ABSTRACT
Despite being a large, relatively abundant predator, the distribution and seasonal occurrence of the broadnose sevengill shark, Notorynchus cepedianus, in New Zealand is poorly understood. During 71 sampling trips conducted from July 2013 to May 2015, sharks were attracted to coastal sampling sites in southern New Zealand at Ōtākou/Otago Harbour and Te Whaka ā Te Wera/Paterson Inlet, Stewart Island, using chum. Using a logistic regression model, water temperature was identified as a key predictor of encountering sevengill sharks. In addition, location, cloud cover and sea state were also identified as influential predictors. At Ōtākou, a clear seasonal pattern of sevengill shark sightings emerged. Sharks were detected on 86% of survey trips in summer, whilst no sharks were detected in winter or spring. At Te Whaka ā Te Wera, sharks were sighted throughout all seasons, but a decline in shark encounters occurred during winter. This study represents the first systematic data on seasonal habitat use by sevengill sharks in New Zealand.
Introduction
Large sharks (Chondrichthyes: Elasmobranchii) often utilise large spatial ranges, potentially influencing the structure and function of multiple ecosystems (Myers et al. Citation2007; Williams et al. Citation2012). For this reason, an understanding of spatio-temporal habitat use by large sharks is important for landscape-scale assessments of ecosystem function (Dill et al. Citation2003; Barnett et al. Citation2011). Such assessments can contribute to effective fisheries management and conservation strategies, and thus to the conservation of shark species and their habitats (Bonfil Citation1997; Baum et al. Citation2003; Ferretti et al. Citation2010).
The broadnose sevengill shark Notorynchus cepedianus (Peron, 1807) (Chondrichthyes: Hexanchidae) is distributed throughout temperate coastal regions worldwide (Barnett et al. Citation2012; Larson et al. Citation2015). It is commonly found in shallow (< 200 m) coastal waters, including bays, estuaries and rocky reefs (Barnett et al. Citation2012; Williams et al. Citation2012). Sevengill sharks display seasonal use of coastal habitats, which they may utilise as nursery areas, for feeding or for mating (Lucifora et al. Citation2005; Williams et al. Citation2012).
Studies conducted off the coasts of Patagonia (Lucifora et al. Citation2005), western USA (Ebert Citation1989; Williams et al. Citation2012) and Tasmania, Australia (Barnett et al. Citation2010a; Abrantes & Barnett Citation2011; Stehfest et al. Citation2014) demonstrated a marked increase in abundance of sevengill sharks in coastal embayments and nearshore areas during spring and summer, followed by near absences in winter. Previous studies have identified water temperature as a key environmental cue responsible for initiating these seasonal migrations (Williams et al. Citation2012; Stehfest et al. Citation2014). On Patagonian (Lucifora et al. Citation2005) and Californian (Ebert Citation1989) coasts, mating and parturition are considered the main drivers of migration in sevengill sharks. In contrast, off Washington (Williams et al. Citation2012) and Tasmania (Barnett et al. Citation2010a, Citation2010b), migration is thought to be mediated by variations in prey abundance; sevengill sharks enter coastal areas seeking seasonally abundant target prey species (Barnett & Semmens Citation2012). In addition, it has been suggested that sevengill sharks may frequent warm shallow embayments to enhance physiological performance relating to elevated core body temperatures (Williams et al. Citation2012). In shark species, warmer environments have been suggested to increase breeding and digestion efficiency, while minimising energy expenditure (Hight & Lowe Citation2007).
Anecdotally, sevengill sharks are known to occur in bays, estuaries and coastal areas of New Zealand, particularly during the warmer summer months. Sevengill sharks in the northern part of the country are thought to be relatively abundant (Cox & Francis Citation1997), but to date there have been no systematic studies of the species anywhere in New Zealand. The current lack of biological, ecological and fisheries data on sevengill sharks leaves many questions unanswered concerning their population and conservation status, and the effects of their presence in coastal areas. We investigated the spatio-temporal occurrence of sevengill sharks in two harbours in southern New Zealand. Studies of the temporal and spatial distribution of sevengill shark populations are important if we are to understand their influence within these habitats.
Materials and methods
Research was carried out at two locations in southern New Zealand; Ōtākou (Otago Harbour), Dunedin and Te Whaka ā Te Wera (Paterson Inlet) on Rakiura (Stewart Island) (). Two sampling sites were chosen in Ōtākou (Harington Point and Saint Leonards) and three in Te Whaka ā Te Wera (Southwest Arm, Sawdust Bay and Ackers Point). Sampling sites were chosen based on anecdotal evidence of local sevengill shark populations, accessibility and the need to avoid impacts on other water users.
Figure 1. Map displaying the two study locations in southern New Zealand. Insets show the two study sites in Ōtākou (A) and three study sites in Te Whaka ā Te Wera (B).
