ABSTRACT
Distribution, nymphal habitat and genetic structure of the New Zealand mayfly Isothraulus abditus were investigated to improve our understanding of its ecology and conservation status. A description of the undescribed subimaginal stage is also provided. Isothraulus abditus has now been recorded from 55 North Island streams, 47 being new to published literature. Its distribution currently extends from Mangonui in Northland to Taumatatahi near Whanganui. Nymphs live in forested stream pools and backwaters containing organic detritus. Genetic structure of six populations was assessed using the mitochondrial cytochrome c oxidase subunit I (COI) gene. Thirty-six haplotypes were identified from 123 individuals, and a maximum uncorrected genetic distance of 1.8% indicated that specimens belong to a single species. However, those within Northland showed some genetic differentiation. Restricted habitat, sparse distribution and some genetic differentiation of I. abditus suggest its conservation status should be classified as ‘At Risk’.
LSID: urn:lsid:zoobank.org:pub:75C386BC-C0A5-4627-AE4B-8C5D749D53BA
Introduction
Mayflies (Ephemeroptera) are widely distributed in freshwaters throughout the world. In many streams and rivers they are among the most abundant benthic invertebrates and are an important food supply for fish and other predators (Hynes Citation1970). Mayflies can also be valuable indicators of organic enrichment as they are particularly sensitive to changes in water quality and the degradation of catchments (Towns and Peters Citation1996). However, not all species are equally sensitive to different kinds of pollution (Stark Citation1993; Gray and Harding Citation2012) and much more knowledge of their distributions, habitat requirements and biology is needed if their role as ecological indicators is to be optimised. Because of their sensitivity to changes in habitat loss and land use, mayflies may also require conservation effort if viable populations of individual species are to be maintained.
Towns and Peters (Citation1996) considered New Zealand’s fringe-gilled mayfly Isothraulus abditus Towns and Peters, Citation1979 to be one of New Zealand’s rarest mayflies. The specific name abditus, meaning hidden, refers to the biology of the nymphs (Towns and Peters Citation1979); a condition that has undoubtedly contributed to the perception of rarity. Isothraulus abditus was described from specimens collected in small streams of Auckland’s Waitakere Ranges (Towns and Peters Citation1979) following a pioneering study of freshwater invertebrate communities by Towns (Citation1976). Imagos (adults) of I. abditus are small insects with a body length of 6.7–7.9 mm and forewings 7.5–8.8 mm long. Distinctive features are the narrow, elongate male penes, which are at least half the length of the genital forceps, and a ventral egg guide (ovipositor) that extends the length of abdominal segment 8 in females. The subimaginal stage (subadult) has not been described, although Towns and Peters (Citation1979) listed a female subimago among their paratype material from the Waitakere River. The nymph is distinctive, as the dorsal and ventral portions of each gill lamella are fringed with narrow finger-like lappets, some of which may be longer than the lamella itself.
Isothraulus abditus is the only known species in its genus and may have relatives in New Caledonia (Towns and Peters Citation1980, Citation1996). There are also numerous morphological similarities between Isothraulus and the tropical genus Thraulus, including the distinctive fringed gills of the nymph. Its closest relative in the New Zealand fauna is Tepakia (Towns and Peters Citation1996) and numerous morphological similarities have also been noted between Isothraulus and a third New Zealand genus, Zephlebia, to which I. abditus was initially referred (Towns Citation1976, Citation1978). A recent molecular study of world Leptophlebiidae based on histone H3 and 28S ribosomal DNA consistently grouped Tepakia, Isothraulus and Zephlebia together within a Palaeoaustral group of genera (O'Donnell and Jockusch Citation2008), but distant from Thraulus, suggesting that similarities between I. abditus and Thraulus spp. may result from convergence. Beet (Citation2016) also reported that Isothraulus and Zephlebia grouped together in a phylogenetic analysis using the mitochondrial cytochrome c oxidase subunit I (COI) gene; Tepakia was not included in her study.
Published records identified I. abditus in the vicinity of Auckland and on nearby Great Barrier Island (Towns and Peters Citation1979, Citation1996; Towns Citation1987), and in the adjacent regions of Northland and Coromandel (Collier Citation1995; Hitchings Citation2001; Hitchings et al. Citation2015; Pohe et al. CitationForthcoming 2018). It was also thought to be present on Little Barrier Island, which lies off the Auckland coast (personal communication from T.K. Crosby to D.R. Towns, cited in Towns and Peters Citation1979). Isothraulus abditus was listed as of potential conservation interest by Collier (Citation1992, Citation1993) due to its known distribution being restricted to two ecological regions (sensu McEwen Citation1987), Auckland and Coromandel. Towns (Citation1987) found numerous nymphs in an isolated pool filled with leaves and twigs on Great Barrier Island and other nymphs in first-order streams with little discernible water movement; stream habitats to which little ecological attention has been paid. These observations led him to suggest that intermittent streams might provide the most common habitat for nymphs, despite water in isolated, leaf-filled pools often providing poor-quality conditions for most mayfly nymphs. This is because such habitats probably develop depleted levels of dissolved oxygen and higher water temperature than the mainstream. However, no further work has been done to corroborate the observations of Towns (Citation1987) that intermittent streams are indeed common habitats of I. abditus nymphs, or whether such environments are able to support them.
Genetic structure, an important tool in the management of species of conservation interest, has only been investigated in three species of New Zealand mayfly. Coloburiscus humeralis (Walker, 1853) showed minimal allelic differences that appeared unrelated to geographic proximity across its New Zealand distribution (Hogg et al. Citation2002). However, Acanthophlebia cruentata (Hudson, 1904) and Siphlaenigma janae Penniket, 1962 showed moderate to high levels of genetic differentiation among some North Island populations, and both species had unique haplotypes in the far north of New Zealand (Smith and Collier Citation2001; Smith et al. Citation2006; Pohe et al. Citation2018).
The aims of the present study were to (1) update the known distribution of I. abditus based on extensive surveys undertaken by the authors, and records of its occurrence in published and unpublished sources, including museum specimen collections; (2) investigate habitat preference of the nymphs through a quantitative benthic survey, with particular attention to the association of nymphal occurrence and environmental conditions; (3) investigate the genetic structure of the species using DNA sequence data of the mitochondrial COI gene fragment to examine genetic structure within and between localities; and (4) assess the conservation status and requirements of the species using the new information we present. Based on material collected by the lead author during light trapping surveys we also provide a description of the previously undescribed subimago (Appendix).
