and TSAT during infusion followed by rapid clearance from the circulation. The PK has been studied in healthy iron-replete subjects and in adult and pediatric HD patients. A single ascending dose study in healthy volunteers, investigated doses of 2.5, 5.0, 7.5, or 10 mg of FPC iron over 4 h or single doses of 15 or 20 mg of FPC iron over 12 h via intravenous infusionCitation32. Serum total iron (sFe) concentrations peaked at the end of infusion (Cmax) and then quickly returned to baseline values (). The mean time to maximum serum iron concentration (tmax) was 4.3–4.5 h, after intravenous administration of the 4-hour infusions and 8–9 h, respectively, after intravenous administration of the 15 and 20 mg doses over 12 h. Serum iron was rapidly cleared, with a mean apparent terminal phase half-life (t½app) of 1.2 h for the 4-hour infusion and 2.0 h for the 12-hour infusion. Serum hepcidin concentrations did not increase during the 4-hour infusions (2.5–10 mg Fe) but approximately doubled by the end of the 12-hour infusion (15 and 20 mg Fe). There was no increase in markers of oxidative stress or inflammation in any dose group. In-vitro studies show that plasma is free from redox-active iron until the IBC is exceeded. The dosing of FPC has been designed to avoid the potential to generate redox-active iron in the plasmaCitation32.
Figure 3. Time-zero corrected concentration-time plot of single ascending does of FPC in healthy volunteers. FPC was administered IV over 4 h at fixed doses in D5/W. Because endogenous iron concentrations are variable, time-zero corrected values were used to show the contribution of FPC iron to the serum iron. (A) Placebo-treated subjects showed a diurnal variation in serum iron. FPC iron administration showed dose proportional increases in Cmax and AUC. (B) Transferrin bound iron paralleled the sFe concentration. At the 10 mg FPC dose, TSAT at Cmax was 100 ± 4.0%.
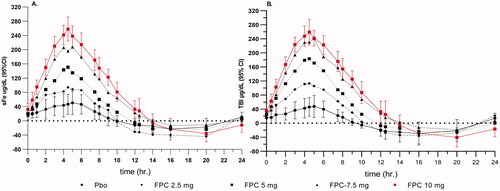
The FDA-approved dialysate concentration of FPC iron is 2 µM (110 µg Fe/L dialysate). The dialysate FPC is provided as one of two presentations: 5-mL ampules containing 27.2 mg of iron (III) per ampule (5.44 mg of iron (III) per milliliter) to be added to 2.5-gallons (9.5 L) liquid bicarbonate concentrate for individual use. Powder packets contain 272 mg of iron (III) per packet and are to be added to 25 gallons (95 L) of liquid bicarbonate in a central feed system. Multiple packets can be used for larger volumes of central feed bicarbonate concentrate. The final dialysate iron concentration is 2 µM (110 µg Fe/L dialysate). Dialysate FPC is added to the bicarbonate concentrate at each hemodialysis session.
The IV formulation of FPC contains 6.75 mg FPC iron in 4.5 mL water for injection. The IV presentation is in low-density polyethylene (LDPE) vented luer-lock ampules to facilitate needle-free removal of the drug to a syringe for infusion during hemodialysis. FPC can be infused IV using an infusion pump to the pre-dialyzer or post-dialyzer bloodline during 3 or 4 h of hemodialysis.
The PK profile of dialysate FPC and intravenous FPC are similar to the PK in healthy volunteers with the only difference being the absence of the diurnal sFe variation. The diurnal variation of sFe is driven by changes in hepcidin in healthy volunteers while the constitutive elevation of hepcidin in HD patients results in a more constant iron concentration. The serum iron concentration-time curves for serum iron and TBI in HD patients are presented in . The approval of the IV formulation was based on demonstrating the equivalence of iron administration by the IV route compared to the dialysate. The equivalence criteria for absolute plasma total iron and TBI are presented in .
Table 1. Summary of results from the relative bioavailability evaluation for absolute total plasma iron and transferrin-bound iron.
The pharmacokinetics and safety of FPC administered via dialysate and IV in pediatric HD patients were investigated in a multicenter, open-label, 2-period, single-dose studyCitation34. FPC (0.07 mg iron/kg) was infused IV into the venous blood return line during hemodialysis. FPC iron was added to bicarbonate concentrate to deliver 2 µM (110 µg/L) iron via dialysate during the second hemodialysis. Mean serum total iron concentrations peaked 3–4 h after administration via dialysate and 2–4 h after IV administration and returned to baseline by 6 h after the end of hemodialysis for both routes. Iron exposure was greater after administration via dialysate than after IV administration. The absolute amount of absorbed iron after administration via dialysate roughly doubled with increasing age, but the weight-normalized amount of absorbed iron was relatively constant across age groups (∼0.06–0.10 mg/kg). FPC was well tolerated in the small number of patients studied. The weight-based dosing algorithm to maintain hemoglobin in pediatric HD patients is currently under investigation.
