Abstract
A shoreline and archaeological excavations at Cook's Cove, eastern North Island, New Zealand were examined for stratigraphy and plant microfossils and results compared with previous interpretations of this site. Buried soils, distal tephras and pollen revealed evidence of pre- and post-settlement forest disturbance. Microfossil starch and calcium oxalate crystals of introduced sweet potato (Ipomoea batatas) and taro (Colocasia esculenta) were identified throughout the deposits, indicating intensive local cultivation and processing of these crops. Results are consistent with revised New Zealand geochronological models and, bearing in mind loss of evidence by erosion, differ in several respects to those of previous studies, as follows. The sea-rafted Taupo Pumice (AD 200) and Loisel's Pumice (from multiple off-shore sources) were not as widespread. Although microscopic charcoal was present to the full depth of all profiles, we did not observe macroscopic pieces below the Loisel's Pumice. The only material we found that appeared to equate to the deposit previously identified tentatively as air-fall Kaharoa Tephra (AD 1300) appeared not to be a tephra. Finally, we found little evidence for marine inundation of the site.
Introduction
Recent studies of plant microfossils from the East Coast of the North Island, New Zealand have yielded evidence of landscape disturbance and Maori horticulture. The first of these studies examined sediment profiles from Anaura Bay and compared results with descriptions of that site made in 1769 by Captain Cook's Endeavour expedition, while a subsequent study examined profiles in the Tolaga Bay catchment (Horrocks et al. Citation2008a, Citationb). Both studies showed evidence of large-scale deforestation and Polynesian-introduced crops.
An opportunity to expand on these East Coast studies arose with a recent archaeological excavation at Cook's Cove (Walter et al. Citation2011) (). The site (Z17/311 in the NZ Archaeological Association Site Recording Scheme) had previously been described in a geological context by Wellman (Citation1962:45–48) as part of his coastal reconnaissance of Holocene deposits of the North Island. Based on continuous exposures on the eroding shoreline, he rated the Cook's Cove section as one of the best in the North Island, describing it as the ‘type locality for the Holocene divisions’. He identified two distinct Maori occupation layers with abundant macroscopic artefacts and also identified several Late Holocene tephra layers. The latter, which he used as time markers, have wide distribution in the North Island and are important archaeologically because they constrain the timing of first settlement of New Zealand. Wellman (Citation1962) also identified possible natural catastrophic events such as a tsunami in the Cook's Cove deposits. Subsequent New Zealand coastal stratigraphic and tephra studies that included Cook's Cove provided radiocarbon data and discussed timing of regional settlement (Pullar et al. Citation1977; McFadgen Citation1982, Citation1985, Citation1994, Citation2007).
We present here results of plant microfossil analysis of archaeologically excavated profiles and eroded shoreline sections at Cook's Cove. Deposits associated with intensive human activity are examined and discussed in geomorphological and archaeological contexts.
The study area
Cook's Cove is a small sheltered inlet on the southern headland of Tolaga Bay (). It is separated from the Bay by a narrow ridge cut through by a small stream which now discharges into Tolaga Bay through a natural tunnel, having abandoned its former outlet at Cook's Cove (Wellman Citation1962). However, there is another small stream that flows into the cove. The hills surrounding Cook's Cove rise to c. 100 m above sea level. Mudstones and siltstones of upper Tertiary age, often loose-jointed and therefore easily eroded, form the soils of the region (New Zealand Soil Bureau Citation1973).
Meteorological records at Gisborne, 40 km SW of Cook's Cove, show that mean annual rainfall is 1050 mm, with a winter maximum (NIWA Citation2007). Mean daily air temperature is 14.3 °C, and the average daily maximum and minimum temperatures are 19.5 °C and 9.1 °C, respectively. The average number of days of ground frost is 33.
A fossil pollen record from Gisborne shows that the region was covered in matai (Prumnopitys taxifolia) and rimu (Dacrydium cupressinum)-dominated podocarp-hardwood forest. There is evidence of widespread burning of this by the first people c. 650 yr BP (Wilmshurst et al. Citation1999).
There have been very few subsurface archaeological investigations in the East Coast region. However, field surveys have disclosed high densities of archaeological sites, suggesting that the region sustained substantial populations, and it has been inferred from the distribution of food storage pits that pre-European gardening was concentrated along river valleys and on the coastal flats and hills (Jones Citation1983a, Citation2007). The Tolaga Bay catchment, directly adjacent to Cook's Cove, was a particularly important focus of settlement based around gardening, beginning perhaps as early as the thirteenth century AD (Jones & Law Citation1987; Jones Citation1990). The recent archaeological excavation at Cook's Cove (Walter et al. 2011) was the first major excavation of prehistoric deposits thus far carried out on the East Coast, and is discussed further below.
Cook's Cove was visited by the Endeavour, Captain James Cook, for six days in October 1769. Both Cook and his botanist, Joseph Banks, noted that the hills around the cove were wooded, but there were also hillslope gardens in which sweet potato and yams were growing (Beaglehole Citation1962:419, 1968:186–187; Jones Citation1986). It is not clear whether there were also gardens on the flats here, but Banks observed that houses in the valley inland of the cove appeared to be deserted in preference for those on higher ground, although Te Kararoa pa (Z17/310) on the ridge top immediately to the north of the cove was derelict (Beaglehole Citation1962:419–420; Jones Citation1983b). Some of Dumont D'Urville's crew from L'Astrolabe visited Cook's Cove in 1827, but left no specific record of it. When JS Polack visited in 1835 it was ‘a neglected valley’, largely overgrown, and visited occasionally only for fishing (Polack Citation1838:134–136). However, an 1869 drawing (Walter et al. 2011: fig. 3) shows Maori activity there and hills largely clear of woody vegetation, while a photograph c. 1900 (Walter et al. 2011: fig. 4) shows widespread return of scrub. Today, the Cook's Cove flats are grazed and the surrounding hills are covered in a mixture of pasture, scrub and regenerating forest.