Methods
Distribution
The distribution of I. abditus was reviewed using published literature (Towns and Peters Citation1979, Citation1996; Towns Citation1987; Collier Citation1995; Hitchings Citation2001; Hitchings et al. Citation2015; Pohe et al. CitationForthcoming 2018), a wide range of unpublished sources, and numerous stream surveys. Unpublished sources included records from the Auckland, Canterbury, Otago and New Zealand (Te Papa) Museums, New Zealand Arthropod Collection, specimens (confirmed by the lead author) from student theses (Cook Citation2002; Pohe Citation2008; Arrieta Citation2010, Citation2012), consultancy reports (Quinn and Parkyn Citation1996; Quinn and Halliday Citation1998; Parkyn et al. Citation2006), State of the Environment monitoring data (1995–2011; MFE Citation2015), and personal communications with other freshwater ecologists (see Acknowledgements). For the stream surveys, imagos, subimagos and nymphs of I. abditus were obtained from 14 localities in 2013–2016 during an extensive nationwide light trapping and benthic sampling survey for mayflies and stoneflies (83 sites; see Winterbourn et al. Citation2017). Specimens were identified using standard New Zealand stream invertebrate texts (Towns and Peters Citation1996; Winterbourn et al. Citation2006). All records were plotted on a map of the North Island () and a complete catalogue of records is provided as supplementary material (Table S1).
Figure 1. Distribution records for Isothraulus abditus. Blue points indicate locations of specimens recorded in published literature, grey points indicate locations of specimens recorded in unpublished literature (reports, theses, monitoring data, national specimen collections and personal communications) and black triangles indicate locations of specimens recorded in a national survey (Pohe 2013–2016). Red circles with corresponding codes indicate locations from which specimens for DNA analysis were obtained in this study. Code details are described in .
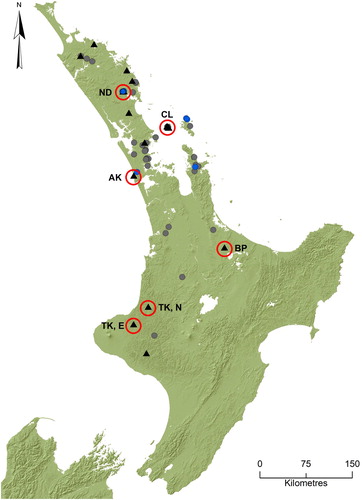
Habitat preference
Habitat use by nymphs of I. abditus was investigated in 2015, in riffles, pools and backwaters of Mangere Stream in Northland. The study was undertaken during mid-September when nymphs of all sizes were still present in the stream (peak adult emergence is December–January). Mangere Stream was selected because I. abditus was known to be present there in moderately high numbers (Pohe et al. CitationForthcoming 2018). Physico-chemical (environmental) variables were recorded at each sampling point before invertebrate sampling. A YSI Professional Plus handheld meter with Quatro multiparameter sensor was used to record pH, dissolved oxygen, conductivity and water temperature. Percentage cover of organic detritus (submerged leaves and twigs on the stream bed), estimated visually to the nearest 10%, was recorded for each sampling point. Depth of water at each sampling point was measured to the nearest centimetre, and mean stream velocity (three readings at each sampling point) was recorded with a Marsh–McBirney Flo-Mate (Model 2000). Sampling was conducted within an 8 hour period on a single day, within a 1.5 km study reach (centre −35.7106°; 174.2452°) that flowed through mixed podocarp–broadleaf forest and had stream widths of 1.9–4.2 m. Because no heavy rainfall events had occurred in the 2 months before sampling, backwater habitats had not been inundated by water from the main channel during that period.
Thirty quantitative benthic samples were taken in each habitat. Individual sampling positions for pools and riffles were selected at approximately 50 m intervals, whereas backwaters were sampled whenever they were encountered (backwaters were a less-common habitat). Riffles were sampled with a 25 cm × 25 cm Surber sampler (0.0625 m2; 250 µm mesh), whereas both the pools and backwaters were sampled by disturbing the substratum within a 25 cm × 25 cm quadrat (0.0625 m2) and scooping up dislodged material with a hand-net (300 µm mesh). Mayfly sampling was done systematically in an upstream direction to avoid the possibility of re-sampling individuals. The distinctive nymphs of I. abditus were counted in the field, their body lengths were measured to the nearest 1 mm, then most were returned alive to their respective sampling points. Small mayfly nymphs of uncertain identity were retained and checked in the laboratory by microscopic examination using a Nikon 10–63× stereomicroscope (Model SMZ800). Specimens were assigned to one of three size classes, which also corresponded approximately to the following nymphal development stages: < 4 mm, wing pads absent; 4–8 mm, developing wing pads present but not extending over abdominal segment 1; and > 8 mm, wing pads extending beyond abdominal segment 1.
Genetic structure
DNA sequences were obtained from 123 specimens (imaginal and nymphal life stages) of I. abditus, sourced from six locations (, ), using the standard ‘barcoding’ fragment of the mitochondrial COI gene (Hebert et al. Citation2003). Sample locations were chosen that provided the largest number of I. abditus specimens available, and that also provided a broad geographic coverage of the known distribution. Only two individuals were obtained from a population in Auckland but were included in the data set because the samples were collected only 8 km from the type locality of I. abditus, and within the same forest. DNA was extracted from three legs of each specimen with an Extract-N-Amp Tissue PCR Kit (Sigma-Aldrich, St Louis, MO, USA), the legs being crushed and added to 25 µL Extraction Solution and 7.0 µL Tissue Preparation Solution, incubated in a thermocycler at 37 °C for 30–45 min, then at 95 °C for 3 min, after which 25 µL Neutralising Solution was added. The barcode fragment of the COI gene was amplified using the universal primer pair LCO1490 (5′-GGTCAACAAATCATAAAGATATTGG-3′) and HCO2198 (5′-TAAACTTCAGGGTGACCAAAAAATCA-3′) (Folmer et al. Citation1994). Polymerase chain reaction (PCR) amplifications were performed in a final volume of 15 µL containing 2× Kapa2G Robust HotStart ReadyMix (7.5 µL), 0.35 µm of each primer (1 µL), nanopure water (5.5 µL) and 1.0 µL of template DNA (118–276 ng/µL). Thermal cycling parameters included an initial denaturation at 95 °C for 3 min, followed by 35 cycles at 95 °C for 15 s, 50 °C for 20 s, 72 °C for 30 s and a final 5 min extension at 72 °C. PCR products were purified with incubation in 2 µL ExoSAP-IT reagent and sequenced in both directions, using the same PCR primers used for amplification, by MacroGen (Seoul, South Korea). Forward and reverse sequences were aligned manually and trimmed to 658 base pairs (bp) using BioEdit Sequence Alignment Editor version 7.2.5 (Hall Citation1999) and deposited in GenBank (Benson et al. Citation2013) (Accession Numbers MH127930–MH127965).
Table 1. Locations and site details used in the genetic structure study.
Statistical analyses and phylogenetic inferences
To assess habitat preference, a t-test was used to compare differences in nymphal abundance between pools and backwaters (no nymphs were found in riffles). Differences in proportions of small, medium and large nymphs between the two habitats were compared with a chi-square test of association. Spearman rank correlation was used to determine whether within-habitat abundance of nymphs was associated with particular physico-chemical variables.