Clinical studies of FPC in hemodialysis patients
Two Phase 3, multicenter randomized, single-blind (patients blinded to treatment assignment), placebo-controlled, parallel-group, 48-week studies were conducted in a total of 599 iron-replete HDD-CKD patients to evaluate the efficacy and safety of FPC (2 μM; 110 μg Fe/L) delivered via the dialysate during each hemodialysis session (CRUISE [Continuous Replacement Using Iron Soluble Equivalents] 1 and 2)Citation35. An additional Phase 2, multicenter, randomized, double-blind, placebo-controlled, 36-week study (Physiological Replenishment Iron Maintenance Equivalency ′) was conducted in 133 HDD-CKD patients who were undergoing hemodialysis 3–4 times weekly to test the hypothesis that FPC (2 μM, 110 μg Fe/L) delivered via the dialysate during each hemodialysis session could reduce prescribed ESA use and maintain hemoglobin levels in the recommended range in HDD-CKD patients.
FPC added to the dialysate reliably delivers iron as measured by the change from pre-HD to post-HD serum iron concentrations (). In the CRUISE studies, mean intradialytic increases in serum iron were observed in the dialysate FPC treatment group, demonstrating the transfer of FPC-iron from the dialysate to the blood compartment with concurrent increases in TSAT. The primary endpoint for the CRUISE studies was hemoglobin concentration. To establish the effect of FPC to maintain Hgb compared to placebo, the dose of erythropoietic stimulating agents (ESA) needed to remain constant because ESA has an independent effect on Hgb. Because of this constraint, pre-specified safety criteria for study completion were established. The Study design and completion criteria are presented in . The end-of-treatment Hgb was defined as the mean of the Hgb values during the last 1/6th of study participation to show the durability of the results.
Figure 5. Pre- and Post-hemodialysis serum iron and TSAT in the CRUISE studies. FPC administered by dialysate in the CRUISE studies shows an incremental increase of 100 µg/dL with a concomitant TSAT increase of 35%. Placebo-treated patients showed no change in serum iron or TSAT pre- to post-HD.
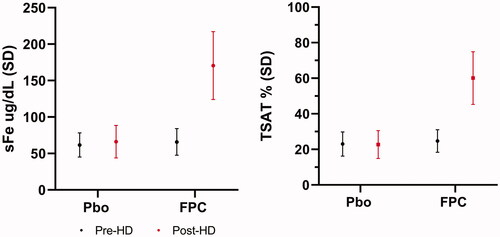
Figure 6. Schematic of the CRUISE study design. Stable iron-replete HD patients were randomized to receive FPC (110 µg Fe/L) or standard dialysate. Once randomized, the dose of ESA could not be changed, and no iron (IV or oral) was allowed. Patients completed the study and were eligible to enter the open-label extension study if pre-specified safety criteria were met. These criteria were: (a) a confirmed Hb <9 or >12 g/dL; (b) a confirmed serum ferritin <100 µg/L or (c) if Hgb was > 11.5 g/dL and associated with a 1 g/dL increase over 4 weeks. The primary endpoint was the Hgb at end of treatment defined as the last one-sixth (1/6th) of time in the randomized treatment phase.
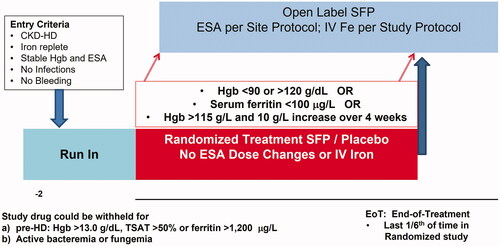
Dialysate FPC was more effective than placebo for maintaining hemoglobin levels in the CRUISE studies. The mean (SD) change in hemoglobin from baseline to end of treatment was −0.03 (1.147) g/dL in the dialysate FPC-treated patients and −0.38 (1.240) g/dL in the placebo-treated patients in the CRUISE 1 study and −0.08 (1.152) g/dL in the dialysate FPC-treated patients and −0.44 (1.157) g/dL in the placebo-treated patients in the CRUISE 2 study (p < .05 for both comparisons). Pooled data from the CRUISE studies demonstrated that dialysate FPC maintained hemoglobin levels throughout the treatment period (), whereas decreases in hemoglobin levels, a consequence of iron-restricted erythropoiesis were observed throughout the treatment period in the placebo-treated patients. A significant treatment difference, favoring dialysate FPC, was observed for the change from baseline in hemoglobin concentration at the end of treatment (least-square mean difference, 0.40 g/dL; p < .001).
Figure 7. Primary and secondary endpoints in the CRUISE study (combined results). In patients receiving FPC, Hgb remained within baseline values for the duration of treatment, while placebo patients Hgb declined due to iron-restricted erythropoiesis. Serum ferritin, an index of iron stores and inflammation, declined in both study arms. Patients receiving a placebo had greater declines in ferritin indicating mobilization of iron stores. The mobilization of iron stores was not sufficient to maintain Hgb without the additional bioavailable iron provided by FPC at each HD treatment.
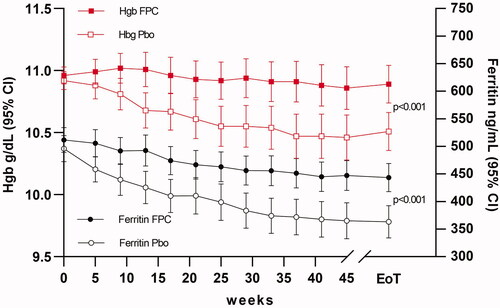
Because patients could complete the study at various times due to protocol-mandated changes in anemia management (PMAM), a number of post-hoc analyses were conducted to demonstrate the robustness of the primary analysis. The forest plot of all pre-specified and post-hoc analyses showed that when missing data was imputed, the effect size was reduced but remained statistically significant compared to placebo to maintain Hgb (). Importantly, the analysis of patients who completed the study due to protocol-mandated changes in anemia management showed a similar overall effect as the primary endpoint analysis confirming the robustness of the results.