The section described by Wellman (Citation1962: fig. 7) is on the south side of Cook's Cove (, ). His illustration is a summary profile, compressing c. 230 m of exposed shoreline into a short diagram. This shows two occupation layers separated by shelly sand with a discontinuous light-brown tuffaceaous layer in the centre, tentatively identified as Kaharoa Tephra, a distal tephra erupted c. AD 1300 (Lowe et al. Citation1998; Buck et al. Citation2003). As the shelly sand appeared to be wave-deposited and well above the 1962 storm waves, he suggested a tsunami or period of severe storms. Loisels Pumice, sea-rafted from the Healy caldera and possibly other sources in the southwest Pacific (Shane et al. Citation1998; Wright et al. Citation2003), was at the base of the lower occupation layer and a few inches above the lowest visible charcoal fragments. The distal Taupo eruption of c. AD 200 (Lowe et al. Citation2000) is represented in the section by soily tuff with sea-rafted pumice lumps. Estuarine sand with totara (Podocarpus totara) logs (none in growth position) underlies the Taupo deposits. Three samples collected by Wellman were subsequently dated (Pullar et al. Citation1977; McFadgen Citation1982, Citation1994), indicating initial human settlement of this locality some time between the mid thirteenth and mid fifteenth centuries AD. McFadgen (Citation1985) correlated the two Cook's Cove occupation layers with two buried soils he identified at coastal sites throughout New Zealand, formed during stable environmental phases towards the end of both the ‘Tamatean’ and overlying ‘Ohuan’ chronozones. These results are discussed further below in relation to more recently acquired evidence.
Figure 2 Cook's Cove 2005 viewed from the west showing sampling site on eroding south bank (arrow). Wooded area level with the horizon is Pourewa Island. Ridge, left, separates the cove from Tolaga Bay. The stream that cut the valley previously flowed into the cove and now discharges into Tolaga Bay through a natural tunnel in the ridge (out of view). Another stream, originating from a valley on the right, is visible flowing into the cove. Water body, centre, is a stock pond on higher ground.
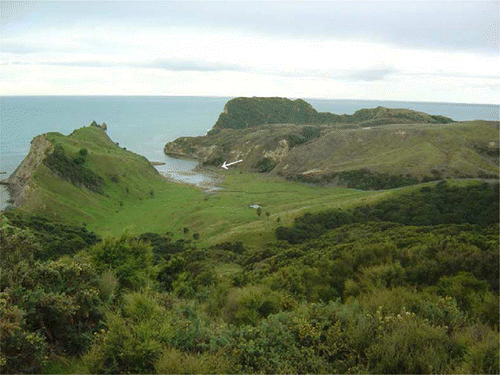
The south side of the cove is actively eroding and several metres have been lost since Wellman's (1962) study. It was this that prompted the 2007 excavations, which opened 32.5 m2 in four discrete areas (Units 1– 4) on flat ground immediately behind the eroding section on the south shore (). Each of these extended from the surface into natural deposits below the lowest occupation layer, providing a more detailed picture of both natural and cultural stratigraphy than previously available. They also provided more reliable estimates of the age of the lowest occupation, with radiocarbon dates on marine shell and moa bone indicating initial settlement by about the middle of the fourteenth century by people whose subsistence pursuits included fishing, shellfish gathering and hunting of seals, moa and a wide range of marine and forest birds (Walter et al. 2011). The early occupation layer represents about a century of repeated or continuous use of the site before it was buried by a deep colluvial deposit. Inclusions of crushed shell in the soil overlying this layer are interpreted as evidence of gardening during a second period of occupation of the site. Although not precisely dated, reoccupation began in late prehistory and extended into the early historic period.
Methods
Three exposed, eroded sections (‘seaward’, ‘central’ and ‘landward’) and two profiles from baulks within excavation units (O11, P11) at Cook's Cove were examined for stratigraphy and evidence of gardening or plant food preparation (Fig. 1). The central section was selected as the part of the eroding south side of the cove that appeared to correlate most closely with Wellman's (1962) summary profile. The seaward and landward sections were located so as to sample points near the NE and SW extents of cultural stratigraphy visible in the exposed section. The O11 profile was from the southeast baulk of square O11 in excavation Unit 1, and the P11 from the northeast baulk of square P11 in excavation Unit 4 (Walter et al. 2011).
Sampling for plant microfossils (pollen and starch) was carried out in a vertical sequence for each profile to give progressive histories. Four oven features (F) in excavation Unit 1 were also sampled, F1 from the upper occupation (Layer 2) and F55, F64 and F84 from the lower occupation (Layer 5).
Samples (3–5 cm3) were prepared for pollen (and spore) analysis by the standard acetylation method (Moore et al. Citation1991). Of the samples that had sufficient pollen for counting, at least 150–200 pollen grains and spores were counted for each sample. All pollen and spore types were included in the pollen sum. Microscopic fragments of charcoal are extracted along with pollen during preparation, providing evidence of fire. Slides of samples with insufficient pollen for counting were also scanned and charcoal and occasional pollen types noted.
As well as starch grains, starch analysis includes other plant material such as calcium oxalate crystals (Torrence & Barton Citation2006). Samples (2.5–6.5 cm3) were prepared for analysis of starch and other residues by density separation (Horrocks Citation2005). For the three exposed sections, presence/absence of these was noted. For the pit and trench, tablets containing a known quantity of exotic Lycopodium spores were added to the samples to allow volumetric counts of starch and associated remains.
Results
Stratigraphy
The stratigraphy of each section is summarized in , which also shows correlations between them by placement of each stratum in one of five phases. These phases represent our interpretation of the sequence of natural processes and cultural events at the site. Layers have been assigned to phases on the basis of observed correlations, in the case of exposed sections, depth and composition. In the seaward section (a), Phase I is represented by a light grey shelly sand interspersed with gravel and large siltstone cobbles at the base of the section. This is overlain by a dark, charcoal-rich silty soil containing midden and oven debris forming the lowest human occupation deposit in the sequence (Phase II). A thick deposit of sandy clay interspersed with fine shell fragments (Phase III) separates the lower occupation layer from a second, dark charcoal-rich layer with whole shells that forms the upper occupation deposit (Phase IV). This grades into a shallow topsoil with no apparent occupational evidence, representing recent post-occupation soil development (Phase V).