Haplotype composition and a statistical parsimony network were calculated using TCS version 1.21 software (Clement et al. Citation2000). Evolutionary relationships were then displayed with a Median-Joining network (Bandelt et al. Citation1999) (ε = 0) in PopART version 1.7 (Leigh and Bryant Citation2015). Genetic distance within and among the six populations was estimated in MEGA 7.0 (Kumar et al. Citation2016) using the uncorrected p-distance method (see Collins et al. Citation2012), and also with the Kimura two-parameter model (Kimura Citation1980) to facilitate comparison with previously published studies. Our pairwise distance estimates employed the best-fitting nucleotide substitution model: a general time-reversible model with γ-distributed among-site rate variation and invariant sites (GTR + G + I; shape parameter 0.9566; bootstrap = 2000), which was calculated in MEGA 7.0 and selected using the corrected Akaike Information Criterion (Akaike Citation1973). Genetic diversity was estimated with Nei’s (Citation1987) gene (Hd) and nucleotide diversity (π) indices in Arlequin version 3.5.1.3 (Excoffier and Lischer Citation2010). Analysis of Molecular Variance (AMOVA; 2000 permutations) was also run in Arlequin to examine the effect of sample location on genetic variation using all data grouped as one ‘New Zealand’ data set, followed by the calculation of pairwise Φst population differentiation values (F-statistics). All results were considered to be statistically significant at α < 0.05.
Results
Distribution
Isothraulus abditus has now been recorded from 55 streams in 37 localities (75 records in total: 9 published, 48 unpublished, 18 new). Its distribution is recorded from Mangonui Forest (35.0152°S) in northern Northland, to the vicinity of Taumatatahi (39.5432°S) near Whanganui (), from an altitudinal range of 10–365 m above sea level. It has not been reported from the southeastern North Island or the South Island, despite the considerable amount of sampling done there. Data from all previous sources (published and unpublished) indicated that of the 57 records, most (88%) were nymphal specimens, and only 12% contained adult material. However, in the recent nationwide survey, 166 individuals were collected from 14 localities, 92% of which were winged stages obtained by light trapping. Of the small number of nymphs collected, five were male and eight were female. Adult males were the most commonly trapped winged life stage (82%), followed by subadult males (10.5%), adult females (7%) and subadult females (0.5%). The largest light trap collections were taken beside first- and second-order streams in mixed podocarp–broadleaf forests on Little Barrier Island (n = 46), in northern Taranaki (n = 35) and in Northland (n = 33), and of the 83 New Zealand streams sampled, I. abditus was only collected from forested sites.
Habitat preference
The three habitat types sampled (riffles, pools, backwaters) were distinctive, particularly with respect to water depth, water velocity and organic cover (). Riffles were shallow (mean 0.16 m), stony sections of stream bed with current velocities 0.3–0.9 ms−1, whereas pools were 0.3–0.6 m deep, had current velocities < 0.1 ms−1 and contained deposits of organic matter. Backwaters were zones at the sides of the stream with negligible water movement and also contained organic deposits (pools and backwaters without organic matter were not sampled). Backwaters were either connected to the main channel by shallow transfer zones, or isolated from the main channel by gravel bars, but generally connected to it by subterranean flow.
Table 2. Mean water depth, water velocity, organic cover and abundance of nymphs of Isothraulus abditus in three habitats sampled in the Mangere River, Northland in September 2015.
No I. abditus nymphs were found in riffle samples but 177 nymphs were taken from pools and backwaters. They were widely distributed within these two habitats, being present in 16 of 30 samples from pools and 24 of 30 samples from backwaters (means of 26/m2 and 68/m2, respectively; ). Mean abundance of nymphs was significantly higher in backwaters than pools (t = 2.97, P < 0.01) but proportions of nymphs categorised as small, medium and large taken from pools and backwaters (n = 18, 22, 9 and 33, 61, 34, respectively) did not differ significantly (χ2 = 2.51, P = 0.29). We also found that abundance of nymphs was strongly associated with organic cover at the individual quadrat level in both pools and backwaters (). Negative correlations with abundance of nymphs were found with dissolved oxygen and pH, which varied considerably at some backwater sampling points (dissolved oxygen 1.7–9.9 mg/L; pH 5.3–7.2; see Table S2 for physico-chemical data summary).
Table 3. Spearman rank correlations (rs) between nymphal abundance of Isothraulus abditus and organic cover, dissolved oxygen and pH in pools and backwaters.
The most abundant macroinvertebrates found in backwater habitats (backwaters with and without I. abditus) were a second leptophlebiid mayfly Arachnocolus phillipsi Towns and Peters, Citation1979 and unidentified mites (Acari) and amphipods. The mayflies Tepakia caligata Towns and Peters, Citation1996 and Zephlebia borealis (Phillips, 1930), and small (< 30 mm total length) crayfish, Paranephrops planifrons White, 1842, were also recorded occasionally in backwater samples. Mites, various dipteran larvae and two mayflies, Z. borealis and Zephlebia versicolor (Eaton, 1899), were the most common invertebrates in pools, which were also inhabited by the mayflies Zephlebia dentata (Eaton, 1871) and Neozephlebia scita (Walker, 1853), the stonefly Austroperla cyrene (Newman, 1845), the caddisfly Triplectides obsoletus (McLachlan, 1862) and larvae of the dragonfly Antipodochlora braueri (Selys, 1871).
Genetic structure
Sequence data for the standard barcode fragment of the mitochondrial COI gene were obtained from 123 I. abditus specimens, collected at six locations across the North Island of New Zealand. There was no evidence of saturation in the data, mean nucleotide composition was T = 30.8%, C = 25.1%, A = 22.6% and G = 21.5%, and of the 658-bp positions, 39 were variable and 13 were parsimony-informative. Overall nucleotide diversity (π) was 0.004 and from the 123 sequences generated, 36 haplotypes were identified, 28 (78%) of which were singletons and 34 (94%) were unique to single populations. Mean p-distance among the 36 haplotypes was 0.7% (see Table S3 for individual haplotype p-distances and standard error estimates). Overall population haplotype diversity (Hd) was 0.7 (± 0.05 SD) and for the five populations represented by 12 or more individuals (i.e. excluding Auckland, n = 2) intra-population haplotype diversity ranged from 0.86 and 0.84 in eastern Taranaki and Northland, respectively, to 0.13 on Little Barrier Island ().
Table 4. Collection and genetic diversity information for Isothraulus abditus specimens used in the study, based on a 658-bp fragment of the cytochrome c oxidase subunit I (COI) barcoding gene.
