ABSTRACT
A major challenge in controlling overabundant wildlife is monitoring their populations, particularly as they decline to very low density. Camera traps and wildlife detector dogs are increasingly being used for this purpose. We compared the cost-effectiveness of these two approaches for detecting feral cats (Felis catus) on two pastoral properties in Hawke's Bay, North Island, New Zealand. One property was subject to intensive pest removal, while the other had no recent history of pest control. Camera traps and wildlife detector dogs detected cats at similar rates at both sites. The operating costs of each method were also comparable. We identify a number of advantages and disadvantages of each technique, and suggest priorities for further research.
Introduction
Invasive predators are one of the greatest threats to global biodiversity, and their impacts in Australia and New Zealand have been catastrophic (Salo et al. Citation2007; Simberloff Citation2010). Control of invasive predators is often hindered by the difficulty of detecting them in the field; often they are cryptic, highly mobile, and occur at low density (Witmer Citation2005; Marks et al. Citation2009; Clayton & Cowan Citation2010). However, even very low densities of invasive predators can threaten populations of vulnerable native species (e.g. Innes et al. Citation2010). Camera trapping is an increasingly popular technique for monitoring cryptic carnivores (e.g. Karanth Citation1995; Lyra-Jorge et al. Citation2008; Bengsen et al. Citation2011a, Citation2011b; Brook et al. Citation2012; Meek et al. Citation2014). Wildlife detector dogs are also highly effective at communicating the presence of carnivores and/or their scats (Long et al. Citation2007a, Citation2007b; Recio et al. Citation2010; Brown et al. Citation2015).
Feral cats (Felis catus) have become widely established around the globe (Nogales et al. Citation2004; Campbell et al. Citation2011; Abbott et al. Citation2014), causing decline and extinction of native species, as well as detrimental impacts on humans and livestock (Medina et al. Citation2011, Citation2014; Glen et al. Citation2013a). To protect native prey species, cats are subject to lethal control in parts of Australia and New Zealand (Algar et al. Citation2007; Moseby & Hill Citation2011; Reardon et al. Citation2012). The effectiveness of control is often judged by numbers of animals removed, which provides no information on the numbers that remain (Glen et al. Citation2014), or by indirect measures of activity such as spoor or spotlight counts, which can suffer from low precision (Cruz et al. Citation2013). An affordable, accurate and precise monitoring method would better allow managers to decide where and when additional effort is needed to control feral cats. The ability to detect feral cats at very low density is also important in eradication campaigns (e.g. Campbell et al. Citation2011; Ramsey et al. Citation2011), when every last animal must be detected and/or removed. We conducted a field trial comparing the effectiveness of camera traps and wildlife detector dogs in two areas, one of which had been subject to intensive cat control. We aimed to determine: (1) whether each method could detect cats at relatively high and low abundances; and (2) which method was more cost-effective for detecting cats.
Methods
Study sites
We monitored feral cats on two pastoral properties – Waitere and Toronui stations – in Hawke's Bay, North Island, New Zealand. Both properties are predominantly covered by introduced pasture with remnants of native vegetation. The farm landscapes include many steep gullies, which often contain thick scrub.
Waitere Station had been subject to trapping and removal of feral cats and ferrets (Mustela furo) for 3 weeks immediately before our trial commenced. A combination of leg-hold, cage and kill traps were placed throughout the property, checked daily, and captured cats and ferrets were removed. The results of the predator control will be reported in detail elsewhere; however, detections of feral cats with camera traps fell by 90% following control, suggesting that most resident cats had been removed. Toronui Station had no recent predator control. We therefore classify Toronui Station as the high-density site and Waitere as the low-density site.
Predator monitoring
Predators were monitored using 80 camera traps for 3 weeks in May–June 2014, giving a total of 1680 camera trap-nights. The length of deployment was comparable to other camera trap surveys for feral cats (e.g. Robley et al. Citation2010). At each site, 40 camera traps (Reconyx Hyperfire PC900, Reconyx Inc.) were placed at approximately 500-m intervals, in a grid formation covering a total area of c. 600 ha. A high density of cameras was required for spatially explicit capture–recapture analyses as part of a parallel study. Cameras were mounted on wooden stakes with the base of the camera 10 cm above the ground. A perforated vial containing a scent lure (fresh rabbit meat) was pegged to the ground 1.5 m in front of each camera. The lure was intended to increase the likelihood of predators encountering cameras, and to encourage predators to pause in the camera's field of view so that they could be clearly photographed (Glen et al. Citation2013b). Because the dog survey was conducted concurrently with the camera trapping (see below), the lures were in place for both methods, and did not bias our comparison. Cameras were set to take three photographs each time they were triggered, with no time delay between successive triggers.