Figure 8. Sensitivity Analyses of the primary endpoint for the CRUISE-1 and CRUISE-2 study. FPC demonstrated maintenance of hemoglobin in all populations, subgroups, and sensitivity analyses evaluated, including the ITT population. With the exception of the multiple imputation analysis of the efficacy evaluable population for SFP-4, all analyses showed statistically significant effects favoring FPC. The post hoc analysis of patients who completed the study due to protocol-mandated changes in anemia management showed a similar mean difference when compared with placebo.
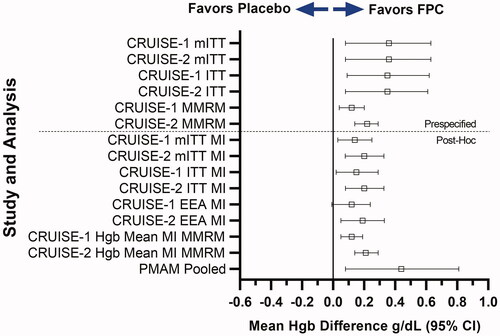
The Kidney Disease Improving Global Outcomes (KDIGO) recommends serum ferritin (a marker of iron stores) and TSAT (a marker of iron availability) as noninvasive markers of iron balance in HDD-CKD patientsCitation3. In the CRUISE studies, the placebo group showed a decline in ferritin of −133.1 ± 231.4 μg/L, while the FPC-treated patients had only a small decline of −69.7 ± 149.3 μg/L. Transferrin saturation from baseline to end of treatment was −1.0% (8.4%) in FPC-treated patients and −3.2% (7.8%) in the placebo group. Ferritin is a marker of iron stores and inflammation, while placebo patients could mobilize stored iron, the amount mobilized was not sufficient to maintain Hgb concentrations.
The objective of the PRIME study was to assess if FPC could improve the efficiency of erythropoiesis and reduce the ESA dose. Patients were randomized to receive FPC via dialysate at each treatment or placebo for 36 weeks. The study was double blinded by providing liquid bicarbonate containers containing FPC or standard bicarbonate concentrate. Stable, iron-replete HD patients were enrolled. During the first 4 weeks, no IV iron was allowed, and the ESA dose was to remain stable (). Subsequently, the ESA dose was adjusted by a blinded Anemia Management Group based on changes in Hgb concentration obtained bi-weekly. IV iron was administered when patients met pre-specified criteria for iron deficiency (ferritin <200 µg/L). The primary endpoint was the percent difference in ESA dose at end-of-treatment (EoT) between the FPC and placebo group.
Figure 9. Schematic of the PRIME study design. The PRIME study was a randomized, double-blind, placebo-controlled study comparing patients receiving FPC to placebo over 36 weeks. Iron-replete stable HD patients were randomized to receive FPC (110 µg Fe/L) or standard dialysate. ESA dose was facilitated by an external blinded anemia management group based on weekly changes in Hgb. Iron repletion was allowed in both study arms if patients met the criteria for iron-deficiency anemia (ferritin <200 µg/L). The primary endpoint was the percent change from baseline in ESA dose at End-of-Treatment (EoT: last 2 weeks on randomized treatment).
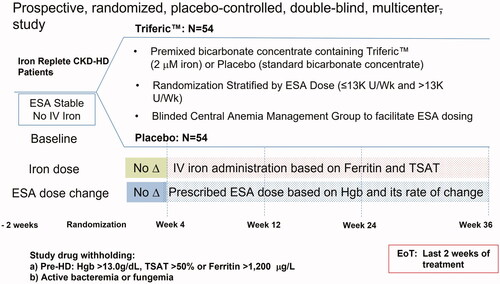
Patients in both study arms maintained Hgb within the specified range (9.5–11.5 g/dL). Patients in the Placebo arm required an increasing ESA dose to maintain Hgb concentration while patients receiving FPC at each HD treatment showed no change from baseline. At the end of treatment (EoT), the ESA dose was not significantly changed from baseline in the FPC group (+4.9%; 95% CI, −19.1, 28.8) but had increased by 39.8% (95% CI, 15.7, 64.0) from baseline in the placebo group (). The least squares mean difference (FPC–placebo) in the prescribed ESA dose was 35% (95% CI, −69.1, 0.8; p = .045). Fewer FPC-treated patients (11/52, 21.2%) than placebo-treated patients (20/51, 39.2%) required IV iron supplementation. Overall, the FPC group required 51% less IV iron supplementation than placebo patients. Serum ferritin concentration declined in the placebo patients compared to FPC treated patients. Markers of inflammation, oxidative stress and hepcidin concentrations in the FPC patients were not different from the placeboCitation36.