The haplotypes formed a single parsimony network (), which comprised two star-shaped phylogenies and a poorly resolved cluster, all linked to a central common ancestor. One star-shaped phylogeny included 12 of the 15 observed Northland haplotypes (a Northland cluster), one of which was common to 11 individuals; the other 14 Northland haplotypes comprised only one to three individuals. Isothraulus abditus from Northland shared no haplotypes with individuals from other regions. However, three Northland haplotypes were included in the poorly resolved cluster, together with the two Auckland haplotypes (i.e. Northland was not monophyletic), and all three were more closely related to haplotypes from regions further south (). One common haplotype (n = 67) was shared among 74% of sequenced individuals from Rotorua, northern Taranaki, eastern Taranaki, and Little Barrier Island and formed the core of the second star phylogeny (a central North Island cluster). A second haplotype was shared among specimens from Little Barrier Island, northern Taranaki and eastern Taranaki, and was placed between the two star phylogenies and the poorly resolved cluster.
Figure 2. Median-Joining network for Isothraulus abditus based on a 658-bp fragment of the cytochrome c oxidase subunit I (COI) barcoding gene. Coloured points represent different sampling sites; the size of the points is relative to the frequency of the haplotypes. Black points indicate hypothetical haplotypes. Where haplotypes are shared by more than one location, relative haplotype frequency is indicated in the form of pie charts. Sampling location codes are described in .
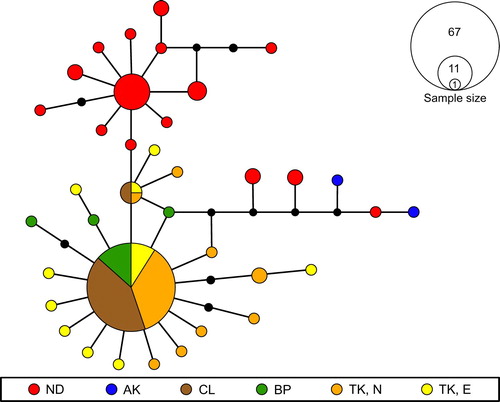
Mean p-distance among groups ranged from 0.3% to 1.3% and within-groups from 0.2% to 0.7% () suggesting populations belong to a single species. Maximum genetic p-distance was 1.8% between individuals from Auckland and Northland, and maximum within-group distances ranged from 0.2% to 1.7%. Analyses using the Kimura two-parameter statistic were identical, or very similar, to those obtained using p-distance so are not reported. AMOVA indicated that overall genetic differentiation in I. abditus was significant (Φst = 0.2799, P < 0.001); 28% of the variance was among populations and 72% within populations. In addition, significant average pairwise differences were found between most populations (), with Northland and Auckland most strongly differentiated from other populations.
Table 5. Estimated evolutionary divergence of Isothraulus abditus based on a 658-bp fragment of the cytochrome c oxidase subunit I (COI) barcoding gene, presented as uncorrected p-distance (%).
Table 6. Pairwise F-statistics (Φst) for Isothraulus abditus based on corrected averages for the 658-bp fragment of the cytochrome c oxidase subunit I (COI) barcoding gene.
Discussion
Before the present study, published distribution records for I. abditus were restricted to four localities (eight streams) in the Waitakere Ranges near Auckland, Great Barrier Island, Coromandel Peninsula, and in Northland. Our nationwide survey combined with existing unpublished records have extended the known distribution by adding 33 further localities (47 streams) in the Northland, Auckland, Coromandel, Waikato, Bay of Plenty, Taranaki and Whanganui regions. The known distribution of I. abditus now extends from Mangonui Forest in Northland, to the Waitotara Forest near Taumatatahi (Whanganui region), and is similar to those of other leptophlebiids such as Arachnocolus phillipsi, Austronella planulata (Towns, 1983), Tepakia caligata, Zephlebia inconspicua Towns, 1983, Zephlebia nebulosa Towns, 1996 and Zephlebia tuberculata Towns, 1996 (Towns and Peters Citation1996; S.R. Pohe, unpubl. data). Its distribution is also similar to the North Island distribution of the siphlaenigmatid mayfly Siphlaenigma janae, although S. janae is also found in the upper South Island (Pohe et al. Citation2018). Although the distribution of I. abditus appears to be fragmented across the upper North Island, a hotspot for the species appears to be the Auckland region where its nymphs have frequently been recorded, especially by State of the Environment monitoring. One reason for this may be that topography in the Auckland region provides suitable habitat for I. abditus owing to its generally short catchments, rolling-hill landscape and forested first- and second-order streams. Further, because of the topography, Auckland’s State of the Environment monitoring programme, by default, has a high proportion of monitoring sites within the habitat of I. abditus. In contrast, headwater streams are not sampled as often in other regions, which target their monitoring towards agricultural inputs and bathing sites lower in their catchments, supplemented by a small number of upper-catchment reference sites.
Our habitat study showed that nymphs of I. abditus occupied the slow-moving waters of pools and backwaters, and were absent from riffles, which have more rapid current velocities. This finding is in accordance with the comments of Towns and Peters (Citation1979) and a later observation by Towns (Citation1987) that they inhabited isolated pools connected by subterranean flow on Great Barrier Island. Towns (Citation1987) also speculated that they might be most common in intermittent streams. Because pools, backwaters and intermittent streams are less commonly sampled in general surveys than faster flowing riffles and streams with permanent flow, the specialised habitat of the nymphs of all sizes is likely to have contributed to the perception of their rarity. We also found strong positive correlations between nymphal abundance and organic cover in both pools and backwaters, indicating that organic matter strongly defines the habitat of I. abditus. However, whether it provides habitat per se, or a food supply, is not known, and requires investigation.
Backwaters were more variable habitats than pools and riffles in terms of temperature, dissolved oxygen, conductivity and pH, presumably reflecting variation in their degree of independence from the main channel. The significant negative correlations between nymphal abundance and dissolved oxygen and pH in backwaters were unexpected, but suggest that nymphs are tolerant of low oxygen concentration and fluctuating pH, at least in the short term. Nymphs of the tropical leptophlebiids Thraulus and Atalophlebia have gill lamellae similar to those of I. abditus; their feather-like fringes increasing the surface area available to extract dissolved oxygen from the warm, still or slow-flowing waters of ponds, lakes and large rivers they inhabit (Gooderham and Tsyrlin Citation2002). The small body size of I. abditus nymphs and their distinctive feather-like gills may therefore aid survival in conditions with low oxygen concentrations, such as those sometimes found in backwater habitats. Interestingly, Tabacchi et al. (Citation1993) found nymphs of Thraulus bellus Eaton, 1881 within the interstitial spaces of a temporary gravel floodplain pond in France, up to 0.8 m below the surface of the bed, and it is possible that as backwater pools dry, I. abditus could make use of the hyporheic zone as a refuge. A number of New Zealand stream invertebrates have been recorded within the interstitial spaces of the hyporheic zone (Adkins and Winterbourn Citation1999; Collier et al. Citation2004) and whether I. abditus makes use of this ecotone is worthy of further investigation.