Beginning on 6 June, as the camera traps were being removed, we surveyed each site using a detector dog team comprising a handler and two cat-detector dogs, which had been working with the handler for 5–7 years (Conservation Dog Team, New Zealand Department of Conservation (DOC)).
In consultation with the dog handler we evaluated three possible approaches for comparing the cost-effectiveness of the camera traps and the dog team for detecting cats: (1) search within a 100-m radius of each camera trap using dogs, travelling by vehicle between search areas; (2) search along the rows of camera traps on foot, briefly circling each camera trap; (3) divide the camera trap grid into ‘search cells’, each containing four or more cameras, then search each cell using the dog handler's judgement to determine the optimal search pattern.
The first method was deemed unsuitable because the dogs were likely to be less effective if deployed for a series of short searches punctuated by vehicle travel; dogs cannot readily be ‘switched on and off’. The second method was briefly trialled but discontinued because the dog handler judged that the fixed search pattern imposed by the grid caused likely areas of cat habitat (e.g. densely vegetated gullies) to be left unsearched. The third method was a compromise between the need to search a comparable area to that sampled by the camera traps, while operating within the practical constraints of a dog team working on foot.
Each time the dog team was deployed we used a handheld GPS to record start and finish time, path walked by the dog handler, and time and location at which the dogs detected the scent of a cat. The handler judged when cat scent had been detected based on a marked alteration in the dogs’ search pattern. It was beyond the scope of this study to estimate the false-positive detection rate, therefore we assumed perfect specificity (i.e. a dog would not detect cat signs if none were present). Ideally, the accuracy of the dog team would have been tested by genetic analysis of scats (e.g. Brown et al. Citation2011). However, we did not collect scat samples as our limited budget did not cover genetic analyses. The dog team continued to search a cell until either: (1) cat scent was detected, or (2) the handler judged that all likely cat habitat had been searched. We define this as a ‘search event’. As our aim was simply to establish whether cats were present in a given search cell, continuing to search in a cell after cat scent had been detected would not have yielded any additional information. For cameras, we defined a search event as a 3-week deployment period. For each search cell we recorded whether cats had been detected by the dogs, and/or by any of the cameras in the search cell.
Detection probabilities
We estimated the probability of detection of cats per search event using cameras and dogs (Long et al. Citation2007a). The detection probability of a device was conditioned on the cat population density in the area. Although the study was conducted in two areas with assumed different cat densities, both the camera and dog trials occurred under identical conditions, therefore the estimated detection probabilities were directly comparable. We used Bayesian logic and a β-binomial model (Gelman et al. Citation2004) to estimate the detection probability (and associated uncertainty) per search event for each method. This approach estimates distributions of detection probabilities (posteriors), avoids single-number parameter estimates (i.e. explicitly incorporates uncertainty; Clark Citation2005), and allows for direct comparison of methods. The approach begins with initial distributions of detection probabilities (β priors) for both methods that are based on previous studies or expert opinion (Gelman et al. Citation2004). The data collected in the study were then used to update the prior distributions using a binomial likelihood (hence β-binomial). In this study we used uninformed priors, (i.e. any probability between 0 and 1 was equally likely), because few previous estimates were available on detection probabilities of cats using camera traps (but see Robley et al. Citation2008; Robley et al. Citation2010; Ramsey et al. Citation2011).
We conducted a power analysis to determine the number of trials required to detect a range of differences in the probability of detection using detector dogs and camera traps. A sample size was deemed sufficient with power > 0.80 . In this analysis the probability of detection is explicitly defined as the probability of detecting a single individual cat, given that it is present in the searched cell.
Cost estimates
We estimated the operating cost of camera trapping by recording the amount of staff time required (including time taken to review camera footage), and all associated costs such as vehicles and consumables. Costs for dogs were estimated based on daily hire rates provided by the DOC Conservation Dog Team. We compared the cost-effectiveness of camera traps and detection dogs in terms of: (1) the cost of surveying both study sites with each method, and (2) the number of search cells in which cats were detected.