Figure 3 Sampled profiles, Cook's Cove (sample locations visible in (a), (b), (c); high tides reach base of same; stratigraphic numbers 1–7 in (d), (e) = excavation layer designations). A, Seaward, 2007 (sampled depth, from near surface to basal sand with pebbles, is 150 cm). B, Central, 2005 (sampled depth 100 cm). Clasts of light-coloured, sea-rafted Taupo Pumice are visible, lower arrows. Position of layer of sea-rafted Loisels Pumice clasts, camouflaged by their grey colour, shown by upper arrows. C, Landward, 2007 (sampled depth 100 cm). D, Excavation O11, east baulk, 2007. E, Excavtion P11, north baulk, 2007.
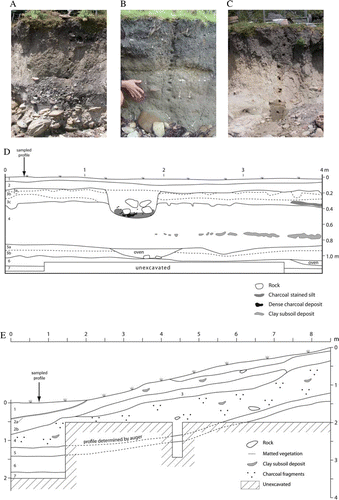
Table 1 Stratigraphy of Cook's Cove profiles and inferred phase correlations (depth measured from ground surface).
All five phases are represented in the central section (b). Here Phase I includes a silty soil above the shelly sand and cobbles. Above this, the lower occupation layer (Phase II) is charcoal-rich but contains only sparse midden remains. The intervening shelly sand, shown mid-section and indented in b, is a much shallower deposit than the equivalent Phase III stratum in the seaward section. The upper occupation layer is similar in form and depth to the seaward Phase IV deposit, and grades into the recent topsoil. The central section also shows two zones of sparse, dispersed, sea-rafted clasts of pumice. The lowest of these is immediately above the basal siltstone cobbles and the second immediately below the lower occupation layer, placing both within Phase I. These are the c. AD 200 Taupo Pumice (Lowe et al. Citation2000) and subsequent Loisels Pumice, respectively. The two are readily differentiated by eye; Taupo Pumice is light yellowish-brown and has coarse irregular gas cavities while Loisels is grey with black bands and has fine cavities (McFadgen Citation1994). The lower occupation layer in this section (Phase II) also contains fine, brown tephra-like lenses that we equate with the ‘discontinuous light brown tuffaceous layer, probably subaerial ash’ that Wellman (Citation1962:46) tentatively identified as c. AD 1300 Kaharoa Tephra (Lowe et al. Citation1998; Buck et al. Citation2003). However, this showed no recognizable glass shards when examined at low-powered magnification. Assuming that we sampled the same deposit that Wellman observed, this demonstrates that it was almost certainly not a tephra.
The landward section (c) provides a simpler profile. This comprises a silty sand with siltstone cobbles beneath a thick layer of silty clay, overlain by a thick, charcoal-rich surficial layer with very occasional shell inclusion. The latter is almost certainly a continuation of the upper occupation layer (Phase IV) and recent topsoil (Phase V) identified in the previous two sections, as detailed examination of the intervening portion of the eroding shoreline shows that the lower occupation peters out c. 10 m south of the central section (Walter et al. 2011: Appendix 1). There can be little doubt that the lowermost component of this section correlates with the basal layer in the seaward and central sections, and this may also be the case for the lower part of the middle component. However, the upper part of this is likely to correlate with Phase III deposits elsewhere, but in the absence of the lower occupation it is difficult to determine where the subdivision between phases I and III would lie.
The O11 profile (d) shows a basal shelly sand beneath a brown silty soil that closely matches the Phase I components in the central section. The lower occupation layer (Phase II) is a well-developed soil into which several ovens and fire scoops had been dug. The overlying silty clay (Phase III) contained sparse inclusions of both culturally derived material and clay nodules, suggesting that it was formed, at least in part, by erosion of adjacent hill slopes on which human occupation had already occurred. The upper occupation (Phase IV) comprises two components; a soil mixed with crushed shell that is interpreted as a garden soil, and a charcoal-darkened soil from which a deep oven had been dug. While the presence of this feature and its artefactual contents demonstrates that the layer 2 soil began to develop during the upper occupation phase, the otherwise sterile nature of the soil suggests that most of its development should be assigned to Phase V, along with the shallow yellow clay at the surface derived from recent slope wash.
The P11 profile (e) shows the basal shelly sand and overlying silty soil (Phase I) and the lower occupation layer (Phase II) wherever the excavations in this unit were deep enough to encounter them. It also shows the layer burying the lower occupation (Phase III) extending up the hill slope and with more clay content than on the flat at O11, consistent with our interpretation of it as colluvial. The upper occupation (Phase IV) is represented by scattered cultural material in a reddish brown, silty clay soil, which also contains isolated patches of probable gardening disturbance (not sampled in our pollen/starch profile). This soil clearly continued development into the recent era (Phase V), as part of the profile shows a lens of vegetation that must have been growing on the surface of this soil before it was buried by recent slope wash (perhaps during cyclone Bola in 1988) which accumulated deeply here, just upslope of a fence line.