Our genetic study indicated that I. abditus exhibited moderate haplotype diversity (Hd = 0.7), although mean p-distance was only 0.7%. The haplotype network comprised two main star-shaped clusters (phylogenies), one based on Northland and the other on the central North Island and Little Barrier Island. This separation suggests that populations in Northland may have been isolated and diversified within the region as proposed for two other mayflies, Acanthophlebia cruentata and Siphlaenigma janae (Smith and Collier Citation2001; Pohe et al. Citation2018) and a stonefly, Stenoperla prasina (Newman, 1845) (Winterbourn et al. Citation2017). However, the processes shaping the evolutionary trajectory of Northland samples appears complex, with several of our Northland individuals forming a poorly resolved cluster together with Auckland individuals, and separate from the two main clusters. This may be a consequence of small sample size but could also be indicative of dispersal linking previously isolated populations. More targeted sampling within Auckland and Northland could highlight source–sink dynamics in these regions.
The second star phylogeny incorporated the three central North Island locations sampled, as well as Little Barrier Island. All of these locations shared a common haplotype comprising 55% of all 123 individuals sequenced, suggesting representation of a metapopulation. The presence in this network of individuals from Little Barrier Island suggests the island was likely colonised by dispersing individuals from central North Island rather than Northland, and the absence of unique haplotypes on Little Barrier Island supports the notion that dispersal may have been relatively recent. Little Barrier Island is the emergent part of a large dacite stratovolcano of mid-Pliocene to early Pleistocene age (Lindsay and Moore Citation1995) and has probably had repeated land connections and separations with Great Barrier Island, Coromandel Peninsula and the Northland mainland (Hamilton Citation1961). A phylogeographic study of the skink Oligosoma smithi (Gray, 1845) using the ND2 mitochondrial gene indicated that individuals on islands of the Hauraki Gulf, including Little Barrier, formed a clade with skinks from the Coromandel Peninsula, whereas skinks from Northland formed a separate clade (Hare et al. Citation2008). A southern connection is also indicated by Hamilton’s (Citation1961, p. 15) statement that vegetation on Little Barrier Island ‘resembles that of Coromandel Peninsula rather than that of the adjacent mainland at Cape Rodney [=Northland]’.
Isothraulus abditus was first noted as of potential conservation interest by Collier (Citation1992, Citation1993) due to its known distribution at the time being restricted to two ecological regions (Auckland and Coromandel). Its conservation status was assessed by Molloy and Davis (Citation1992, Citation1994) and classified as ‘Category I’, a grouping of species for which little information was known, but based on existing evidence, were considered threatened. It was subsequently relisted as ‘Range Restricted Data Poor’ (Hitchmough Citation2002; Hitchmough et al. Citation2007) and most recently as ‘At Risk (Declining A(2/1)) Data Poor, Range Restricted’ (Grainger et al. Citation2014). Based on new data we provide in this paper, and by applying the New Zealand Threat Classification System criteria (Townsend et al. Citation2008), I. abditus would be considered as ‘Not Threatened’. However, it is difficult to classify aquatic invertebrate species using current threat classification criteria with confidence. First, difficulty arises because the ‘population size’ criterion for most aquatic invertebrate taxa is unknown, and difficult to estimate. Second, mature stages of some aquatic invertebrates, particularly insects, inhabit entirely different ecosystems, e.g. terrestrial riparian margins, from their juvenile stages, which are usually confined to aquatic habitats, e.g. groundwater, ponds and streams (and often to patches of suitable habitat within them) and hence the ‘area of occupancy’ criterion also falls short. Third, population trends are not known, and no capacity currently exists for population assessments to be conducted. As reported in the present study, the nymph of I. abditus has specific habitat requirements in forested streams and exists as geographically separated populations that show some genetic differentiation within the upper North Island. Consequently, some level of the ‘At Risk’ threat classification is appropriate.
The current fragmented distribution of I. abditus, especially in the northern half of its range, coincides with a part of New Zealand that was subject to intensive logging and forest degradation in the nineteenth century (Diamond Citation1966) and native forest tracts are now highly fragmented. Before logging it seems reasonable to speculate that I. abditus would have been much more widely distributed but due to its weak flying ability, present-day dispersal is likely limited. Effective conservation of the species requires that first- and second-order headwater tributaries be present within tracts of native forest and that they be given adequate protection from future development. National Parks and reserves largely serve this role at present and ideally will be supplemented by further protected areas that connect habitats, not only for the conservation of I. abditus but for freshwater faunas and ecosystems more generally. Furthermore, to conserve genetic variation within I. abditus, suitable forest habitat must be maintained throughout its distributional range, especially in Northland where genetic variability is greatest.
Supplementary Table S1. Distribution records and metadata for Isothraulus abditus as at 12 January 2018. File prepared by Stephen R. Pohe, Pohe Environmental.
Download MS Excel (26.6 KB)Supplementary Table S2. Summary of physico-chemical data obtained in the habitat experiment on the Mangere Stream. CV = coefficient of variation (SD/Mean).
Download PDF (90.2 KB)Supplementary Table S3. Individual haplotype p-distances (below the diagonal) and standard error estimates (above the diagonal in blue) for Isothraulus abditus, based on a 658-bp fragment of the COI barcoding gene.
Download MS Excel (24.7 KB)Supplementary Figure S1. A). Image of the subimago male of Isothraulus abditus. B). Image of the subimago female of Isothraulus abditus.
Download PDF (691.8 KB)Acknowledgements
We thank Matthew Shaw and Terry Hitchings (Canterbury Museum), Phil Sirvid (Museum of New Zealand), Cody Fraser (Otago Museum), Darren Ward (NZAC) and John Early (Auckland Museum) for enabling us to examine the mayfly collections in their care, and/or for providing information on their collection material. We also thank Brian Smith (NIWA Hamilton), Mark Hamer (Waikato Regional Council) and Kevin Collier (University of Waikato) for providing distribution records of Isothraulus abditus. Preliminary fieldwork by Aran Arrieta aided the design of the habitat study. Valuable technical assistance at the University of Canterbury was provided by Alan Woods, Linda Morris, Matt Walters, Nick Etheridge and members of ConSERT. The Pukenui Western Hills Forest Charitable Trust and Nga Ahi Kaa O Pukenui provided permission to conduct research and collect samples in the Pukenui Forest and the Department of Conservation issued a research and specimen collection permit for both the Pukenui Forest habitat study and the national mayfly survey (permit 37682-RES). We also acknowledge Associate Editor Dr Chrissie Painting and two anonymous reviewers for comments that improved the manuscript.
Disclosure statement
No potential conflict of interest was reported by the authors.