Results
The camera traps and dog team detected cats at both the high-density and the low-density sites; however, poor weather forced the trial to stop before the dog team had searched both sites completely. Our comparison is therefore limited to nine search cells (five on Waitere, four on Toronui), which encompassed 41 of the 80 camera traps.
Camera traps detected cats in four of the nine search cells, whereas the dog team detected cats in five cells. There were two search cells in which cats were detected by both methods (). Where cats were found, mean time to detection by the dogs was 16 minutes (range 13–25 minutes). In cells where cat scent was not detected, the dog team searched for an average of 109 minutes (range 76–140 minutes). On two occasions the dogs flushed a cat, providing visual confirmation that they had correctly identified the scent. We are therefore confident of the dog team's accuracy in identifying cat scent.
Table 1. Detections of cats (Felis catus) by camera traps and wildlife detector dogs deployed for one search event (see text for definition) in each of nine search cells on Waitere Station (low predator density) and Toronui Station (high predator density), Hawke's Bay, New Zealand.
Detection probabilities
The posterior mean probability of detecting cats was 0.45 per search event for cameras and 0.54 per search event for dogs. The distributions of the two posterior means overlapped extensively (), indicating no significant difference in detection probability between the two methods.
Figure 1. Estimated probability of detection for cats (Felis catus) per search event was similar using camera traps (0.45) or wildlife detector dogs (0.54). Extensive overlap between the posterior distributions indicates no significant difference in probability of detection between the two methods.
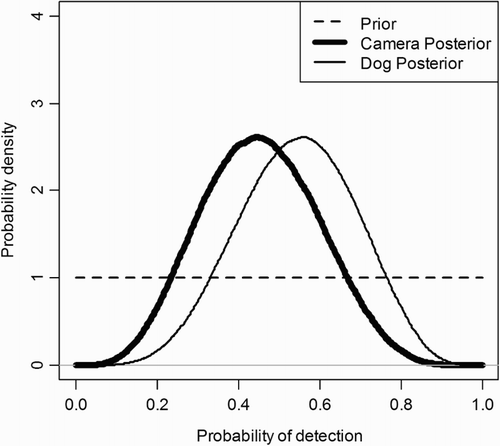
The power analysis indicated that the number of searched cells in our trial would only be sufficient to detect a very large difference (> 0.6) in detection probability between the two methods (). For simplicity we have assigned the true detection probability for cameras at 0.1 and compared this with a range of detection probabilities for dogs. However, if the true probability for cameras was closer to 0.5, the required sample sizes would be larger.
Table 2. Power analysis showing the number of search cells required to detect a difference (pDiff) in detection probability of feral cats (Felis catus) using camera traps (pCamera) and wildlife detector dogs (pDog).
Cost estimates
Because the trial was cut short when the dog team had covered approximately half the study area, we estimated the cost of surveying the entire study area by doubling the expenses incurred by the dog team in the field. This assumes that a similar number of search cells would have been sampled each day. We estimated the operating cost to sample both study sites would be similar using wildlife detector dogs or camera traps ().
Table 3. Operating cost of monitoring for cats (Felis catus) on Waitere and Toronui Stations, Hawke's Bay, New Zealand, using camera traps and wildlife detector dogs.
Discussion
Our study suggests that the probability of detecting cats per search event was similar using either camera traps or wildlife detector dogs. Detection probabilities would be likely to vary depending on the search effort involved; therefore these estimates depend on the definition of a search event. The search effort applied in this trial was intended to represent a realistic scenario for the practical use of each method.
Although both methods detected cats in a similar number of search cells, there were only two cells in which cats were detected by both dogs and cameras. This may be a result of the different spatial and temporal scales over which these methods operate; cameras sampled at a single point over 3 weeks, whereas the dog team searched more widely within each search cell, but took a more instantaneous snapshot. It is possible that the dog team may have detected scats that were more than 3 weeks old, which may have been deposited by cats that were removed by the predator control. In this case, the estimated detection probability using wildlife detector dogs would be inflated relative to the estimate derived for camera traps. Our estimates of detection probability have wide confidence intervals, and should be regarded only as indicative. More precise estimates would require larger sample sizes than were obtained in this trial.
The estimated operating cost of each method was also comparable; however, this does not include the purchase price of the camera traps, which was NZ$56,000. Although less expensive camera traps are available, detection rates can vary substantially between different models (Meek & Pittet Citation2012; Meek et al. Citation2012; Glen et al. Citation2013b). We do not know whether less expensive camera traps could have achieved a comparable result.