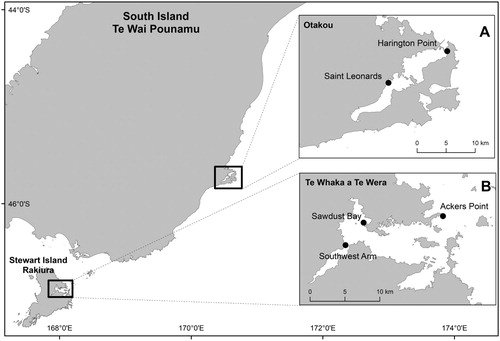
Survey trips took place between July 2013 and May 2015, approximately every 3 weeks at Ōtākou, and on approximately five survey trips per month during 11 months at Te Whaka ā Te Wera. Uniform sampling effort was attempted across all sites at the two locations, but the choice of site was ultimately dictated by weather and sea conditions on the day. Sampling was only carried out in swell heights less than 0.25 m. At Te Whaka ā Te Wera sampling was conducted from a 6.3 m rigid hulled inflatable boat anchored near the shore in 3–6 m of water while, due to ease of access, sampling at Ōtākou was conducted from the harbour shore. On each sampling occasion the following variables were recorded on arrival at the sampling site: water temperature (measured to the nearest 0.5 °C); water flow (measured in cm/s with a drogue); sea state (estimated on the Beaufort scale); and estimated percentage cloud cover. Survey trips took place at dawn and twilight, periods associated with peak sevengill shark activity, as observed in Tasmania and South Africa (Ebert Citation1991; Barnett et al. Citation2010c).
Sharks were attracted by pouring chum into the water (Soldo & Peirce Citation2005; Bruce et al. Citation2006). The chum used was a mixture of tuna oil and minced fish, including either blue cod (Parapercis colias) or sole (Peltorhamphus novaezeelandiae). To entice a shark to ascend directly beside the research vessel or sample site, the head of a blue cod was tied to the end of a rope and used as a lure. Information specific to each individual could then be collected. For each shark sighting, the time of arrival and duration of visibility in the vicinity of the sampling site was recorded. Individual sharks were identified using unique spots, scars or fin nicks. Sevengill sharks are well marked on their dorsal surface, with a pattern of light and dark spots unique to each individual. An attempt was made to photograph these features ().
Figure 2. Examples of dorsal patterns used to distinguish between individual sevengill sharks at Te Whaka ā Te Wera.
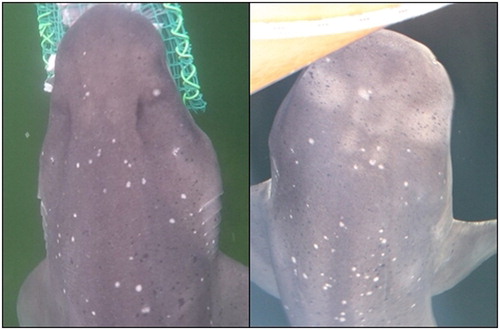
Statistical analysis
No sevengill sharks were sighted at Harington Point, Ōtākou (14 sampling trips) or Ackers Point, Te Whaka ā Te Wera (six sampling trips) and these sites were omitted from the statistical analysis. Sightings from Sawdust Bay and Southwest Arm were pooled, resulting in data from two locations: Ōtākou and Te Whaka ā Te Wera. To compare the seasonal occurrence and mean number of sevengill sharks sighted in this study, the proportion of survey trips with sharks present was calculated, along with the mean number of sharks present per trip.
The effect of abiotic variables and sighting conditions on the probability of encountering sevengill sharks was investigated by constructing a suite of logistic generalised linear models (GLMs). GLMs are extensions of ordinary linear regressions, allowing for non-normal error distributions in the data, using a function to link predictors to a response variable (McCullagh & Nelder Citation1983). In the case of a binary response, such as presence or absence of the species of interest, a logistic link function can be used (Guisan et al. Citation2002).
As the number of sharks attracted to the surface bait was an unknown fraction of all sharks present, the response variable was sighting (1) or not sighting (0) a sevengill shark on each sampling trip, standardised to within 2 hours of when chumming began. Water temperature, water flow, sea state and cloud cover were included as continuous explanatory variables in the logistic models. The following categorical variables were included: ‘Location’; ‘Season’, (spring [September–November], summer [December–February], autumn [March–May], winter [June–August]); and ‘Chum Type’ (blue cod, sole or mixed fish). These seven predictors were considered to potentially influence the likelihood of encountering a sevengill shark. All possible combinations of the seven explanatory variables were used to construct a set of competing models. Akaike's Information Criterion (AIC) was then used to select the best explanatory model from the suite of constructed models (Burnham & Anderson Citation2002). AIC identifies the optimal model among a selection of competing models, accounting for best fit and model parsimony (Bozdogan Citation1987). Using AIC, Akaike weights can then be calculated, which can be interpreted as conditional probabilities for each model (Wagenmakers & Farrell Citation2004). As the number of survey occasions was small in relation to the number of predictor variables, AIC values were corrected for small sample size (AICc) according to Gill et al. (Citation2011). The appropriateness of the best fitting model was determined by examining diagnostic plots. The model residuals were approximately normally distributed, and the Cook's distance for all data points was less than 0.5, indicating none had excessive influence.