ORCID
Stephen R. Pohe http://orcid.org/0000-0002-7357-5185
Michael J. Winterbourn http://orcid.org/0000-0002-3544-2613
Sharyn J. Goldstien http://orcid.org/0000-0002-0619-4016
Olivier J.-P. Ball http://orcid.org/0000-0003-4734-2107
Jon S. Harding http://orcid.org/0000-0002-1070-1594
Additional information
Funding
References
- Adkins SC, Winterbourn MJ. 1999. Vertical distribution and abundance of invertebrates in two New Zealand stream beds: a freeze coring study. Hydrobiologia. 400:55–62. doi: 10.1023/A:1003703028261
- Akaike H. 1973. Information theory and an extension of the maximum likelihood principle. p. 267–281. In: Petrov BN, Csáki F, editors. Proceedings of the 2nd international symposium on information theory, Armenia, USSR, 2–8 September, 1971. Budapest: Akadémiai Kiadó. 451 p.
- Arrieta A. 2010. Riparian impacts on the macroinvertebrate study of the Sandy Bay run-off stream [Unpublished Dip. thesis]. Whangarei: NorthTec. 11 p.
- Arrieta A. 2012. Habitat associations of macroinvertebrates in Pukenui Stream, Northland, New Zealand, with particular reference to Isothraulus abditus (Ephemeroptera: Leptophlebiidae) [Unpublished BAppSci thesis]. Auckland: Unitec. 29 p.
- Bandelt H-J, Forster P, Röhl A. 1999. Median-joining networks for inferring intraspecific phylogenies. Molecular Biology and Evolution. 16:37–48. doi: 10.1093/oxfordjournals.molbev.a026036
- Beet CR. 2016. Assessing the diversity of Antarctic and New Zealand arthropods through DNA barcoding [Unpublished MSc thesis]. Hamilton: University of Waikato. 106 p.
- Benson DA, Cavanaugh M, Clark K, Karsch-Mizrachi I, Lipman DJ, Ostell J, Sayers EW. 2013. Genbank. Nucleic Acids Research. 41:D36–D42. doi: 10.1093/nar/gks1195
- Clement M, Posada D, Crandall KA. 2000. TCS: a computer program to estimate gene genealogies. Molecular Ecology. 9:1657–1659. doi: 10.1046/j.1365-294x.2000.01020.x
- Collier KJ. 1992. Freshwater macroinvertebrates of potential conservation interest. Science & Research Series 50. Wellington: Department of Conservation. 45 p.
- Collier KJ. 1993. Review of the status, distribution, and conservation of freshwater invertebrates in New Zealand. New Zealand Journal of Marine and Freshwater Research. 27:339–356. doi: 10.1080/00288330.1993.9516574
- Collier KJ. 1995. Environmental factors affecting the taxonomic composition of aquatic macroinvertebrate communities in lowland waterways of Northland, New Zealand. New Zealand Journal of Marine and Freshwater Research. 29:453–465. doi: 10.1080/00288330.1995.9516679
- Collier KJ, Wright-Stow AE, Smith BJ. 2004. Trophic basis of production for a mayfly in a North Island, New Zealand, forest stream: contributions of benthic versus hyporheic habitats and implications for restoration. New Zealand Journal of Marine and Freshwater Research. 38:301–314. doi: 10.1080/00288330.2004.9517239
- Collins RA, Boykin LM, Cruickshank RH, Armstrong KF. 2012. Barcoding's next top model: an evaluation of nucleotide substitution models for specimen identification. Methods in Ecology and Evolution. 3:457–465. doi: 10.1111/j.2041-210X.2011.00176.x
- Cook TL. 2002. The diversity and community structure of macroinvertebrates in native forest streams of Northland: a comparison with Hawkes Bay forest streams [Unpublished BSc Honours thesis]. Palmerston North: Massey University. 37 p.
- Crosby TK, Dugdale JS, Watt JC. 1998. Area codes for recording specimen localities in the New Zealand subregion. New Zealand Journal of Zoology. 25:175–183. doi: 10.1080/03014223.1998.9518148
- Diamond JT. 1966. Once the wilderness. 2nd ed. Auckland: V. H. Wilkinson. 224 p.
- Excoffier L, Lischer HEL. 2010. Arlequin suite ver 3.5: a new series of programs to perform population genetics analyses under Linux and Windows. Molecular Ecology Resources. 10:564–567. doi: 10.1111/j.1755-0998.2010.02847.x
- Folmer O, Black M, Hoeh W, Lutz R, Vrijenhoek R. 1994. DNA primers for amplification of mitochondrial cytochrome c oxidase subunit I from diverse metazoan invertebrates. Molecular Marine Biology and Biotechnology. 3:294–299.
- Gooderham J, Tsyrlin E. 2002. The waterbug book: a guide to the freshwater macroinvertebrates of temperate Australia. Victoria: CSIRO Publishing. 232 p.
- Grainger N, Collier K, Hitchmough R, Harding J, Smith B, Sutherland D. 2014. Conservation status of New Zealand freshwater invertebrates, 2013. New Zealand Threat Classification Series 8. Wellington: Department of Conservation. 28 p.
- Gray DP, Harding JS. 2012. Acid mine drainage index (AMDI): a benthic invertebrate biotic index for assessing coal mining impacts in New Zealand streams. New Zealand Journal of Marine and Freshwater Research. 46:335–352. doi: 10.1080/00288330.2012.663764
- Hall TA. 1999. Bioedit: a user-friendly biological sequence alignment editor and analysis program for Windows 95/98/NT. Nucleic Acids Symposium Series. 41:95–98.
- Hamilton, WM. 1961. Little Barrier Island (Hauturu). 2nd ed. Bulletin 137. Wellington: New Zeland Department of Scientific and Industrial Research. 180 p.
- Hare KM, Daugherty CH, Chapple DG. 2008. Comparative phylogeography of three skink species (Oligosoma moco, O. smithi, O. suteri; Reptilia: Scincidae) in northeastern New Zealand. Molecular Phylogenetics and Evolution. 46:303–315. doi: 10.1016/j.ympev.2007.08.012
- Hebert PDN, Cywinska A, Ball SL, deWaard, JR. 2003. Biological identifications through DNA barcodes. Proceedings of the Royal Society B: Biological Sciences. 270:313–321. doi: 10.1098/rspb.2002.2218
- Hitchings TR. 2001. The Canterbury Museum mayfly collection and database (Insecta: Ephemeroptera). Records of the Canterbury Museum. 15:11–32.
- Hitchings TR, Hitchings TR, Shaw MD. 2015. A revision of the distribution maps and database of New Zealand mayflies (Ephemeroptera) at Canterbury Museum. Records of the Canterbury Museum. 29:5–34.
- Hitchmough R. 2002. New Zealand threat classification system lists—2002. Threatened Species Occasional Publication 23. Wellington: Department of Conservation. 210 p.
- Hitchmough, R, Bull, L, Cromarty, P. 2007. New Zealand threat classification system lists—2005. Wellington: Department of Conservation. 194 p.