Our cost comparison is limited to operating costs for a single survey, and does not include the cost of purchasing cameras. Ideally, depreciation of camera traps should be included; however, we have no data on the useful life of camera traps. Future research should compare the two methods for repeated surveys over a number of years. Their relative cost-effectiveness will be influenced by the number of times cameras can be re-deployed. This will depend on their durability, as well as the conditions in which they are deployed (e.g. weather conditions, likelihood of theft).
Camera traps deployed for longer than 3 weeks may have achieved a higher detection probability (e.g. Robley et al. Citation2010). However, the time taken for cameras to detect cats in a search cell ranged from 1 to 3 days, suggesting that 3 weeks was adequate. Although it is difficult to estimate the additional cost of deploying cameras for longer (e.g. additional analysis of footage, higher risk of camera loss/damage), it is likely these would be small compared with the costs of camera deployment and retrieval. However, a longer deployment would be less likely to satisfy assumptions of population closure.
Our estimates of detection probability using wildlife detector dogs and camera traps may help to inform the design of future studies, to estimate relative abundances of feral cats, and to assess the likelihood that eradication efforts have succeeded (e.g. Ramsey et al. Citation2011). To detect a significant difference in probability of detection between cameras and dogs would require a larger sample size than was achieved in this study. Therefore, although our results suggest that the methods were comparable, further trials with larger sample sizes are required. Regardless, our trial illustrated a number of advantages and disadvantages associated with camera trapping and wildlife detector dogs. The effectiveness of the dog team was dependent on fair weather as the dogs’ ability to detect scent is reduced by rain or strong wind (S. Aitcheson, DOC, pers. comm.). Our cost estimates are therefore based on the assumption of 10 days of fine weather; interruptions due to bad weather would increase costs. In contrast, camera traps can operate in a wide range of weather conditions, and can be deployed for long periods so that individual weather events are less likely to influence overall results.
Searching with dogs can cover an area more quickly and thoroughly than deploying camera traps at fixed positions. This may be particularly useful when rapid detection is required, e.g. a suspected incursion into a predator-free reserve. On the other hand, camera traps can operate for long periods with little or no maintenance.
Another potential advantage of wildlife detector dogs is their ability to help catch and remove animals that have survived a control programme (e.g. Ramsey et al. Citation2011). In contrast, camera traps are a monitoring tool only.
Camera traps may be unsuitable for use in some areas because of their vulnerability to interference by people and/or livestock. In our trial, livestock were frequently photographed by camera traps, producing many thousands of pictures and increasing the time taken to review the footage. Livestock also knocked or rubbed against cameras, sometimes leaving them inoperative. In contrast, a dog team can operate effectively in the presence of livestock. Because our trial was conducted on private property, human visitation was minimal. One camera was stolen; however, the risk of theft may be much greater in areas open to the public.
Finally, camera traps can give useful data on a wide range of other species. In the course of our trial the cameras detected numerous species of mammals and birds in addition to cats (P. Garvey and M. Nichols, unpublished data). Although collating the data is time-consuming, such information may often be valuable to researchers or land managers.
Future research
Our study suggests that both camera traps and wildlife detector dogs may be useful for monitoring feral cats. It also draws attention to a number of questions and priorities for further research:
How do detection probabilities for cats compare with those for other species? Detector dogs have been used to search for various species of carnivores (Wasser et al. Citation2004; Smith et al. Citation2005; Gompper et al. Citation2006; Reindl-Thompson et al. Citation2006; Long et al. Citation2007a; Dematteo et al. Citation2009; Brown et al. Citation2011) and rodents (Gsell et al. Citation2010; Duggan et al. Citation2011; Shapira et al. Citation2011). Detection probabilities can vary widely between species (e.g. Long et al. Citation2007a). However, we know of no studies that compare detection probabilities of feral cats with those of other sympatric carnivores.
Is it more efficient for dogs to specialise in detecting one species, or to search for all carnivore scats, then use DNA to assign them to species? When combined with genetic analysis of scats, wildlife detector dogs can provide a fast, reliable and inexpensive way to survey for cryptic species (Long et al. Citation2007a). In Tasmania, trained dogs detected 80% of fox (Vulpes vulpes) scats, even after the scats had been in the field for 63 days. After 91 days in the field, 99% of fox scats were genetically identifiable to species (Brown et al. Citation2011; Caley et al. Citation2015; but see also Marks et al. Citation2014).