All analyses were conducted using the R programming language run under RStudio v0.98.1091 (RStudio: Integrated Development for R. RStudio, Inc. http://www.rstudio.com/).
Results
A total of 71 survey trips were conducted between July 2013 and May 2015 (). Sevengill sharks were encountered on 53% of trips. At Ōtākou, sevengill sharks were detected on 86% of survey trips in summer (mean = 2.14 sharks per trip), while no sharks were detected in winter or spring, despite similar effort across seasons ().
Table 1. Summary of sampling effort, percentage of trips with at least one sevengill shark detected and mean number of sharks sighted per trip at Ōtākou and Te Whaka ā Te Wera, New Zealand between July 2013 and May 2015.
At Te Whaka ā Te Wera, sevengill sharks were sighted in all seasons, over a total of 45 survey trips (). Sharks were encountered on between 71% and 79% of trips between spring and autumn, but only on 33% of trips in winter. The mean number of sevengill sharks sighted per survey trip at Te Whaka ā Te Wera ranged from 2.36 in autumn to 3.29 in spring ().
Location, water temperature, cloud cover and sea state were included in the top four models (), and the best-fit model, indicated by the lowest AICc score, included only these four predictors. Model weights (wi) suggest that model 1 was 28 times more likely than model 2 (). The low weights assigned to the remaining models suggest that season, water flow and chum type were not important predictors of encountering sevengill sharks.
Table 2. Model selection results.
The individual effects of each explanatory variable in model 1 are displayed in . Location was a significant predictor of encountering sevengill sharks; the likelihood of sighting a shark at Te Whaka ā Te Wera being 12.8 times greater than at Ōtākou (). Water temperature and cloud cover had significant positive effects, while sea state had a significant negative effect. The magnitudes of the effects are illustrated by the odds ratios. For example, with every unit increase in water temperature, the likelihood of sighting a shark was 1.67 times greater.
Table 3. Effects of the explanatory variables in the best model (model 1).
Further details about the habitat preferences of sevengill sharks are revealed by examining plots of the raw data (). The preference for warmer water was evident in both locations, although more pronounced at Ōtākou where a wider range of temperatures was experienced (A). At Ōtākou, water temperatures ranged from as low as 4 °C during winter sampling to 18 °C in summer, with no sharks sighted below 12 °C. In contrast, water temperatures at Te Whaka ā Te Wera ranged from 7 °C to 14.5 °C during sampling, with sharks sighted throughout the range of temperatures. The effect of cloud cover was more pronounced at Te Whaka ā Te Wera, where encounters mostly occurred with fully overcast skies (B). A wider range of sea states was experienced at Ōtākou, although in both locations sharks were generally encountered at sea states of Beaufort 3 or less (C).
Discussion
Despite being recognised as one of the most important apex predators in temperate coastal communities (Stehfest et al. Citation2014), no studies have investigated the seasonal occurrence of sevengill sharks in New Zealand. The present study begins to address this knowledge gap, providing insight into the distribution of sevengill sharks, and factors affecting the likelihood of encountering them, in southern New Zealand.
The data on seasonal variation in occurrence from Ōtākou and Te Whaka ā Te Wera suggest that, in southern New Zealand, sevengill sharks utilise coastal embayments, or at least specific areas within embayments, at distinct times of the year. The seasonal pattern at Ōtākou, where sharks were sighted in spring and summer, but not in autumn and winter, is similar to distribution patterns displayed by sevengill sharks in Patagonia (Lucifora et al. Citation2005), western USA (Ebert Citation1989; Williams et al. Citation2012) and Tasmania (Barnett et al. Citation2010a; Abrantes & Barnett Citation2011; Stehfest et al. Citation2014). Despite the year-round presence of sevengill sharks at Te Whaka ā Te Wera, a lower sighting rate was observed in winter, consistent with this pattern.
These findings suggest that, at specific times of the year, sevengill sharks in New Zealand disperse from coastal bays. Large scale movements or migrations of apex predators, such as sevengill sharks (Abrantes & Barnett Citation2011) have been attributed to a change in prey availability, reproductive opportunities or environmental conditions (Kuhn et al. Citation2009; Knip et al. Citation2010; Speed et al. Citation2010). Assessing these distribution patterns and their drivers contributes to further understanding the ecology of the species, and may also be useful for predicting responses to anthropogenic impacts (Stehfest et al. Citation2014).