Figure 10. The primary endpoint in the PRIME study. The primary endpoint was the % change from baseline in ESA dose at EoT. Hgb concentration was maintained in both treatment arms. The primary endpoint was achieved with an ESA dose reduction of 35% (95% CI −69.1, −0.8) compared to a placebo. The absolute ESA change from the baseline dose in the FPC arm was 388 ± 5556 U/week. The placebo arm change from baseline was 3423 ± 11,642 U/week for a difference of 3035 U/week increase in placebo compared to FPC.
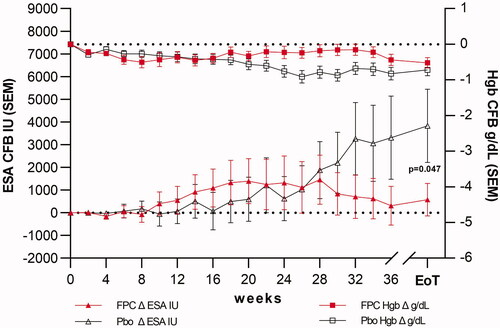
Intravenous FPC for use with solid bicarbonate delivery systems
There is increasing use of solid bicarbonate to generate liquid bicarbonate online for individual dialysis patients. FPC cannot be added to the solid bicarbonate, so an intravenous formulation was developed. The IV formulation delivers an equivalent amount of iron as does the dialysate. The equivalence study was a randomized 3-period crossover study to show that the IV formulation (6.75 mg Fe/4.5 mL) delivered over 3 h into the pre- or post-dialyzer bloodlines provided equivalent iron as when administered via dialysateCitation37. The delivery of FPC iron IV was the same as observed in the CRUISE and PRIME studies as measured by the pre-HD to post-HD serum iron concentrations ().
Figure 11. Equivalence of iron delivery from dialysate and IV. The change from pre-HD to post-HD serum iron concentrations is proportional to the amount of iron delivered. In the CRUISE-1 and CRUSIE-2 studies, FPC was delivered exclusively by dialysate. In the clinical pharmacology equivalence study, FPC was delivered by dialysate and IV pre-dialyzer and post-dialyzer. The pre-HD to post-HD serum iron concentrations were the same indicating the equivalence of iron delivery. This confirms the average iron delivery by dialysate was 6.5 mg at each HD treatment.
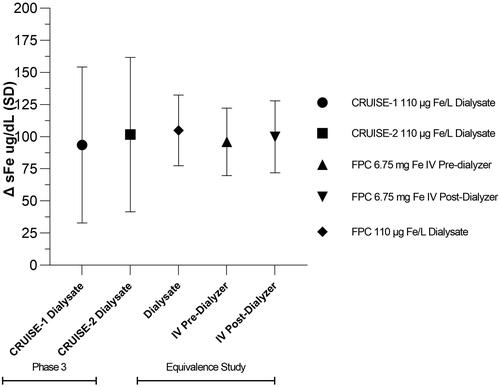
During the development program for FPC IV, the IV infusion was provided by the on-machine syringe pump used to administer heparin. Continuous heparin for anticoagulation is still used in some patients to maintain dialyzer patency. To establish the safety and pharmacodynamics of a mixture of unfractionated heparin (UFH) and IV FPC, a drug-interaction study was conducted both in-vitro and in-vivoCitation38. FPC-IV ampules for single use are designed for needle-free removal of the drug contents. For this study, the patient’s usual heparin dose was drawn into the syringe from the multi-dose UFH vial with a needle and the volume was noted. The needle was removed, and the syringe was attached to the FPC ampule using the luer adapter and the 4.5 mL of FPC was withdrawn. The syringe was inverted to mix the contents and the total volume was noted. The syringe was attached to the pre-dialyzer bloodlines using the existing heparin connection. The syringe pump was programmed to deliver the entire contents of the syringe over 3 h. FPC did not change the pharmacodynamics of UFH as measured by Anti-Xa activity and aPTT whether administered separately or together with FPC in the same syringe. UFH did not change iron delivery when admixed with FPC ().
Figure 12. No drug-drug interaction between FPC and unfractionated heparin (UFH). FPC and UFH mixed together and infused pre-dialyzer have the same effect as if administered in separate sites along the dialyzer blood circuit. Anti-Xa activity and aPTT were not different when administered during the first 3 h of HD. Anti-Xa activity and aPTT returned to baseline levels within an hour after stopping the heparin infusion with or without FPC.
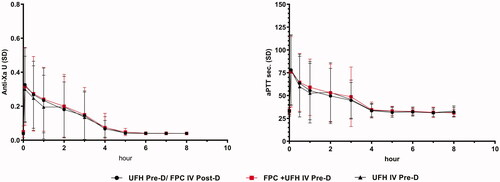
The safety profile of dialysate FPC is comparable to placebo
The clinical development program for FPC consists of 4 placebo-controlled studies in hemodialysis patients; Two (2) Single-blind studies of up to 48 weeks (CRUISE-1 and CRUISE-2); One (1) Double-blind Placebo-controlled study of 9 months; One (1) short term double-blind crossover study of 2 weeks and, 3 open-label extension studies of up to 52 weeks additional exposure to FPC. A total of 1411 patients were exposed to FPC in controlled, open-label and clinical pharmacology studies. Safety in all studies was assessed by collection of adverse events (AE), serious adverse events (SAE), primarily hospitalizations, laboratory assessments and vital sign data.