Pollen and spores
All of the Cook's Cove samples had microscopic fragments of charcoal, to the full depth of the profiles. Although charcoal was not quantified, the oven feature samples had noticeably higher concentrations. Pollen and spore preservation ranged from good to mostly poor, with many samples having insufficient pollen for counting. Of the exposed sections, the central section provides the most detail, with manuka/kanuka (Leptospermum/Kunzea) pollen showing an unusually high value (100%) in the lowermost sample, from the Phase I horizon (b). A single hornwort (Anthocerotales) spore was also found in this sample and more were found from after 60 cm depth (top of Phase II) to the surface. Spores of bracken (Pteridium) and pollen of puha/dandelion (Sonchus/Taraxacum) first appear at c. 70 cm depth, in Phase II, the former having high values in the up-section samples where counts were conducted, at 47 cm depth (Phase III) and in the uppermost two samples (Phase V). Brassospora pollen is recorded mid-section, from 72 to 30 cm depth, across deposits encompassing both occupation layers (Phases II, III, IV). This is from a group of southern broad-leaf beeches that became extinct in New Zealand c. 1 million years ago (Mildenhall & Byrami Citation2004). European-introduced pine (Pinus) pollen first appears at 25 cm depth, in the upper occupation layer (Phase IV).
Figure 4 Pollen and starch diagrams, Cook's Cove. (Pollen: bars =%,+= found after count; Starch:+= present). a, Seaward. b, Central. c, Landward. d, O11. e, P11. f, Oven features.
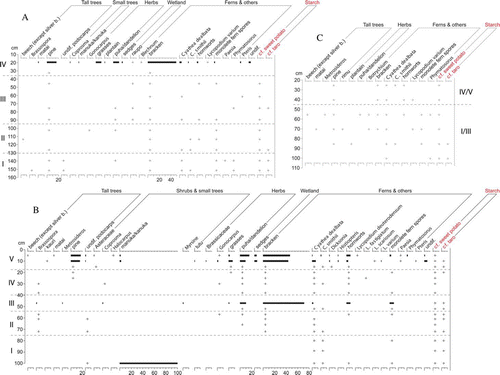
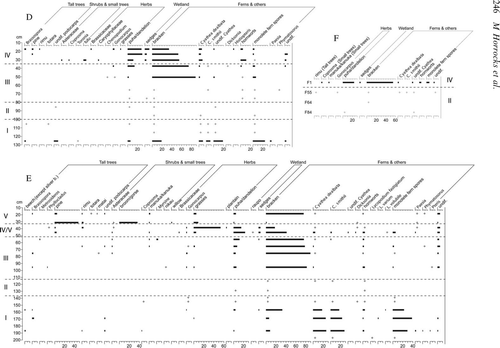
Of the remaining two exposed sections, only the uppermost sample of the seaward section (from Phase IV) had sufficient pollen for counting, showing abundant bracken spores. However, these and pine pollen were found to the full depth (c. 150 cm) of this section and pine pollen was also found to near the base of the landward section (c. 85 cm depth) (a, c).
Spores of Cyathea and other ferns are abundant in the lowermost sample from excavation O11 (d), from the upper part of the basal shelly sand (Phase I). Bracken spores and puha/dandelion pollen are also present here, along with a small amount of podocarp pollen. Pollen is not sufficiently concentrated in subsequent O11 samples for counting until midway through the colluvial fill layer (Phase III), at c. 50 cm depth. Puha/dandelion and especially bracken have high values in these upper samples. Pine pollen was found to c. 25 cm depth in the upper occupation layer (Phase IV).
Excavation P11 also had abundant spores of Cyathea and other ferns, bracken and hornworts, and pollen of puha/dandelion in Phase I samples from 155 cm and below, in the basal shelly sand and the subsoil above this (e). Pollen is not sufficiently concentrated in subsequent samples for counting until above the lower occupation layer and into the overlying clay at c. 90 cm depth (Phase III). Bracken spores have high values at the start of this upper sequence, then decline as pollen of puha/dandelion and grasses (Poaceae) increases, the latter with a very high value at c. 40 cm depth (Phase IV/V). Immediately above this in the buried matted layer of vegetation, pine and broom/gorse (Ulex/Cytisus) pollen show similarly high values. Pine pollen was found to a little below 45 cm depth in this profile (Phase IV/V).
Of the oven features in excavation O11, only F1 (Phase IV) had sufficient pollen for counting, again with puha/dandelion pollen present and abundant bracken spores (f). Pine pollen was not found in any of the oven features.
Starch and other remains
Two types of starch residues were identified in the Cook's Cove samples. The following rationale for identification of the starch types to species is from previous ancient starch studies (Horrrocks et al. 2008a,b; Horrocks & Rechtman 2009). The first type of starch comprises medium-sized grains, ovate to sub-triangular, often bell-shaped, with a vacuole (often fissured) at the central hilum, and one domed surface and several flattened pressure facets, consistent with root of sweet potato (Ipomoea batatas) (a–c, , a–c, a–c), found as individual grains. A few reasonably well-preserved examples of these (i.e. colourless with Maltese cross) were found as individual grains. However, the vast majority were degraded, showing discolouration (yellow/amber), expansion and distortion of the grain and vacuole, loss of the Maltese cross, pitting, cracking, fragmentation and disintegration. These have been identified at archaeological sites elsewhere (Horrocks & Barber Citation2005; Horrocks et al. Citation2007a; Horrocks & Wozniak Citation2008; Horrocks & Rechtman Citation2009).
Figure 6 Microfossils of Polynesian-introduced cultigens from Cook's Cove (mounted in glycerol jelly; transmitted or cross-polarized light (CPL), the latter with black background; 400× or 600×; scale bars: 20 µm). A–C, Faceted starch grains with central vacuoles (cf. starch grains of Ipomoea batatas root, a–7c). Well-preserved grains shown in (a), with lower left grain also shown in CPL with central Maltese cross. Degraded, discoloured grains shown in (b,c), with vacuoles in (b) greatly expanded. D, High concentration of tiny starch grains within cell membrane (cf. amyloplast of Colocasia esculenta corm, d). E, Raphide (cf. ‘long-thin’ raphides of C. esculenta, e). F, Raphide (cf. ‘short-thick’ raphide of C. esculenta, f). G,H, Druse, also shown in CPL (cf. druse of C. esculenta, g, h).