Identifying scats to species may allow population density estimates through occupancy modelling, whereas identifying individual animals allows mark–recapture analysis (Marks et al. Citation2009; Gleeson et al. Citation2010). Identifying individuals can also tell us about their movements and behaviour. However, identifying individuals from scat DNA is more expensive and requires fresher samples than identifying species. Hence, there may be a trade-off between cost and rigour of different techniques.
Once the above questions have been addressed, the cost-effectiveness of detector dogs and scat DNA should be compared with other non-invasive techniques such as camera trapping.
We conclude that the choice to use camera traps or wildlife detector dogs will depend on the study aims (e.g. research versus eradication), as well as site-specific factors such as weather, land-use and degree of human visitation. Although further testing is required to compare long-term cost-effectiveness, both methods can detect feral cats at relatively high and low densities.
Acknowledgements
We are grateful to S, K and B Aitcheson, M Perry, R Dickson and S Cave for logistical support, and to B Cheetham (Landcorp, Waitere Station) and D Schaw (Toronui Station) for site access, accommodation and assistance. K Vincent (DOC Conservation Dog Team) provided helpful advice. We thank M Barron, R Pech, G Norbury and two anonymous referees for helpful comments on a previous draft.
Associate Editor: Dr Grant Norbury.
Disclosure statement
No potential conflict of interest was reported by the authors.
Additional information
Funding
References
- Abbott I, Peacock D, Short J. 2014. The new guard: the arrival and impacts of cats and foxes. In: Glen AS, Dickman CR, editors. Carnivores of Australia: past, present and future. Collingwood: CSIRO Publishing; p. 69–104.
- Algar D, Angus GJ, Williams MR, Mellican AE. 2007. Influence of bait type, weather and prey abundance on bait uptake by feral cats (Felis catus) on Peron Peninsula, Western Australia. Conservation Science Western Australia. 6:109–149.
- Bengsen A, Butler J, Masters P. 2011a. Estimating and indexing feral cat population abundances using camera traps. Wildlife Res. 38:732–739. doi:10.1071/WR11134.
- Bengsen AJ, Leung LK-P, Lapidge SJ, Gordon IJ. 2011b. Using a general index approach to analyze camera-trap abundance indices. J Wildlife Manage. 75:1222–1227. doi: 10.1002/jwmg.132
- Brook LA, Johnson CN, Ritchie EG. 2012. Effects of predator control on behaviour of an apex predator and indirect consequences for mesopredator suppression. J Appl Ecol. 49:1278–1286. doi:10.1111/j.1365-2664.2012.02207.x.
- Brown B, Gaffney R, Pauza M, Barclay C, MacDonald A, Sarre S. 2011. The mysterious case of the disappearing poo: fox scat degradation in Tasmania. In: Saunders G, Lane C eds. Proceedings of the 15th Australasian Vertebrate Pest Conference, Australasian Vertebrate Pest Committee, Sydney, 2011; p. 161.
- Brown WE, Ramsey DSL, Gaffney R. 2015. Degradation and detection of fox (Vulpes vulpes) scats in Tasmania: evidence from field trials. Wildlife Res. 41:681–690. doi: 10.1071/WR14152
- Caley P, Ramsey DSL, Barry SC. 2015. Inferring the distribution and demography of an invasive species from sighting data: the red fox incursion into Tasmania. PLoS ONE. 10:e0116631. doi:10.1371/journal.pone.0116631.
- Campbell KJ, Harper G, Algar D, Hanson CC, Keitt BS, Robinson S. 2011. Review of feral cat eradications on islands. In: Veitch CR, Clout MN, Towns DR eds. Island Invasives: Eradication and Management. Proceedings of the International Conference on Island Invasives. Gland, Switzerland and Auckland, New Zealand, IUCN; p. 37–46.
- Clark JS. 2005. Why environmental scientists are becoming Bayesians. Ecol Lett. 8:2–14. doi:10.1111/j.1461-0248.2004.00702.x.
- Clayton R, Cowan P. 2010. Management of animal and plant pests in New Zealand - patterns of control and monitoring by regional agencies. Wildlife Res. 37:360–371. doi:10.1071/WR09072.
- Cruz J, Glen AS, Pech RP. 2013. Modelling landscape-level numerical responses of predators to prey: the case of cats and rabbits. PLoS ONE. 8:e73544. doi:10.1371/journal.pone.0073544.