The safety analysis examined the incidence of AE and AE of special interest. Exposure-adjusted event rates and incidences of adverse events were also calculated because patients were in the studies for varying times up to 18 months depending on the study.
The patient populations enrolled in the controlled FPC studies were 58.5 ± 13.1 years of age, with 32.0% > 65 years; Females were 36.7% and 44.5% were non-white. In the uncontrolled extension and safety studies, the age was 58.8 ± 13.3 years with 31.5% >65 years; Females were 38.3% and 49.2% were non-white.
In the 48-week CRUISE studies, the safety profile of dialysate FPC was comparable to that of placeboCitation35. At least one treatment emergent AE was reported in 78.4% of dialysate FPC-treated patients and in 75.3% of placebo-treated patients. One SAE was reported in 27.7% of FPC patients and 27.4% of placebo-treated patients. Only 2.4% of the dialysate FPC-treated patients discontinued treatment because of drug-related TEAEs. No treatment-related deaths were reported.
In the 36-week double-blind, PRIME studyCitation36, comparable percentages of dialysate FPC-treated patients and placebo-treated patients experienced at least one TEAE (7.4% vs 6.1%) and at least one SAE (33.3% vs 40.8%). More dialysate FPC-treated patients than placebo-treated patients discontinued treatment due to TEAEs (7.4% vs 2.0%); however, none of the events that led to discontinuation of dialysate FPC were assessed by investigators as related to the drug. No treatment-related SAEs or deaths were reported.
The most common adverse events in controlled and uncontrolled clinical trials are presented in . Adverse reactions in patients receiving FPC were similar to patients receiving Placebo. The most common adverse reactions leading to treatment discontinuation in both groups included headache, asthenia, dizziness, constipation, nausea, intradialytic hypotension, pruritis pyrexia and hypersensitivity reactions.
Table 2. Adverse events in controlled and All FPC studies.
Adverse events of special interest concerning iron administration were analyzed in all studies. Procedural hypotension was the most common event occurring at similar rates across all analyses, followed by av-fistula/graft complications, headache, nausea, dizziness and hemodialysis-induced complications. There were small numerical differences in the numbers of patients and events observed for AVF thrombosis. The number of events per patient was the same between FPC (1.25/pt) and Placebo (1.25/pt) as well as in the long-term uncontrolled studies (1.29/pt). Post-marketing surveillance confirms the lack of reported AVF thrombosis events. All these events commonly occur in hemodialysis procedures.
Treatment with dialysate FPC was not associated with increased levels of inflammation (as assessed by C-reactive protein or IL-6) or oxidative stress (as assessed by malondialdehyde F2 isoprostanes and isofurans)Citation36. Treatment with dialysate FPC did not induce significant increases in hepcidin levels, indicating the absence of hepcidin induced sequestration. The controlled FPC studies followed patients for 36–48 weeks. Serum ferritin, an index of iron overload and inflammation declined over time in both treatment groups. The lack of a ferritin increase with the administration of iron at each treatment supported the conclusion that FPC does not contribute to iron overload.
A non-serious hypersensitivity reaction was reported in 1 (0.3%) of 292 patients receiving FPC in the two randomized CRUISE trials. Further review of this case concluded that this may have been an idiosyncratic infusion reaction. The event started within 5 min of starting the first dialysis with FPC. The event was characterized by flushing and facial tingling. There was no hypotension or tachycardia. FPC was stopped, the patient received diphenhydramine and dialysis resumed using standard dialysate. No rechallenge was performed and the patient subsequently refused further FPC therapy. In the large safety study (NCT01503021), no adverse events meeting the criteria for anaphylaxis or hypersensitivity were reported among 703 patients who received dialysate FPC during a 5-week randomized, double-blind, crossover study or among the 309 patients who received dialysate FPC during the subsequent 52 weeks open-label treatment period. No cases of anaphylaxis or hypersensitivity were reported among the 54 patients who received dialysate FPC for up to 36 weeks in the PRIME study. A second case, while classified by the investigator as hypersensitivity to study drug, did not meet any criteria for a possible hypersensitivity reaction. Two events were reported within this AE, first was mild flushing lasting a few seconds and resolving with no treatment while continuing to receive FPC. The second was an event of volume depletion. The patient started dialysis below his dry body weight and experienced hypotension approximately 2.5 h into the treatment. The patient responded to IV saline and completed the dialysis while still receiving FPC. The patient received the full dose of FPC via dialysate during HD and only required IV saline. None of the events met the criteria for Serious and no specific treatments were required. Other adverse events in the clinical studies with FPC had verbatim terms such as allergy or reaction to, but these events occurred in the interdialytic interval, were attributed to other drugs, and were not temporally associated with dialysate FPC administration.
Discussion
Iron replacement therapy is needed to manage anemia chronic hemodialysis patients. Ferric pyrophosphate citrate is a novel iron replacement product developed to maintain iron balance and hemoglobin for adult chronic hemodialysis patients. FPC has a mechanism of action unlike that of other macromolecular IV iron products. FPC donates iron directly to transferrin for transport to iron-requiring tissues, primarily the bone marrow. Upon donation of iron, the pyrophosphate and citrate are added to the plasma in physiologic quantities and the iron is rapidly cleared from the circulation. FPC is not sequestered in the macrophages of the reticuloendothelial system. Hepcidin, elevated in chronic hemodialysis patients and known to block iron egress from macrophages, does not decrease iron availability from FPC.