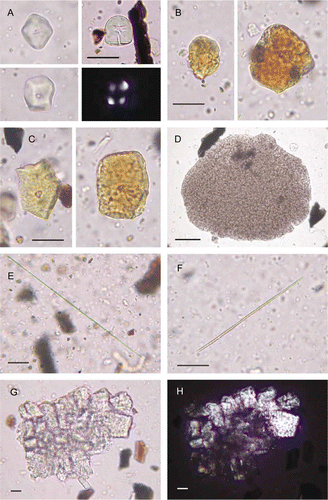
Figure 7 Microparts of cultigens from modern reference samples (mounted in glycerol jelly; transmitted or cross-polarized light (CPL), the latter with black background; 400× or 600×; scale bars: 20 µm). A–C, Starch grains of Ipomoea batatas root. Grains are spherical to sub-spherical, often bell-shaped, up to c. 25 µm diam., with a vacuole at the central hilum, and nearly all have one domed surface and up to six flattened pressure facets. Vacuole clearly visible in lower left grain (b). Top left grain (a) has six facets. Grains in (a) viewed in CPL with central Maltese cross. D, Amyloplast of Colocasia esculenta corm, with high concentration of tiny grains. Abundant individual grains are scattered outside. Amyloplasts are ovate, up to c. 185 µm diam., with thin walls, <0.5 µm thick. Grains are mostly <4 µm in diameter, spherical and often angular. E, ‘Long-thin’ raphides (and scattered starch grains) of C. esculenta. These are up to c. 125 µm long and <0.25 µm diam. F, ‘Short-thick’ raphides (and scattered starch grains) of C. esculenta. These are up to c. 90 µm long and 1.0–2.5 µm diam. G,H, Druse of C. esculenta, also shown in CPL.
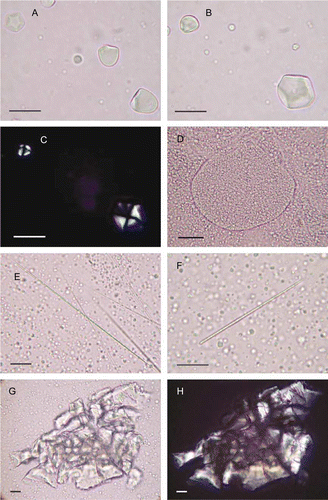
The second type of starch (and associated material) identified comprises tiny, spherical angular particles consistent with starch grains of the corm of taro (Colocasia esculenta). These were found densely packed in masses or amyloplasts (hundreds to thousands of grains) (d). Also found were calcium oxalate crystals (raphides and druses) consistent with taro (e–h). These are mostly raphides (needle-like), occasionally found in partial bundles but mostly as individuals or fragments. Although calcium oxalate crystals occur in many plant taxa, the ‘long-thin’ raphide type we identified reduces the possibilities and these, the ‘short-thick’ raphide type and the druses we found are especially abundant in corms and shoots of taro (Figs. 7e–7h) (Sunell & Healey Citation1979; Loy et al. Citation1992). We conclude that the raphides are probably from this taxon. Druses were identified only in the seaward section. In this section several largely intact druses were found; unlike raphides, when druses are fragmented they are difficult to distinguish from other mineral particles on slide preparations.
Sweet potato and taro remains were identified in all three of the exposed sections, with the former taxon recorded in more samples (a–c). In the remaining profiles (O11, P11 and oven features), where concentrations were quantified, sweet potato starch grains and taro raphides were most highly concentrated in P11, notably at 115 cm and 130 cm depths (>10,000 and 1500 per cm3, respectively), in the lower occupation layer (Phase II) (). The highest concentrations in O11 were at 95 and 115 cm depths, in the lower occupation layer (Phase II) and underlying subsoil (Phase I) respectively, with raphides recorded in more samples than in the P11 (<1000 per cm3). Concentrations were lowest in the oven features, with cf. sweet potato starch grains and cf. taro raphides <2500 per cm3 and <400 per cm3, respectively. Taro amyloplasts were identified in low concentrations in all three excavation contexts (<300 per cm3). Remains of cf. sweet potato were thus more abundant than cf. taro at Cook's Cove, being found more highly concentrated and in more samples.
Discussion and conclusions
Taphonomy and preservation of plant microfossils
The Cook's Cove sections we examined revealed a series of buried soils and other deposits. The behaviour of plant microfossils in New Zealand soils has been discussed elsewhere (Horrocks et al. Citation2008b), building on theoretical processes of pollen incorporation into soils and other well-drained substrates modelled by Dimbleby (Citation1985). Pollen is deposited on the ground surface and carried downwards through the soil by percolating groundwater. The percolating profile mainly conforms to the chronological order of pollen deposition at the surface with older pollen found increasingly towards the bottom of the profile. The rate that these processes operate at depends on such factors as soil type and drainage, rainfall and vegetation. This effect has been disrupted by three additional processes at Cook's Cove: mixing by mechanical disturbance by people, burial, and exposure of profiles by erosion. The first of these is likely to be significant in the upper part of at least some of the profiles where Maori gardening is in evidence, while the digging of ovens and fire scoops during the early occupation phase may have impacted on the lower parts of the profiles. The deep colluvial deposit burying the lower occupation appears to have provided at least a partial barrier to percolation and downward movement of microfossils, perhaps contributing to the sharp declines in bracken spores within or below this level in the O11 and P11 profiles (d, e). The third of these processes is probably responsible for the much deeper penetration of European-introduced pine pollen in two of the exposed sections (seaward, to 150 cm; landward, to 85 cm) than in the excavated profiles (O11, to 25 cm; P11, to 45 cm).
Considerable variation in amounts of plant microfossils (percentages of pollen and concentrations of starch remains) is shown in some of the Cook's Cove sections (, ), indicating heterogeneity of sections allowing interpretation of progressive temporal changes. However, for the above reasons we note that our interpretations based on the microfossils are made with caution.
The pre-occupation environment
The plant microfossil sequence at Cook's Cove commences in the basal shelly sand horizon with sea-rafted Taupo Pumice in the central section (b). Assuming that this pumice was deposited at the cove not long after the c. AD 200 eruption, this is well before human settlement of New Zealand. The unusually large amount of manuka/kanuka pollen (which is locally dispersed) in the lowermost sample indicates a probably dense stand of these early seral small trees and shrubs in the immediate vicinity of the sampled site. As this taxon commonly colonizes disturbed banks and plains of waterways, its presence probably reflects local, natural stream channel migration and storms. Alternatively or combined with this, it may be related to the disturbance event that deposited the totara logs into the estuary (see below).