Species-distribution models (SDMs) are numerical tools that combine observations of species occurrence or abundance with measurements of environmental parameters (Elith & Leathwick Citation2009). These models are used to interpret the role of environmental conditions in driving population distributions (Hacohen-Domené et al. Citation2015), and may be useful in predicting the likelihood of species occurrence in areas where biological knowledge is limited (Robertson et al. Citation2003). SDMs can be constructed using a variety of methods, ranging from relatively simple regression models to complex non-linear models (e.g. Guisan et al. Citation2002; Gey & Nedelec Citation2005). SDMs have been applied in many distribution analyses of migratory shark species, and generally incorporate measurements of hydrographic variables with sighting or occurrence data (e.g. Dambach & Rödder Citation2011; McCallister et al. Citation2013; Drymon et al. Citation2014).
Combining the outcomes of seasonal occurrence with the species-distribution modelling enabled identification of key factors associated with encountering sevengill sharks in southern New Zealand. In the top-ranked SDM, water temperature was identified as an important predictor of encountering a sevengill shark. Throughout the year water temperature varied at Ōtākou and Te Whaka ā Te Wera, contributing to the likelihood of sighting a shark and explaining the observed seasonal trends in the data. The relatively narrow range of water temperatures at Te Whaka ā Te Wera, compared to the broader range observed at Ōtākou, may explain the disparity in shark encounters between these locations during different times of the year.
Water temperature and season have been previously recognised in a number of studies as influencing the distribution of shark species (Heithaus Citation2001; Barnett et al. Citation2010a). Although water temperature and season are likely to be correlated, temperatures were quite different at Ōtākou and Te Whaka ā Te Wera, so both explanatory variables were included. Environmental factors such as water flow, sea state and cloud cover may affect sensory reception or detection of prey (Ebert Citation1991; Hammerschlag et al. Citation2006; Robbins Citation2007). The type of prey available and the location of the habitat may also influence the occurrence of shark species (Knip et al. Citation2010).
Acoustic and satellite tagging suggests that sevengill sharks migrate away from coastal sites when ambient temperature falls below 11 °C (Williams et al. Citation2012; Stehfest et al. Citation2014). Van Dykhuizen & Mollet (Citation1992) also reported the highest abundance of sevengill sharks in water temperatures between 12 °C to 18 °C. Similarly, there may be an optimal range of water temperatures for sevengill sharks in southern New Zealand. At Te Whaka ā Te Wera the majority of sharks were sighted at water temperatures between 11 °C and 14 °C, although there were sightings at temperatures as low as 7 °C. At Ōtākou sharks were only sighted between 13.5 °C and 17 °C.
On Patagonian (Lucifora et al. Citation2005) and Californian (Ebert Citation1989) coasts, researchers observed the seasonal use of embayments by sevengill sharks, for the purpose of mating and parturition. In contrast, the seasonal occurrence of sevengill sharks in Willapa Bay, Washington and both Derwent and Norfolk Bays, Tasmania was attributed to the exploitation of seasonally abundant prey species such as gummy sharks (Mustelus antarcticus) and spiny dogfish (Squalus acanthias) (Barnett et al. Citation2010b, Citation2010c; Williams et al. Citation2012). This information suggests that sevengill sharks use different habitats to carry out specific biological processes, and migrate between locations to perform them.
Although the reason for the increased occurrence of sevengill sharks in coastal habitats in southern New Zealand is not known, this may be a reflection of prey distribution. At Ōtākou, a number of fish species seasonally migrate into the harbour, using shallow, protected areas as nurseries and feeding grounds (Boyd Citation2008; James et al. Citation2010). Populations of known sevengill shark prey species, such as spiny dogfish (S. acanthias) (Barnett et al. Citation2010d) and elephant fish (Callorhinchus milii) (Crespi-Abril et al. Citation2003), occur at Ōtākou (Boyd Citation2008), whilst other small shark species such as rig (Mustelus lenticulatus) and school shark (Galeorhinus galeus) occur on a seasonal basis for the purpose of parturition (Boyd Citation2008). Other potential prey species, including speckled sole (Peltorhamphus latus) sand flounder (Rhombosolea plebeia) and greenback flounder (R. tapirina) demonstrate peak abundances of juveniles during summer at Ōtākou (Roper & Jillett Citation1981). At Te Whaka ā Te Wera, the narrower seasonal temperature range may mean that seasonal changes in prey abundance are less pronounced. For example, blue cod (P. colias), one of the most abundant fish species in the inlet, are present in high numbers year round (James et al. Citation2004). A more consistent supply of prey could explain why sharks are present throughout the year. As P. colias are endemic to New Zealand (Pankhurst & Conroy Citation1987), no literature has yet described the species as prey for sevengill sharks. However, their consumption is not unlikely given the broad diet of sevengill sharks (Ebert Citation2002). Furthermore, the consistent occurrence of sevengill sharks at Te Whaka ā Te Wera may be influenced by the presence of king salmon or ‘chinook’ (Oncorhynchus tshawytscha) farms, located at Big Glory Bay; a divaricating arm within the inlet. There are anecdotal reports of sharks aggregating around the two aquaculture facilities throughout the year. The use of the two habitats may also be influenced by their history of modification, which could affect prey availability. For example, Te Whaka ā Te Wera is a largely unmodified ecosystem, with much of the catchment in native forest and relatively intact benthic communities (Department of Conservation Citation2012). In contrast, Ōtākou is much more impacted by human activity, including fisheries and shipping (Smith et al. Citation2010).