FPC can be added to the dialysate solution for administration to patients by transfer across the dialyzer membrane or administered directly intravenously. The pharmacokinetics of FPC have been well characterized. FPC shows dose proportional kinetics up to maximum iron binding capacity (TSAT). Plasma clearance is rapid with a t½ of 1.4–2.0 hr. in both healthy volunteers and HD patients. PK of dialysate and IV delivered FPC are the same. Dialysate FPC delivers approximately 6.5 mg of iron with each 4-hour treatment. This amount of iron matches the estimated ongoing iron losses of HDCitation1,Citation2. No dose adjustment for body weight, dialyzer membrane, blood flow rate (BFR) or dialysate flow rate (DFR) is required. Pharmacokinetics of dialysate FPC in pediatric HD patients shows dependence on dialysate flow rates and blood flow rate as well as on dialyzer membrane surface areaCitation34. The single-dose pediatric study suggests that FPC IV at a dose of 0.1 mg Fe/kg should be an adequate dose to maintain hemoglobin. This hypothesis is currently being studied in a clinical trial (NCT 04239391).
Clinical studies have demonstrated that administration of FPC at each hemodialysis session is well tolerated, provides reliable delivery of iron, and effectively maintains hemoglobin levels and iron balance. FPC is well tolerated with an adverse event profile similar to patients receiving a placebo. FPC is not associated with an increased risk of infection or inflammation and is not contraindicated for use in any patient population. Evidence from observational studies suggests that FPC administered with each hemodialysis treatment may reduce IV iron, lower doses of ESA and show pharmacoeconomic benefitsCitation39,Citation40. It is estimated that FPC can reduce anemia management costs by approximately $1000/pt/year based on lower ESA and conventional IV iron costs. This estimate is based on the publicly available cost for ESA and IV iron and may not be representative of savings for any individual HD unitCitation39. Because FPC improves the efficiency of erythropoiesis, it may also improve anemia management in ESA hyporesponsive patientsCitation36.
All parenteral iron products including FPC contain a warning in the approved labeling for hypersensitivity reactions. Hypersensitivity reactions to iron are poorly characterized. The spectrum of reactions ranges from infusion reactions characterized by flushing, headache, nausea, pruritis, metallic taste, etc. These reactions are usually self-limited, resolve shortly after the infusion is stopped and do not usually recur upon re-exposureCitation41,Citation42. Serious life-threatening anaphylaxis is rare (20–29/100,000 patients) and most likely to occur on the first doseCitation43,Citation44. Another category of hypersensitivity is the pseudo-allergic reaction. Pseudo-allergy is the activation of inflammatory mechanisms independent of antigen specificity. Three types of agents have been implicated in this response: (1) direct mast cell activators; (2) complement activators (complement-mediated pseudo-allergy-CARPA); and (3) non-steroidal anti-inflammatory agentsCitation45–47. Of the 7 FDA-approved iron products, 6 are macromolecular nanoparticulate compounds with an iron hydroxide gel core surrounded by a carbohydrate (sucrose, gluconate…etc.) shell. The particle size of the nanoparticles ranges from 8 to 20 microns. The nanoparticle formulation may contribute to the reported adverse reactions with traditional IONP products. FPC is a different molecule from these iron products, being a true solution devoid of any particulate material. The FPC development program administered FPC iron for up to 18 months of exposure. Post-marketing FPC exposure is greater than 1,500,000 individual doses. The single non-serious hypersensitivity reaction in the approved product labeling was characterized by transient flushing and anxiety on the first administration of FPC starting within minutes of dialysis. The FPC administration was stopped, and dialysis was continued without FPC. There was no hypotension, back pain, nausea or vomiting. The patient received 25 mg diphenhydramine orally but completed the entire HD session. This reaction was characterized as a possible hypersensitivity reaction due to the temporal relationship with FPC administration but did not meet any criteria for “anaphylaxis.” There have been no reports of delayed “hypersensitivity type” reactions in the clinical studies or post-marketing exposure.
The safety profile of FPC is very similar to the background events common in HD patients. Dialysate administration of FPC may have an additional benefit of reducing staff contact with patients, an important preventative measure during the COVID pandemic. The IV presentation of FPC is designed for easy removal of drug from the ampule without the need for needles. FPC can be safely admixed with unfractionated heparin for administration via the on-machine syringe pump or by an independent infusion pump.
The FPC studies provide long-term safety and efficacy information about iron replacement. Because FPC is a maintenance iron replacement therapy all patients enrolled were iron replete and on stable doses of ESA. The limitation of the studies was that incident dialysis patients were excluded. The FPC study population was different from the PIVOTAL trial where patients were new to dialysis and iron deficientCitation48. In PIVOTAL, patients in the repletion arm achieved Hgb targets faster than in the reactive arm where iron deficiency persisted longer. HD patients should have iron deficiency corrected as a priority, but the long-term use of high-dose macromolecular iron repletion is currently used to drive ferritin to >800 mcg/L requires further study.
In summary, FPC represents a novel physiologic iron replacement product for the long-term management of hemoglobin levels and iron balance in patients with HDD-CKD. The low doses of iron administered at each HD provide a well-tolerated and effective means to maintain iron balance. Traditional IV iron products may still be needed to replete IV iron stores in patients experiencing excessive blood or tissue losses leading to iron deficient anemia.