As bracken is almost always associated with fire in New Zealand pollen spectra, its presence (along with microscopic charcoal fragments) in the pre-settlement deposits is probably a result of mixing due to percolation, bioturbation and mechanical disturbance (e.g. digging) by people, not unexpected given the evidence of intensive human activity at the site in deposits above this (see below) (a, b, d, e). This is likewise the case for hornwort spores, which are almost always associated with human presence in New Zealand pollen spectra of the last millenium, and for the direct indicators of Polynesian presence, namely microremains of cf. sweet potato and taro, also identified in the basal samples of all our profiles. Bracken, an invasive ground fern with widely dispersed spores, is often abundant in New Zealand pollen assemblages of the last two millennia. It may form dense stands, averaging 1–2 m tall, over extensive dryland areas. Although bracken is most commonly associated with large-scale, repeated burning of forest by early Maori, it is is also associated with extensive disturbance to vegetation following the Taupo eruption. The latter effect is thought to be due to a major, widespread increase in natural fires in the central North Island, with a large increase in fuel provided by dead and damaged trees, and is clearly seen in the Cook's Cove region in the pollen record from nearby Gisborne (Wilmshurst et al. Citation1999). Some of the bracken spores and charcoal in our basal samples are thus almost certainly from this source also. Although we would expect Cyathea (tree ferns) and ferns with monolete spores (ground ferns) to have been common in the Cook's Cove pre-settlement vegetation, the large amounts of their spores in some of the basal samples also reflect differential preservation; these types of fern spores are known to survive better than pollen grains in aerated deposits.
The origin and age of the array of totara logs (up to c. 90 cm diam.) recorded in Wellman's profile and still visible today in the intertidal zone at the inland end of the cove is uncertain. A sea-rafted origin seems unlikely; the cove entrance is narrow and to our knowledge similar deposits have to date not been found along the adjacent coast (Bruce McFadgen, pers. comm.). Although the Taupo eruption was one of the largest and most violent of the Holocene, the eruption centre (Lake Taupo) is too distant to have had a direct effect (e.g. blast shock wave) on local vegetation. As none of the logs has been reported in growth position, a logical explanation is that the trees were deposited onto the estuarine sands (into an environment suitable for wood preservation) from the surrounding (steep) slopes via a land slip. Slips caused by tree mass are not unexpected natural events. A possible, further contributing factor is that as the central eastern North Island is tectonically active, the slip may have been earthquake-triggered (Bruce McFadgen, pers. comm.). Wellman (Citation1962:46–47) described the logs as below Taupo Tephra, which is now dated to c. AD 200 (Lowe & de Lange Citation2000). However, a radiocarbon determination (NZ 651) reported to be on a sample that Wellman had collected from one of these trees (Pullar et al. Citation1977:715) gave the much younger age of 1111±41 BP (cal AD 2σ 889–1038). As totara is long-lived (many centuries), this determination may have considerable inbuilt age, which would make the true age of the event that displaced these trees even more recent. Information for this sample held in the New Zealand Radiocarbon Database (Citation2010) (NZRCDB) lists it as ‘wood from below Loisels Pumice. Sample in sandy silt with trees up to 2 ft, no standing trunks’. This leaves ambiguity as to whether the sample was taken directly from one of the trees or from other, associated wood. It also makes no mention of Taupo ash, instead relating the position of the trees to the much younger Loisels Pumice. Our investigations located neither ash nor pumice at the inland end of the cove, so we are unable to resolve these ambiguities or throw clearer light upon the age of the logs. Nonetheless, their disposition clearly reflects a localized disruption of forest during the pre-settlement era and may partly explain the microfossil pattern in our samples from below the lower occupation horizon.
The timing and effects of human occupation
Previous estimates of the date of initial human occupation at Cook's Cove (McFadgen Citation1982, Citation1994, Citation2007) were based on radiocarbon determinations on two further samples collected by Wellman. NZ631 (525±44 BP; cal AD 2σ 1392–1483), ‘stratified above Loisels Pumice in the lower occupation layer’ (NZRCDB), is on charcoal that was not identified to species, therefore is now removed from consideration of the age of human occupation according to discard protocols applied in New Zealand archaeology (Anderson Citation1991; Schmidt Citation2000). NZ632 (1008±60 BP; cal AD 2σ 1242–1462), also ‘from the lower occupation layer, stratified above Loisels Pumice’ (NZRCDB), is listed as ‘shell (unspecified)’ in the database, which would also remove it from consideration, but is reported as Lunella smaragda by Schmidt (Citation2000): table 8.2), who nonetheless still suspends it from consideration because it lacks a reliable charcoal pair.
The 2007 excavations yielded four radiocarbon dates for the lower occupation layer (Phase II), two each from its lower (layer 5b) and upper (layer 5a) components (Walter et al. 2011). Wk-23489 (844±33 BP; cal AD 2σ 1391–1621) on marine shell (Haliotis iris) and Wk-23490 (624±30 BP; cal AD 2σ 1308–1420) on moa bone date layer 5b. They overlap at 2σ in the period 1391–1420 which, on present evidence, provides the best estimate for the age of initial occupation at Cook's Cove. Layer 5a is dated by two samples of small-diameter charcoal from short-lived species: Wk-24846 (361±35 BP; Hebe sp., Plagianthus/Hoheria sp., Coprosma sp., Melicytus ramiflorus) and Wk-24847 (389±36 BP; Hebe sp., Plagianthus/ Hoheria sp., Pseudopanax arboreous, Pseudopanax crassifoloius, Melicyytus ramiflorus, Kunzea ericoides). These are statistically indistinguishable, and give a pooled mean age (Ward & Wilson Citation1978) of 375±25 BP (cal AD 2σ 1448–1631), strongly suggesting that the early occupation phase persisted until at least the mid fifteenth century.