Diet studies may provide a useful technique to explore such predator–prey relationships in southern New Zealand. In southeast Tasmania, stomach flushing and stable isotope sampling determined that local sevengill shark populations exploit seasonally abundant prey species (Barnett et al. Citation2010b; Abrantes & Barnett Citation2011). These methods would provide greater insight into the predator–prey relationships of sevengill sharks at Ōtākou and Te Whaka ā Te Wera.
Cloud cover was also identified as a significant predictor when assessing the likelihood of sighting a sevengill shark, with sharks more likely to be sighted with greater cloud cover. This conclusion is supported by the predatory behaviour of sevengill sharks observed elsewhere. For example, in Humboldt Bay, California sharks were observed foraging most actively during overcast days, or nocturnally (Ebert Citation1991). In Lüderitz Lagoon, Namibia sevengill sharks displayed very little response to extensive chumming from shore during daylight hours, contrasting with very active approaches at night (Ebert Citation1991). In addition, fine scale predator–prey studies conducted in southeast Tasmania suggest that sevengill sharks are nocturnal feeders, which may opportunistically prey on animals during daytime encounters (Barnett et al. Citation2010c).
The negative relationship between increasing sea state and the likelihood of sighting sevengill sharks may be interpreted in a number of ways. One explanation is that sharks did not alter their behaviour according to sea state, but our ability to detect the animals was affected. Incorporating quality underwater video equipment into future sampling methods would decrease this sampling bias (MacCauley et al. Citation2012). Alternatively, the increasingly poor environmental conditions associated with higher sea state may have affected the ability of sevengill sharks to detect the presence of food in disturbed water conditions. Behavioural research on great white sharks (Carcharodon carcharias) suggests that abiotic factors affecting chemoreception and vision will likely influence predator–prey dynamics (Hammerschlag et al. Citation2006). Therefore, if factors such as near-surface water flow and water clarity were influenced by an increased sea state, the ability of sevengill sharks to detect the chum and bait may have been compromised. To clarify the effects of sea state it is recommended that water clarity be included as a factor in future studies of distribution of sevengill sharks.
While we are confident that our methods provided a representative picture of relative sighting rates in different environmental conditions at the nearshore sites we sampled, there are a number of potential biases that should be acknowledged. As sampling was conducted from the research vessel at Te Whaka ā Te Wera and from the shore at Ōtākou some bias may have been introduced to the study, potentially contributing to some of the difference in encounter rates experienced at the two locations. This may be a form of gear bias (Robson & Regier Citation1964) whereby the fishing technique used affects the cohort of fish encountered (Kohler & Turner Citation2001; McCallister et al. Citation2013). Furthermore, our surveys were not intended to provide an estimate of the total number of sharks at sampling sites, simply to make first attempts to investigate seasonal variation and relative abundance. As sevengill sharks are typically associated with demersal feeding during daylight hours (Barnett et al. Citation2010c), luring the animals to the surface may not give an accurate estimate of the total number of sharks in the area. Passive sampling by chumming to attract sharks was chosen over fishing techniques, such as long-lining, to reduce the risk of injury to, or mortality of, the animals. Although underwater video was trialled to assess shark occurrence, water turbidity at Ōtākou meant this method was unsuccessful. In regions where water clarity is suitable, baited underwater video (e.g. Espinoza et al. Citation2014) could potentially be used to investigate relative abundance of sevengill sharks. Lastly, the limited spatial coverage of our study means our conclusions apply only to the specific locations we sampled. For example, all our study sites were nearshore in relatively shallow water and we may not have attracted sharks from nearby deeper habitat. Future studies should sample a wider range of locations in New Zealand to test the generality of our findings.
Acknowledgements
We are very grateful to all of the field assistants and skippers who worked on this project, in particular: S. King, E. Kearney, B. Twist, M. Desmond, S. O’Connell-Milne, C. Stewart, P. Russell, T. Stephens, K. Swann, T. Hill, P. Pidduck and S. Tranmer. We thank the Marine Science Department at the University of Otago for logistic support. We also thank Jonathan Banks and an anonymous reviewer for helpful comments on the manuscript.