- Hogg ID, Willmann-Huerner P, Stevens MI. 2002. Population genetic structures of two New Zealand stream insects: Archichauliodes diversus (Megaloptera) and Coloburiscus humeralis (Ephemeroptera). New Zealand Journal of Marine and Freshwater Research. 36:491–501. doi: 10.1080/00288330.2002.9517104
- Hynes HBN. 1970. The ecology of running waters. Toronto: University of Toronto Press. 555 p.
- Kimura M. 1980. A simple method for estimating evolutionary rates of base substitutions through comparative studies of nucleotide sequences. Journal of Molecular Evolution. 16:111–120. doi: 10.1007/BF01731581
- Kumar S, Stecher G, Tamura K. 2016. MEGA7: molecular evolutionary genetics analysis version 7.0 for bigger datasets. Molecular Biology and Evolution. 33:1870–1874. doi: 10.1093/molbev/msw054
- Leigh JW, Bryant D. 2015. POPART: full-feature software for haplotype network construction. Methods in Ecology and Evolution. 6:1110–1116. doi: 10.1111/2041-210X.12410
- Lindsay JM, Moore P. 1995. Geological features of Little Barrier Island, Hauraki Gulf. Tane. 35:25–38.
- McEwen WM. 1987. Ecological regions and districts of New Zealand (third revised ed. in four maps). New Zealand Biological Resources Centre, Publication No. 5. Wellington: Department of Conservation.
- MFE. 2015. Ministry for the environment national indicator data for river condition in New Zealand. Collected by Regional Councils and the National Institute of Water and Atmospheric Research (NIWA), collated and processed by NIWA and protected by copyright owned by the Ministry for the Environment on behalf of the Crown. Accessed 9th July 2015.
- Molloy J, Davis A. 1992. Setting priorities for the conservation of New Zealand's threatened plants and animals. Wellington: Department of Conservation. 44 p.
- Molloy J, Davis A. 1994. Setting priorities for the conservation of New Zealand's threatened plants and animals. Second ed. Wellington: Department of Conservation. 64 p.
- Nei M. 1987. Molecular evolutionary genetics. New York: Columbia University Press. 512 p.
- O'Donnell BC, Jockusch EL. 2008. Phylogenetic relationships of leptophlebiid mayflies as inferred by histone H3 and 28S ribosomal DNA. Systematic Entomology. 33:651–667. doi: 10.1111/j.1365-3113.2008.00434.x
- Parkyn S, Phillips N, Smith B. 2006. Aquatic invertebrate biodiversity and spatial characterisation of non-perennial streams in native forest in the Waikato Region. Environmental Waikato Technical Report 2006/38. 27 p.
- Pohe SR. 2008. Aquatic invertebrate fauna of Matapouri, Northland [Unpublished MSc thesis]. Auckland: Auckland University of Technology. 114 p.
- Pohe SR, Winterbourn MJ, Goldstien SJ, Harding JS. 2018. Distribution, body size, genetic structure and conservation of Siphlaenigma janae (Insecta: Ephemeroptera). New Zealand Journal of Zoology. 45:154–170. doi: 10.1080/03014223.2017.1405823
- Pohe SR, Winterbourn MJ, Harding JS. Forthcoming 2018. Comparison of fluorescent lights with differing spectral properties on catches of adult aquatic and terrestrial insects. New Zealand Entomologist. doi: 10.1080/00779962.2017.1398704
- Quinn JM, Halliday J. 1998. Effects of harvesting Whangapoua Forest on stream habitat and invertebrate communities: report on 1998 surveys. NIWA Client Report ERN90201/2 prepared for Ernslaw One Ltd. 33 p.
- Quinn JM, Parkyn SM. 1996. Effects of harvesting Whangapoua Forest on stream habitat and invertebrate communities: report on 1995 surveys. NIWA Consultancy Report ERN60201/2 prepared for Ernslaw One Ltd. 29 p.
- Smith PJ, Collier KJ. 2001. Allozyme diversity and population genetic structure of the caddisfly Orthopsyche fimbriata and the mayfly Acanthophlebia cruentata in New Zealand streams. Freshwater Biology. 46:795–805. doi: 10.1046/j.1365-2427.2001.00719.x
- Smith PJ, McVeagh SM, Collier KJ. 2006. Genetic diversity and historical population structure in the New Zealand mayfly Acanthophlebia cruentata. Freshwater Biology. 51:12–24. doi: 10.1111/j.1365-2427.2005.01462.x
- Stark JD. 1993. Performance of the macroinvertebrate community index: effects of sampling method, sample replication, water depth, current velocity, and substratum on index values. New Zealand Journal of Marine and Freshwater Research. 27:463–478. doi: 10.1080/00288330.1993.9516588
- Tabacchi E, Décamps H, Thomas A. 1993. Substrate interstices as a habitat for larval Thraulus bellus (Ephemeroptera) in a temporary floodplain pond. Freshwater Biology. 29:429–439. doi: 10.1111/j.1365-2427.1993.tb00777.x
- Towns DR. 1976. Dynamics of benthic invertebrate communities in a northern New Zealand Kauri forest stream ecosystem [Unpublished PhD thesis]. Auckland: The University of Auckland. 150 p.
- Towns DR. 1978. Some little-known benthic insect taxa from a northern New Zealand river and its tributaries. New Zealand Entomologist. 6:409–419. doi: 10.1080/00779962.1978.9722306
- Towns DR. 1987. The mayflies (Ephemeroptera) of Great Barrier Island, New Zealand: macro- and micro-distributional comparisons. Journal of the Royal Society of New Zealand. 17:349–361. doi: 10.1080/03036758.1987.10426476
- Towns DR, Peters WL. 1979. New genera and species of Leptophlebiidae (Ephemeroptera) from New Zealand. New Zealand Journal of Zoology. 6:439–452. doi: 10.1080/03014223.1979.10428383
- Towns DR, Peters WL. 1980. Phylogenetic relationships of the Leptophlebiidae of New Zealand (Ephemeroptera). p. 57–69. In: Flannagan JF, Marshall KE, editors. Advances in Ephemeroptera biology. Proceedings of the 3rd International Conference on Ephemeroptera, Winnipeg, Canada, 1979. New York: Plenum. 552 p.
- Towns DR, Peters WL. 1996. Leptophlebiidae (Insecta: Ephemeroptera). Fauna of New Zealand 36. Lincoln: Manaaki Whenua Press. 143 p.
- Townsend AJ, de Lange PJ, Duffy CAJ, Miskelly CM, Molloy J, Norton DA. 2008. New Zealand threat classification system manual. Wellington: Department of Conservation. 35 p.
- Winterbourn MJ, Gregson KLD, Dolphin CH. 2006. Guide to the aquatic insects of New Zealand. 4th ed. Bulletin of the Entomological Society of New Zealand 14. 108 p.