All clinical trials referenced have been registered. NCT registration numbers are provided as a Supplemental table (S-1).
Transparency
Declaration of funding
This work was funded by Rockwell Medical, Inc (Wixom, MI, USA).
Declaration of financial/other relationships
SF and TG are consultants for Rockwell Medical. SF has done research and consulted for Astra Zeneca, Fibrogen, Akebia. TG is the scientific co-founder of Intrinsic LifeSciences and Silarus Pharma and has consulted for ADARx, Akebia, Pharmacosmos, Ionis, Gossamer Bio, Global Blood Therapeutics, American Regent, and Disc Medicine. RDP was an employee of Rockwell Medical Inc. and received salary, stock grants and options. Peer reviewers on this manuscript have no relevant financial or other relationships to disclose.
Author contributions
RDP prepared the manuscript. All authors reviewed and edited subsequent and final versions of the manuscript.
Clinical_Trial_Registrations_S-1.docx
Download MS Word (16.1 KB)Acknowledgements
The authors thank Dr. Ajay Gupta, who re-discovered FPC, contributed to the development of the product and provided a review of the manuscript concept; Dr. Allen Nissenson for his review and conceptual comments on this manuscript. All authors were responsible for and approved the content of the manuscript.
References
- Sargent JA, Acchiardo SR. Iron requirements in hemodialysis. Blood Purif. 2004; 200422(1):112–123.
- Babitt JL, Lin HY. Mechanisms of anemia in CKD. J Am Soc Nephrol. 2012;23(10):1631–1634.
- KDIGO. KDIGO clinical practice guideline for anemia in chronic kidney disease. Kidney Int Suppl. 2012;2(4):279–334.
- Wang C, Graham DJ, Kane RC, et al. Comparative risk of anaphylactic reactions associated with intravenous iron products. JAMA. 2015;314(19):2062–2068.
- Rostoker G, Vaziri ND, Fishbane S. Iatrogenic iron overload in dialysis patients at the beginning of the 21st century. Drugs. 2016;76(7):741–757.
- Rostoker G, Vaziri ND. Impact of iatrogenic iron overload on the course of hepatitis C in the dialysis population: a plea for caution. Hemodial Int. 2017;21 (Suppl 1):S68–S77.
- Ganz T. Systemic iron homeostasis. Physiol Rev. 2013;93(4):1721–1741.
- Paganini EP. Overview of anemia associated with chronic renal disease: primary and secondary mechanisms. Semin Nephrol. 1989;9(1 Suppl 1):3–8.
- Wizemann V, Buddensiek P, de Boor J, et al. Gastrointestinal blood loss in patients undergoing maintenance dialysis. Kidney Int Suppl. 1983;16:S218–S20.
- Rosenblatt SG, Drake S, Fadem S, et al. Gastrointestinal blood loss in patients with chronic renal failure. Am J Kidney Dis. 1982;1(4):232–236.
- Noris M, Remuzzi G. Uremic bleeding: closing the circle after 30 years of controversies? Blood. 1999;94(8):2569–2574.
- Tsukamoto T, Matsubara T, Akashi Y, et al. Annual iron loss associated with hemodialysis. Am J Nephrol. 2016;43(1):32–38.
- Ganz T. Erythropoietic regulators of iron metabolism. Free Radic Biol Med. 2019;133:69–74.
- Ganz T, Nemeth E. Iron balance and the role of hepcidin in chronic kidney disease. Semin Nephrol. 2016;36(2):87–93.
- Brătescu LO, Bârsan L, Gârneaţă L, et al. Effects of additional iron doses on hepcidin-25 level in hemodialysis patients without evident iron deficiency. Int Urol Nephrol. 2014;46(5):1005–1012.
- Fishbane S, Spinowitz B. Update on anemia in ESRD and earlier stages of CKD: core curriculum 2018. Am J Kidney Dis. 2018;71(3):423–435.
- Shirazian S, Grant C, Miller I, et al. How can erythropoeitin-stimulating agent use be reduced in chronic dialysis patients? The use of iron supplementation to reduce ESA dosing in hemodialysis. Semin Dial. 2013;26(5):534–536.
- Grutzmacher P, Tsobanelis T, Roth P, et al. Effect of recombinant human erythropoietin on iron balance in maintenance hemodialysis: theoretical considerations, clinical experience and consequences. Clin Nephrol. 1992;38 (Suppl 1):S92–S97.
- Plastina JCR, Obara VY, Barbosa DS, et al. Functional iron deficiency in patients on hemodialysis: prevalence, nutritional assessment, and biomarkers of oxidative stress and inflammation. Braz J Nephrol. 2019;41(4):472–480.
- Goodnough LT. Iron deficiency syndromes and iron-restricted erythropoiesis (CME). Transfusion. 2012;52(7):1584–1592.
- Health ARCf. DOPPS practice monitor; 2020. Available from: https://www.dopps.org/DPM/Default.aspx.
- Rostoker G, Vaziri ND. Iatrogenic iron overload and its potential consequences in patients on hemodialysis. Presse Med. 2017;46(12 Pt 2):e312–e28.