Disclosure statement
No potential conflict of interest was reported by the authors.
References
- Abrantes KG, Barnett A. 2011. Intrapopulation variations in diet and habitat use in a marine apex predator, the broadnose sevengill shark Notorynchus cepedianus. Marine Ecology Progress Series. 442:133–148. doi: 10.3354/meps09395
- Barnett A, Abrantes KG, Stevens JD, Bruce BD, Semmens JM. 2010c. Fine-scale movements of the broadnose sevengill shark and its main prey the, gummy shark. PloS One. 5:1–10.
- Barnett A, Abrantes KG, Stevens JD, Semmens JM. 2011. Site fidelity and sex-specific migration in a mobile apex predator: implications for conservation and ecosystem dynamics. Animal Behaviour. 81:1039–1048. doi: 10.1016/j.anbehav.2011.02.011
- Barnett A, Abrantes KG, Stevens JD, Yick J, Frusher S, Semmens J. 2010b. Predator–prey relationships and foraging ecology of a marine apex predator with a wide temperate distribution. Marine Ecology Progress Series. 416:189–200. doi: 10.3354/meps08778
- Barnett A, Braccini M, Awruch CA, Ebert DA. 2012. An overview on the role of Hexanchiformes in marine ecosystems: Biology, ecology and conservation status of a primitive order of modern sharks. Journal of Fish Biology. 80:966–990. doi: 10.1111/j.1095-8649.2012.03242.x
- Barnett A, Redd KS, Frusher SD, Stevens JD, Semmens JM. 2010d. Non-lethal method to obtain stomach samples from a large marine predator and the use of DNA analysis to improve dietary information. Journal of Experimental Marine Biology and Ecology. 393:188–192. doi: 10.1016/j.jembe.2010.07.022
- Barnett A, Semmens JM. 2012. Sequential movement into coastal habitats and high spatial overlap of predator and prey suggest high predation pressure in protected areas. Oikos. 121:882–890. doi: 10.1111/j.1600-0706.2011.20000.x
- Barnett A, Stevens JD, Frusher SD, Semmens JM. 2010a. Seasonal occurrence and population structure of the broadnose sevengill shark Notorynchus cepedianus in coastal habitats of South-East Tasmania. Journal of Fish Biology. 77:1688–1701. doi: 10.1111/j.1095-8649.2010.02810.x
- Baum JK, Myers RA, Kehler DG, Worm B, Harley SJ, Doherty PA. 2003. Collapse and conservation of shark populations in the Northwest Atlantic. Science. 299:389–391. doi: 10.1126/science.1079777
- Bonfil R. 1997. Status of shark resources in the Southern Gulf of Mexico and Caribbean: implications for management. Fisheries Research. 29:101–117. doi: 10.1016/S0165-7836(96)00536-X
- Boyd RO. 2008. Fisheries resources in Otago Harbour and on the adjacent coast. https://www.nextgenerationportotago.nz/assets/Uploads/PDF-Uploads/Preliminary-Reports-Documents/7-Fisheries-Preliminary-Boyd-2008.pdf.
- Bozdogan H. 1987. Model selection and Akaike’s Information Criterion AIC: the general theory and its analytical extensions. Psychometrika. 52:345–370. doi: 10.1007/BF02294361
- Bruce BD, Stevens JD, Malcolm H. 2006. Movements and swimming behaviour of white sharks (Carcharodon carcharias) in Australian waters. Marine Biology. 150:161–172. doi: 10.1007/s00227-006-0325-1
- Burnham K, Anderson D. 2002. Model selection and multimodel inference: a practical information-theoretic approach, 2nd ed. New York: Springer-Verlag.
- Cox G, Francis M. 1997. Sharks and rays of New Zealand. Christchurch: Canterbury University Press.
- Crespi-Abril AC, García NA, Crespo EA, Coscarella MA. 2003. Consumption of marine mammals by broadnose sevengill shark Notorynchus cepedianus in the Northern and Central Patagonian Shelf. Latin American Journal of Aquatic Mammals. 2:101–107. doi: 10.5597/lajam00038
- Dambach J, Rödder D. 2011. Applications and future challenges in marine species distribution modeling. Aquatic Conservation: Marine and Freshwater Ecosystems. 21:92–100. doi: 10.1002/aqc.1160
- Department of Conservation New Zealand. 2012. Stewart Island/Rakiura conservation management strategy and Rakiura National Park Management Plan 2011–2021. https://www.doc.govt.nz/about-us/our-policies-and-plans/statutory-plans/statutory-plan-publications/conservation-management-strategies/stewart-island-rakiura/.