The later phase of occupation (Phase IV) is not so precisely dated. There are two dates on fragments of Pahies australis and Austrovenus stutchburyi shell from the layer 3 garden soil, Wk-23487 (4542±40 BP) and Wk-23488 (4254±34 BP), which are interpreted as evidence for the use of ancient shell as a garden additive (Walter et al., 2011), as has been observed in other archaeological contexts (Furey Citation2006:50). Two further dates on small-diameter charcoal from short-lived species (Hebe sp., Coprosma sp., Plagianthus/Hoheria sp.) derive from the uppermost oven, dug from Layer 2. Wk-24844 (80±35 BP) and Wk-24845 (135±35 BP) are statistically indistinguishable and pool at 108±25 BP (cal AD 2σ 1683–1950). This is consistent with the presence of both European-introduced items (glass, metal and ceramic artefacts; pig and chicken bones) and pre-European artefact forms (stone flakes) in the upper component of layer 3, indicating that it probably derives from the late eighteenth or early nineteenth century. The lower components of layer 3 (3b and 3c) contained no historic period items, suggesting that the upper occupation began late in the prehistoric era.
Microfossil evidence of intensive human activity at the site is clear in the lower occupation layer (Phase II). This layer is charcoal-rich and has the highest concentrations of cf. sweet potato and taro starch (a,b). With numerous ovens and fire scoops in this layer along with postholes suggesting dwellings (Walter et al. 2011), it is clear that there was an occupation site on the area sampled in excavation units 1–3, making it likely that these plant remains reflect processing of crops on this part the site rather than intensive gardening here. Although disturbance of forest appears to have begun in the pre-settlement era, it was undoubtedly still present during the initial occupation, as land snails reliant on forest have been reported from a shell midden in the lower layer (McFadgen Citation1985).
Disturbance to the landscape during the occupation era is most strongly evident in the microfossil profiles from Phase III and above, with bracken spores recording very high values (). This is consistant with McFadgen's (1985) observation that land snails were not found in middens from the upper occupation layer. The common occurrence of extinct Brassospora pollen in the sections reflects regolith disruption of the hill slopes and incorporation of eroded material into the surfaces at lower elevations. Hill slope erosion is particularly apparent in P11, which has a 60 cm thick deposit of clay with abundant starch remains, suggesting swidden agriculture (b). Hornwort spores throughout the profiles in particular reflect intensive gardening at the site; this taxon has been shown to be associated with vegetation and soil disturbance by people and horticultural activity at sites elsewhere in New Zealand sites and beyond (Koelbloed & Kroeze Citation1965; Anchukaitis & Horn Citation2005; Horrocks et al. Citation2007b; Overland & Hjelle Citation2009). Pollen of the early seral taxon puha/dandelion generally throughout the sections also indicates human disturbance of the landscape.
The higher concentrations of cf. sweet potato starch remains compared to those of cf. taro in more samples suggest that the latter was not as important or as commonly used at the site (). This may be the case, although difficulty in identifying the tiny grains of taro starch and differential preservation are probably factors in this. As taro grains are near the limits of light microscopy, they are easily overlooked when scanning slides, and individual tiny grains may not be preserved for as long as large grains such as those of sweet potato. The low concentrations of starch in the oven features probably reflect a proportional effect due to the much higher concentrations of charcoal fragments in these samples.
Puha, an indigenous rosette herb of disturbed ground and also a source of edible shoots, may have been processed at the site along with the starch crops. Dandelion, closely related to puha, was introduced by Europeans and is a common weed of pasture and other disturbed areas. The high values of this pollen type in the uppermost samples reflect modern pasture, as does the high grass pollen in one of the P11 samples (at 38.5 cm depth, Phase IV/V) (). The high value of pollen of introduced broom/gorse (a local pollen disperser) in the subsequent P11 sample, from the matted layer of buried vegetation, indicates invasion of pasture in the immediate vicinity of the site by this taxon (common on the catchment hill slopes today). The large amount of pine pollen in the same sample indicates pine trees close by. The surficial layer of hill slope material with a small amount of starch remains covering this sample is probably storm-related. The March 1988 Cyclone Bola is a likely candidate; the East Coast region of the North Island was devastated by this, with three days of torrential rain resulting in catastrophic erosion and flooding.
Although early European accounts mention that ‘yams’ were grown at Cook's Cove (Beaglehole Citation1962, Citation1968), we did not find evidence of any introduced crops other than sweet potato and taro. Given the apparently high concentrations of cf. sweet potato starch grains in some of the samples, if yam (Dioscorea alata is the particular species reported being cultivated at first contact) had been intensively used at the site we would have expected to have found at least a few starch grains of this taxon, which has grains larger than sweet potato and distinctively shaped.
Evidence for marine inundation
Wellman (Citation1962:46) described the layer separating the two occupation horizons at Cook's Cove as a ‘shelly sand layer with many whole shells, not midden material’ and ‘not wind-blown; appears to be wave deposited’. Its position well above 1962 average storm waves suggested that it was the result of a higher sea-level or more likely a tsunami or a period of severe storms. His summary section shows the intervening layer comprising a lower ‘shelly soil’ component extending along almost half of his c. 230 m section at heights from c. 0.9–1.45 m above mean high water, and an upper ‘shelly sand’ component extending c. 95 m at heights of c. 1.35–1.8 m. Our observations on what remained of this shoreline in 2007 showed the shell layer was composed of fine shell fragments, never whole shells, within a matrix that varied from sandy to silty clay. This extended for no more than 35 m inland from a point approximately 5 m NE of our seaward section at heights of c. 0.55–1.0 m above mean high water at the seaward end, but thinning to c. 0.48–0.6 m in our central section, which is just before it disappears.
These differences are undoubtedly a result of erosion (extent unknown) since Wellman's (1962) study, and also show that the eroding face he recorded was higher than that in 2007, with the ground surface presumably decreasing in elevation further from the shore. We therefore assess Wellman's suggested origin of the shelly sand with caution.