- Dematteo KE, Rinas MA, Sede MM, Davenport B, Argüelles CF, Lovett K, Parker PG. 2009. Detection dogs: an effective technique for bush dog surveys. J Wildlife Manage. 73:1436–1440. doi:10.2193/2008-545.
- Duggan JM, Heske EJ, Schooley RL, Hurt A, Whitelaw A. 2011. Comparing detection dog and livetrapping surveys for a cryptic rodent. J Wildlife Manage. 75:1209–1217. doi:10.1002/jwmg.150.
- Gelman A, Carlin JB, Stern HS, Rubin DB. 2004. Bayesian data analysis. Boca Raton, FL: Chapman & Hall/CRC.
- Gleeson DM, Byrom A, Howitt R. 2010. Non-invasive methods for genotyping of stoats (Mustela erminea) in New Zealand: potential for field applications. New Zeal J Ecol. 34:356–359.
- Glen AS, Atkinson R, Campbell KJ, Hagen E, Holmes ND, Keitt BS, Parkes JP, Saunders A, Sawyer J, Torres H. 2013a. Eradicating multiple invasive species on inhabited islands: the next big step in island restoration? Biol Invasions. 15:2589–2603. doi: 10.1007/s10530-013-0495-y
- Glen AS, Cockburn S, Nichols M, Ekanayake J, Warburton B. 2013b. Optimising camera traps for monitoring small mammals. PLoS ONE. 8:e67940. doi:10.1371/journal.pone.0067940.
- Glen AS, Warburton B, Cruz J, Coleman M. 2014. Comparison of camera traps and kill traps for detecting mammalian predators: a field trial. New Zeal J Zool. 41:155–160. doi:10.1080/03014223.2014.898667.
- Gompper ME, Kays RW, Ray JC, Lapoint SD, Bogan DA, Cryan JR. 2006. A comparison of noninvasive techniques to survey carnivore communities in northeastern North America. Wildlife Society Bulletin. 34:1142–1151. doi:10.2193/0091-7648(2006)34[1142:ACONTT]2.0.CO;2.
- Gsell A, Innes J, de Monchy P, Brunton D. 2010. The success of using trained dogs to locate sparse rodents in pest-free sanctuaries. Wildlife Res. 37:39–46. doi:10.1071/WR09117.
- Innes J, Kelly D, Overton JMcC, Gillies C. 2010. Predation and other factors currently limiting New Zealand forest birds. New Zeal J Ecol. 34:86–114.
- Karanth KU. 1995. Estimating tiger Panthera tigris populations from camera-trap data using capture-recapture models. Biol Conserv. 71:333–338. doi: 10.1016/0006-3207(94)00057-W
- Long RA, Donovan TM, Mackay P, Zielinski WJ, Buzas JS. 2007a. Comparing scat detection dogs, cameras, and hair snares for surveying carnivores. J Wildlife Manage. 71:2018–2025. doi:10.2193/2006-292.
- Long RA, Donovan TM, Mackay P, Zielinski WJ, Buzas JS. 2007b. Effectiveness of scat detection dogs for detecting forest carnivores. J Wildlife Manage. 71:2007–2017. doi:10.2193/2006-230.
- Lyra-Jorge MC, Ciocheti G, Pivello VR, Meirelles ST. 2008. Comparing methods for sampling large- and medium-sized mammals: camera traps and track plots. Eur J Wildlife Res. 54:739–744. doi:10.1007/s10344-008-0205-8.
- Marks CA, Gigliotti F, McPhee S, Piggott MP, Taylor A, Glen AS. 2009. DNA genotypes reveal red fox (Vulpes vulpes) abundance, response to lethal control and limitations of contemporary survey techniques. Wildlife Res. 36:647–658. doi: 10.1071/WR08109
- Marks CA, Obendorf D, Pereira F, Edwards I, Hall GP. 2014. The dispersion and detection patterns of mtDNA-assigned red fox Vulpes vulpes scats in Tasmania are anomalous. J Appl Ecol. 51:1033–1040. doi: 10.1111/1365-2664.12278
- Medina FM, Bonnaud E, Vidal E, Nogales M. 2014. Underlying impacts of invasive cats on islands: not only a question of predation. Biodivers Conserv. 23:327–342. doi:10.1007/s10531-013-0603-4.