- Dill LM, Heithaus MR, Walters CJ. 2003. Behaviorally mediated indirect interactions in marine communities and their conservation implications. Ecology. 84:1151–1157. doi: 10.1890/0012-9658(2003)084[1151:BMIIIM]2.0.CO;2
- Drymon JM, Ajemian MJ, Powers SP. 2014. Distribution and dynamic habitat use of young bull sharks Carcharhinus leucas in a highly stratified Northern Gulf of Mexico estuary. PLoS ONE. 9:e97124. http://doiorg/101371/journalpone0097124. doi: 10.1371/journal.pone.0097124
- Ebert DA. 1989. Life history of the sevengill shark, Notorynchus cepedianus (Peron 1807), in two Northern California Bays. California Fish and Game. 75:102–112.
- Ebert DA. 1991. Observations on the predatory behaviour of the sevengill shark Notorynchus cepedianus. South African Journal of Marine Science. 11:455–465. doi: 10.2989/025776191784287637
- Ebert DA. 2002. Ontogenetic changes in the diet of the sevengill shark Notorynchus cepedianus. Marine and Freshwater Research. 53:517–523. doi: 10.1071/MF01143
- Elith J, Leathwick JR. 2009. Species distribution models: ecological explanation and prediction across space and time. Annual Review of Ecology Evolution and Systematics. 40:677–697. doi: 10.1146/annurev.ecolsys.110308.120159
- Ferretti F, Worm B, Britten GL, Heithaus MR, Lotze HK. 2010. Patterns and ecosystem consequences of shark declines in the ocean. Ecology Letters. 13:1055–1071.
- Espinoza M, Cappo M, Heupel MR, Tobin AJ, Simpfendorfer CA. 2014. Quantifying shark distribution patterns and species-habitat associations: implications of Marine Park zoning. PLoS One. 9:1–17. doi: 10.1371/journal.pone.0106885
- Gey S, Nedelec E. 2005. Model selection for CART regression trees. IEEE Transactions on Information Theory. 51:658–670. doi: 10.1109/TIT.2004.840903
- Gill P, Morrice M, Page B, Pirzl R, Levings A, Coyne M. 2011. Blue whale habitat selection and within-season distribution in a regional upwelling system off southern Australia. Marine Ecology Progress Series. 421:243–263. doi: 10.3354/meps08914
- Guisan A, Edwards TC, Hastie T. 2002. Generalized linear and generalized additive models in studies of species distributions: setting the scene. Ecological Modelling. 157:89–100. doi: 10.1016/S0304-3800(02)00204-1
- Hacohen-Domené A, Martínez-Rincón RO, Galván-Magaña F, Cárdenas-Palomo N, de la Parra-Venegas R, Galván-Pastoriza B, Dove ADM. 2015. Habitat suitability and environmental factors affecting whale shark Rhincodon typus aggregations in the Mexican Caribbean. Environmental Biology of Fishes. 98:1953–1964. doi: 10.1007/s10641-015-0413-5
- Hammerschlag N, Martin RA, Fallows C. 2006. Effects of environmental conditions on predator–prey interactions between white sharks (Carcharodon carcharias) and Cape fur seals (Arctocephalus pusillus pusillus) at Seal Island South Africa. Environmental Biology of Fishes. 76:341–350. doi: 10.1007/s10641-006-9038-z
- Heithaus MR. 2001. The biology of tiger sharks, Galeocerdo cuvier, in Shark Bay, Western Australia: sex ratio, size distribution, diet, and seasonal changes in catch rates. Environmental Biology of Fishes. 61:25–36. doi: 10.1023/A:1011021210685
- Hight BV, Lowe CG. 2007. Elevated body temperatures of adult female leopard sharks, Triakis semifasciata, while aggregating in shallow nearshore embayments: evidence for behavioral thermoregulation? Journal of Experimental Marine Biology and Ecology. 352:114–128. doi: 10.1016/j.jembe.2007.07.021
- James GD, Unwin MJ, Carbines G. 2004. Stewart Island Marine recreational fishing survey 2002/2003. Final research report for ministry of fisheries project REC2001/04. http://fs.fish.govt.nz/Doc/22575/REC2001-04%20Survey%20Marine%20recreational%20fishing%20Stewart%20Island%20Final.pdf.ashx.
- James M, Boyd R, Probert K. 2010. Information on key species of interest to Ngai Tahu—supplementary paper for next generation project. https://www.orc.govt.nz/media/3257/26-key-species-ngai-tahu-james_boyd_probert-2010-va275964.pdf.