- Winterbourn MJ, Pohe SR, Goldstien SJ. 2017. Genetic and phenotypic variability in Stenoperla prasina (Newman, 1845) (Plecoptera: Eustheniidae) in relation to latitude and altitude in New Zealand. Aquatic Insects. 38:49–65. doi: 10.1080/01650424.2017.1302091
Appendix. Description of male and female subimagos of Isothraulus abditus
Introduction
Towns and Peters (Citation1979) described the male imago, female imago and nymph of Isothraulus abditus but not the subimago, which they stated was unknown, despite a female subimago being listed as a paratype. The adult and nymph were redescribed in their subsequent monograph on the New Zealand Leptophlebiidae (Towns and Peters Citation1996). We have now obtained male and female subimagos and take the opportunity to provide descriptions of them here. Descriptions and specimen dimensions are based on all available material: 23 male and four female subimagos. Body size ranges are provided with mean values in parentheses. Subimagos were confidently associated with adult material based on morphological characters, but were also associated by DNA analysis with nymphal and adult material. Collection localities are identified using the abbreviated codes of Crosby et al. (Citation1998) and latitude and longitude coordinates use the New Zealand Geodetic Datum 2000 (version 20160701). All specimens are held in the personal collection of Stephen R. Pohe.
Description
Isothraulus abditus Towns and Peters, Citation1979
Dimensions (mm). Male subimago: length of body 6.5–8.9 (7.6); forewings 7.5–9.8 (8.5). Female subimago: length of body 8.2–11.0 (9.5); forewings 9.1–12.1 (10.6).
Male subimago (in ethanol; see Figure S1A for image). Head grey-white with prominent conical ocelli; antennae filiform, shorter than width of head; eyes fused in midline, upper portion reddish-brown, lower portion black.
Thorax grey-white dorsally and ventrally; metanotum and posterior third of mesonotum each with a pair of grey longitudinal bands medially; mesopleura with a prominent ‘bar’ of dark pigment extending forward from a more diffuse patch of dark pigment on the posterior mesopleura and metapleura; metasternum with a transverse band of dark pigment. Wings translucent white; fringes of hairs on their posterior margins. Forewings with pale longitudinal veins; crossveins brown, those in cells C and Sc and near the middle of R1 surrounded by narrow clouds of dark pigment; diffuse patches of dark pigment at the fork of MA near the middle of the wing, and extending from the thickest crossveins in R1. Hindwings translucent; fringes of hairs on their posterior margins; venation pale; Sc about two-thirds length of wing. Legs grey-white; femora faintly yellowish.
Abdomen predominantly white dorsally and ventrally; ganglia not visible; terga 1–8 each with a narrow transverse dark band on hind margin; terga 8 and 9 dark grey, lighter in midline; tergum 10 and external genitalia whitish; dark articulation points of nymphal gills prominent laterally on segments 1–7. Caudal filaments pale, as long as body.
Female subimago (in ethanol; see Figure S1B for image). As in male, except as follows. Head grey-white washed with black adjacent to eyes, around bases of ocelli and in the dorsal midline; eyes black, separated by approximately their own width. Abdomen darker than in male, yellowish-grey washed with brown-grey pigment medially giving the appearance of large paired pale patches laterally; terga 8 and 9 darker. Pairs of small yellowish maculae (spots) present anteriorly on terga 3 to 7; abdominal sterna paler than terga; ganglia not visible; sternum 7 with an egg guide reaching about the middle of sternum 8; sternum 9 rounded posteriorly with no terminal notch.
Material examined
ND. 1♂ subimago, Tapapa Stm, Raetea Forest, Mangamuka, 35.1934 S; 173.4792 E, light trap, 10 January 2014, SR Pohe [POHE10120]. 1♂ subimago, Punaruku Stm, Russell Forest, Punaruku, 35.3976 S; 174.3087 E, light trap, 16 December 2013, SR Pohe [POHE10844]. 3♂ and 2♀ subimagos, Kauri Stm [informal name], Pukenui Forest, Whangarei, 35.7046 S; 174.2616 E, light trap, 23–26 October 2013, SR Pohe [POHE00637, POHE01062, POHE01074, POHE01050, POHE01155]. 2♂ and 1♀ subimagos, Kauri Stm [informal name], Pukenui Forest, Whangarei, 35.7046 S; 174.2616 E, light trap, 22 December 2013, SR Pohe [POHE01746, POHE01784, POHE01783]. 3♂ and 1♀ subimagos, Pekapeka Stm [informal name], Pukenui Forest, Whangarei, 35.7141 S; 174.2508 E, light trap, 25–26 October 2013, SR Pohe [POHE01153, POHE01196, POHE01228, POHE01247]. 1♂ subimago, Mangere Stm, Pukenui Forest, Whangarei, 35.7122 S; 174.2461 E, light trap, 23 October 2013, SR Pohe [POHE00719]. AK. 1♂ subimago, Pohuehue Stm [informal name], Pohuehue Reserve nr Warkworth, 36.4535 S; 174.6515 E, light trap, 11 December 2013, SR Pohe [POHE03101]. BP. 2♂ subimagos, Ohaupara Stm, Mangorewa Ecological Area, 37.9609 S; 176.1706 E, light trap, 01 February 2014, SR Pohe [POHE09931, POHE09934]. TK. 6♂ subimagos, Kotare Stm, Hutiwai Forest nr Kotare, 38.8631 S; 174.7784 E, light trap, 05 February 2014, SR Pohe [POHE12113, POHE12149, POHE12180, POHE12182, POHE12185, POHE12191]. 2♂ subimagos, tributary of Waitara R., Pouiatoa Forest nr Purangi, 39.1234 S; 174.5119 E, light trap, 06 February 2014, SR Pohe [POHE06509, POHE06569]. RI. 1♂ subimago, tributary of Waitotara R., Waitotara Forest nr Taumatatahi, 39.5432 S; 174.7624 E, light trap, 10 February 2014, SR Pohe [POHE03347].
Remarks. The subimago of Isothraulus abditus can be most easily distinguished from that of its closest relative Tepakia caligata by the characteristic forewing markings (as in the imago), the absence of dark brown tarsi, and the white rather than dark brown abdomen of the male. Although the abdomen of female I. abditus is darker than in the male it differs from that of T. caligata in having very prominent dark bands at the posterior margins of terga 1–8, prominent gill articulations on abdominal segments 1–7, and pairs of yellowish maculae anteriorly on terga 3–7. The egg guide is narrow and tube-like whereas that of T. caligata is shorter and broader (Towns and Peters Citation1996). Note however that the egg guide of the subimagos we examined were shorter than that of the imago illustrated by Towns and Peters (Citation1996). This may indicate that the egg guide lengthens at the final moult, since egg guides of adults collected at the same site extended to the posterior end of abdominal segment 8. Although colour patterns are useful for identification of both imagos and subimagos of I. abditus they are difficult to define precisely, and apparent colours are influenced by condition of preservation, microscope lighting and the nature of the background against which they are examined.