- Medina FM, Bonnaud E, Vidal E, Tershy BR, Zavaleta ES, Donlan CJ, Keitt BS, Le Corre M, Horwarth SV, Nogales M. 2011. A global review of the impacts of invasive cats on island endangered vertebrates. Global Change Biol. 17:3503–3510. doi:10.1111/j.1365-2486.2011.02464.x.
- Meek P, Fleming P, Ballard G. 2012. An introduction to camera trapping for wildlife surveys in Australia. Canberra: Invasive Animals CRC.
- Meek PD, Fleming PJS, Ballard G, Banks P, Claridge AW, Sanderson J, Swann D, editors. 2014. Camera trapping: wildlife research and management. Collingwood: CSIRO Publishing.
- Meek PD, Pittet A. 2012. User-based design specifications for the ultimate camera trap for wildlife research. Wildlife Res. 39:649–660. doi: 10.1071/WR12138
- Moseby KE, Hill BM. 2011. The use of poison baits to control feral cats and red foxes in arid South Australia I: aerial baiting trials. Wildlife Res. 38:338–349. doi:10.1071/WR10235.
- Nogales M, Martin A, Tershy BR, Donlan CJ, Witch D, Puerta N, Wood B, Alonso J. 2004. A review of feral cat eradication on islands. Conserv Biol. 18:310–319. doi:10.1111/j.1523-1739.2004.00442.x.
- Ramsey DSL, Parkes JP, Will D, Hanson CC, Campbell KJ. 2011. Quantifying the success of feral cat eradication, San Nicolas Island, California. New Zeal J Ecol. 35:163–173.
- Reardon JT, Whitmore N, Holmes KM, Judd LM, Hutcheon AD, Norbury G, Mackenzie DI. 2012. Predator control allows critically endangered lizards to recover on mainland New Zealand. New Zeal J Ecol. 36:141–150.
- Recio MR, Mathieu R, Maloney R, Seddon PJ. 2010. First results of feral cats (Felis catus) monitored with GPS collars in New Zealand. New Zeal J Ecol. 34:288–296.
- Reindl-Thompson SA, Shivik JA, Whitelaw A, Hurt A, Higgins KF. 2006. Efficacy of scent dogs in detecting black-footed ferrets at a reintroduction site in South Dakota. Wildlife Society Bulletin. 34:1435–1439. doi:10.2193/0091-7648(2006)34[1435:EOSDID]2.0.CO;2.
- Robley A, Gormley A, Woodford L, Lindeman M, Whitehead B, Albert R, Bowd M, Smith A. 2010. Evaluation of camera trap sampling designs used to determine change in occupancy rate and abundance of feral cats. Arthur Rylah Institute for Environmental Research Technical Report No. 201. Heidelberg, VIC: Department of Sustainability and Environment.
- Robley A, Ramsey D, Woodford L, Lindeman M, Johnston M, Forsyth D. 2008. Evaluation of detection methods and sampling designs used to determine the abundance of feral cats. Heidelberg, VIC: Arthur Rylah Institute for Environmental Research, Department of Sustainability and Environment.
- Salo P, Korpimäki E, Banks PB, Nordström M, Dickman CR. 2007. Alien predators are more dangerous than native predators to prey populations. Proc R Soc Lond B Biol Sci. 274:1237–1243. doi: 10.1098/rspb.2006.0444
- Shapira I, Buchanan F, Brunton DH. 2011. Detection of caged and free-ranging Norway rats Rattus norvegicus by a rodent sniffing dog on Browns Island, Auckland, New Zealand. Conserv Evid. 8:38–42.
- Simberloff D. 2010. Invasive species in New Zealand. In: Sodhi NS, Ehrlich PR, editors. Conservation biology for all. Oxford: Oxford University Press; p. 132–133.
- Smith DA, Ralls K, Cypher BL, Maldonado JE. 2005. Assessment of scat-detection dog surveys to determine kit fox distribution. Wildl Soc Bull. 33:897–904. doi:10.2193/0091-7648(2005)33[897:AOSDST]2.0.CO;2.
- Wasser SK, Davenport B, Ramage ER, Hunt KE, Parker M, Clarke C, Stenhouse G. 2004. Scat detection dogs in wildlife research and management: application to grizzly and black bears in the Yellowhead Ecosystem, Alberta, Canada. Can J Zool. 82:475–492. doi:10.1139/z04-020.
- Witmer GW. 2005. Wildlife population monitoring: some practical considerations. Wildlife Res. 32:259–263. doi:10.1071/WR04003.