- Ashby DR, Gale DP, Busbridge M, et al. Plasma hepcidin levels are elevated but responsive to erythropoietin therapy in renal disease. Kidney Int. 2009;75(9):976–981.
- Robiquet ME. Therapeutic employment of the pyrophosphate of iron. Am J Med Sci. 1857;34:215–217.
- Chapman EM. The history, preparation and theraeutical uses of the citro-ammoniacal pyrophosphate of iron, named in brief pyrophosphate of iron. Boston Med Surg J. 1862;66(1):9–12.
- Gupta A, Amin NB, Besarab A, et al. Dialysate iron therapy: infusion of soluble ferric pyrophosphate via the dialysate during hemodialysis. Kidney Int. 1999;55(5):1891–1898.
- Gupta A, Pratt R, Mishra B. Physicochemical characterization of ferric pyrophosphate citrate. Biometals. 2018;31(6):1091–1099.
- Gupta A, Crumbliss AL. Treatment of iron deficiency anemia: are monomeric iron compounds suitable for parenteral administration? J Lab Clin Med. 2000;136(5):371–378.
- Pratt R, Handelman GJ, Edwards TE, et al. Ferric pyrophosphate citrate: interactions with transferrin. Biometals. 2018;31(6):1081–1089.
- Edwards TE, Pratt R. Crystal structure of human transferrin bound to Triferic FPC iron pyrophosphate; 2018. Available from: https://www.rcsb.org/structure/6CTC.
- Noinaj N, Steere A, Mason AB, et al. The 2.1 angstrom crystal structure of diferric human transferrin; 2012. Available from: https://www.rcsb.org/structure/3V83.
- Pratt RD, Swinkels DW, Ikizler TA, et al. Pharmacokinetics of ferric pyrophosphate citrate, a novel iron salt, administered intravenously to healthy volunteers. J Clin Pharmacol. 2017;57(3):312–320.
- Brissot P, Ropert M, Le LC, et al. Non-transferrin bound iron: a key role in iron overload and iron toxicity. Biochim Biophys Acta. 2012;1820(3):403–410.
- Pratt RD, Grimberg S, Zaritsky JJ, et al. Pharmacokinetics of ferric pyrophosphate citrate administered via dialysate and intravenously to pediatric patients on chronic hemodialysis. Pediatr Nephrol. 2018;33(11):2151–2159.
- Fishbane SN, Singh AK, Cournoyer SH, et al. Ferric pyrophosphate citrate (triferic) administration via the dialysate maintains hemoglobin and iron balance in chronic hemodialysis patients. Nephrol Dial Transplant. 2015;30(12):2019–2026.
- Gupta A, Lin V, Guss C, et al. Ferric pyrophosphate citrate administered via dialysate reduces erythropoiesis-stimulating agent use and maintains hemoglobin in hemodialysis patients. Kidney Int. 2015;88(5):1187–1194.
- Marbury T, van Heuveln F, van der Horst E, et al. Pharmacokinetics and safety of ferric pyrophosphate citrate intravenous: Equivalence to administration via dialysate. J Clin Pharmacol. 2021;62(5):681–688.
- Pratt RD. Pharmacokinetics and pharmacodynamics of unfractionated heparin and FErric pyrophosphate citrate co-administration during hemodialysis: no drug-drug interaction. J Bioequivalence Bioavailab. 2021;13(S4):1–7.
- Hoffman M, Delvalle R, Pratt RD. Retrospective observational analysis of ferric pyrophosphate citrate (Triferic®) administered via dialysate. Experience at a Single Facility over 2 Years Archives of Clinical Nephrology. 2021;7(1):044–049.
- Shan Wang LD, Dhanani L, Malone B, et al. Institutional usage of ferric pyrophosphate citrate (FPC) delivered via dialysate in reducing erythropoiesis stimulating agents (ESAs) and IV iron cost. Hosp Pharm. 2022;57(3):355–358.
- Szebeni J, Fishbane S, Hedenus M, et al. Hypersensitivity to intravenous iron: classification, terminology, mechanisms and management. Br J Pharmacol. 2015;172(21):5025–5036.
- Rampton D, Folkersen J, Fishbane S, et al. Hypersensitivity reactions to intravenous iron: guidance for risk minimization and management. Haematologica. 2014;99(11):1671–1676.
- Wang C, Wong S, Graham DJ. Risk of anaphylaxis with intravenous iron products. JAMA. 2016;315(20):2232–2233.
- Dave CV, Brittenham GM, Carson JL, et al. Risks for anaphylaxis with intravenous iron formulations: a retrospective cohort study. Ann Intern Med. 2022;175(5):656–664.
- Wang H, Wang HS, Liu ZP. Agents that induce pseudo-allergic reaction. Drug Discov Ther. 2011;5(5):211–219.
- Moghimi SM. Nanomedicine safety in preclinical and clinical development: focus on idiosyncratic injection/infusion reactions. Drug Discov Today. 2018;23(5):1034–1042.
- Szebeni J. Complement activation-related pseudoallergy: a stress reaction in blood triggered by nanomedicines and biologicals. Mol Immunol. 2014;61(2):163–173.
- Macdougall IC, White C, Anker SD, et al. Intravenous iron in patients undergoing maintenance hemodialysis. N Engl J Med. 2019;380(5):447–458.