- Knip D, Heupel M, Simpfendorfer C. 2010. Sharks in nearshore environments: models, importance, and consequences. Marine Ecology Progress Series. 402:1–11. doi: 10.3354/meps08498
- Kohler N, Turner P. 2001. Shark tagging: a review of conventional methods and studies. Environmental Biology of Fishes. 60:191–223. doi: 10.1023/A:1007679303082
- Kuhn CE, Crocker DE, Tremblay Y, Costa DP. 2009. Time to eat: measurements of feeding behaviour in a large marine predator, the northern elephant seal Mirounga angustirostris. Journal of Animal Ecology. 78:513–523. doi: 10.1111/j.1365-2656.2008.01509.x
- Larson S, Farrer D, Lowry D, Ebert DA. 2015. Preliminary observations of population genetics and relatedness of the broadnose sevengill shark, Notorynchus cepedianus, in two Northeast Pacific estuaries. Plos One. 10:e0129278. http://doiorg/101371/journalpone0129278. doi: 10.1371/journal.pone.0129278
- Lucifora L, Menni R, Escalante A. 2005. Reproduction, abundance and feeding habits of the broadnose sevengill shark Notorynchus cepedianus in north Patagonia Argentina. Marine Ecology Progress Series. 289:237–244. doi: 10.3354/meps289237
- MacCauley DJ, McLean KA, Bauer J, Young HS, Micheli F. 2012. Evaluating the performance of methods for estimating the abundance of rapidly declining coastal shark populations. Ecological Applications. 22:385–392. doi: 10.1890/11-1059.1
- McCallister M, Ford R, Gelsleichter J. 2013. Abundance and distribution of sharks in Northeast Florida waters and identification of potential nursery habitat. Marine and Coastal Fisheries. 5:200–210. doi: 10.1080/19425120.2013.786002
- McCullagh P, Nelder JA. 1983. Generalized linear models. London: Chapman and Hall.
- Myers RA, Baum JK, Shepherd TD, Powers SP, Peterson CH. 2007. Cascading effects of the loss of apex predatory sharks from a coastal ocean. Science. 315:1846–1850. doi: 10.1126/science.1138657
- Pankhurst NW, Conroy AM. 1987. Seasonal changes in reproductive condition and plasma levels of sex steroids in the blue cod, Parapercis colias (Bloch and Schneider) (Mugiloididae). Fish Physiology and Biochemistry. 4:15–26. doi: 10.1007/BF02073862
- Robbins RL. 2007. Environmental variables affecting the sexual segregation of great white sharks Carcharodon carcharias at the Neptune Islands South Australia. Journal of Fish Biology. 70:1350–1364. doi: 10.1111/j.1095-8649.2007.01414.x
- Robertson MP, Peter CI, Villet MH, Ripley BS. 2003. Comparing models for predicting species’ potential distributions: a case study using correlative and mechanistic predictive modelling techniques. Ecological Modelling. 164:153–116. doi: 10.1016/S0304-3800(03)00028-0
- Robson DS, Regier HA. 1964. Sample size in Petersen mark-recapture experiments. Transactions of the American Fisheries Society. 93:215–226. doi: 10.1577/1548-8659(1964)93[215:SSIPME]2.0.CO;2
- Roper DS, Jillett JB. 1981. Seasonal occurrence and distribution of flatfish (Pisces: Pleuronectiformes) in inlets and shallow water along the Otago coast. New Zeal Journal of Marine and Freshwater Research. 15:1–13. doi: 10.1080/00288330.1981.9515891
- Smith AM, Wood ACL, Liddy MFA, Shears AE, Fraser CI. 2010. Human impacts in an urban port: the carbonate budget, Otago Harbour, New Zealand. Estuarine, Coastal and Shelf Science. 90:73–79. doi: 10.1016/j.ecss.2010.07.004
- Soldo A, Peirce R. 2005. Shark chumming in the Eastern Adriatic. Annales Series Historia Naturalis. 15:203–208.
- Speed CW, Field IC, Meekan MG, Bradshaw CJA. 2010. Complexities of coastal shark movements and their implications for management. Marine Ecology Progress Series. 408:275–293. doi: 10.3354/meps08581
- Stehfest KM, Patterson TA, Barnett A, Semmens JM. 2014. Intraspecific differences in movement, dive behavior and vertical habitat preferences of a key marine apex predator. Marine Ecology Progress Series. 495:249–262. doi: 10.3354/meps10563
- Van Dykhuizen G, Mollet HF. 1992. Growth, age estimation and feeding of captive sevengill sharks, Notorynchus cepedianus, at the Monterey Bay aquarium. Australian Journal of Marine and Freshwater Research. 43:297–318. doi: 10.1071/MF9920297
- Wagenmakers EJ, Farrell S. 2004. AIC model selection using Akaike weights. Psychonomic Bulletin & Review. 11:192–196. doi: 10.3758/BF03206482
- Williams GD, Andrews KS, Katz SL, Moser ML, Tolimieri N, Farrer DA, Levin PS. 2012. Scale and pattern of broadnose sevengill shark Notorynchus cepedianus movement in estuarine embayments. Journal of Fish Biology. 80:1380–1400. doi: 10.1111/j.1095-8649.2011.03179.x