Our examination of microfossils from the shelly sand (or any other deposits) found no evidence of marine dinoflagellate cysts. Where present, these are extracted along with pollen during sample preparation. Although pollen preservation is often poor in our sections and although the cysts are not ubiquitous, if there had been major sea inundations at the site we might have expected to have found these, as was the case in several terrestrial pollen profiles at Anaura Bay (Horrocks et al. Citation2008b). Additionally, the shelly sand in our central section and comparable Phase III deposits in the O11 and P11 profiles yielded relatively abundant pollen, spores and starch residues, strongly suggesting considerable terrestrial input in the formation of these layers. Furthermore, the O11 and P11 profiles show that this is primarily a colluvial deposit which has ancient shell mixed into it in places by gardening activity (Walter et al., in press). Also, we suggest that the shelly sand may have been spread over occupation surfaces by people to reduce mud from colluvium washed down from the steep slope immediately behind the sampled area. Evidence of catastrophic storm damage has been reported elsewhere in the Gisborne-East Coast region c. AD 1650 (Pullar & Penhale Citation1970).
Reinterpretation of the Holocene deposits
Since Wellman's (1962) pioneering work, geochronological models have played an increasingly important role in interpreting the history of human settlement in New Zealand. The c. AD 200 Taupo Tephra (Lowe et al. Citation2000), recognized as a significant isochronous benchmark well before human settlement, has assisted reconstruction of the immediate pre-settlement palaeoenvironment. The c. AD 1300 Kaharoa Tephra (Lowe et al. Citation1998; Buck et al. Citation2003) is highly significant as a stratigraphic marker because of its close proximity in age to the established age of initial human settlement, which most researchers consider to have occurred no earlier than AD 1250–1300 (e.g. Higham & Jones Citation2004; Wilmshurst et al. Citation2008). To date there is no unequivocal evidence of artefacts or culturally formed deposits beneath geochemically identified deposits of Kaharoa Tephra, and the earliest unequivocal palynological evidence for deforestation is immediately below this tephra, at c. AD 1280 (Newnham et al. Citation1998; Lowe et al. Citation2000, Citation2002). Wellman (Citation1962) suggested that the Loisels Pumice is ‘the key horizon for correlating archaeological and other sections on the east coast of the North Island’. However, as sea-rafted pumice deposits are subject to possible reworking, and as Loisels has since been shown to be from multiple sources and possibly deposited at different times (Shane et al. Citation1998), its use as a stratigraphic marker requires caution, and helps to explain observations of Loisels both above and below Kaharoa Tephra (Lowe et al. Citation2000). As well as tephras, sand dune sequences and tectonic uplift have provided useful geochronological models (e.g. McFadgen Citation1980, Citation1985, Citation1994, Citation2003a, Citationb). The great value of these models is that they allow correlation between sites.
Our results from Cook's Cove are consistent with these revised geochronological models, and differ in five significant respects to either the observations or interpretation of Wellman. (1) While Taupo Pumice is at the base of one of our profiles, below evidence of human presence (b), it was not as widespread as Wellman reported it, and in particular we did not observe it at the head of the inlet overlying the estuarine sand with totara logs. As noted above, the radiocarbon determination reportedly associated with these logs is not consistent with a pre-Taupo age. (2) Loisels Pumice was also restricted in distribution and, in the one profile where it was visible, occurred as sparse dispersed clasts (b), suggesting that it may not be a primary deposit. (3) While microscopic charcoal was present to the full depth of all our profiles, we did not observe macroscopic pieces below Loisels Pumice, as previously reported (Wellman Citation1962; McFadgen Citation1985). (4) The only material we could find that appeared to equate to the deposit tentatively identified by Wellman as Kaharoa Tephra appeared almost certainly not to be a tephra. (5) We found little evidence to support marine inundation as an explanation for the shelly sand layer separating the two occupation horizons.
Some of these differences reflect advances in tephra identification over the past c. 50 years, and others can be probably attributed to the removal of evidence by erosion. However, they have also arisen from the more detailed investigation of the site enabled by archaeological excavation and examination of its plant microfossils.
Acknowledgements
The project was funded by the New Zealand Lottery Grants Board (Environment and Heritage), New Zealand Historic Places Trust, the University of Otago and the Gisborne Branch Committee of New Zealand Historic Places Trust. The project was carried out in partnership with Te Aitanga a Hauiti. We thank Phil Shane for examining the putative Kaharoa Tephra.
References
- Anchukaitis , J and Horn , SP . 2005 . A 2000-year reconstruction of forest disturbance from southern Pacific Costa Rica . Palaeogeography, Palaeoclimatology, Palaeoecology , 221 : 35 – 54 .
- Anderson , AJ . 1991 . The chronology of colonization in New Zealand . Antiquity , 5 : 767 – 795 .
- Beaglehole JC 1 1962 . The Endeavour journal of Joseph Banks, 1768–1771 Sydney , Angus and Robertson.
- Beaglehole JC 1968 . The journals of Captain James Cook. The voyage of the Endeavour, 1768–1771 . CUP for the Hakluyt Society.
- Buck , C , Higham , TFG and Lowe , DJ . 2003 . Bayesian tools for tephrochronology . The Holocene , 13 : 639 – 647 .
- Dimbleby , G . 1985 . The palynology of archaeological sites , London : Academic Press .
- Higham TFG, Jones M 2004. Chronology and settlement. In: Furey L, Holdaway S ed. “Change through time: 50 years of New Zealand archaeology. Auckland, NZAA Monograph 26. Pp. 215–234.
- Furey , L . 2006 . Maori gardening: an archaeological perspective , Wellington : DOC .
- Horrocks , M . 2005 . A combined procedure for recovering phytoliths and starch residues from soils, sedimentary deposits and similar materials . Journal of Archaeological Science , 32 : 1169 – 1175 .
- Horrocks , M and Barber , I . 2005 . Microfossils of introduced starch cultigens from an early wetland ditch in New Zealand . Archaeology in Oceania , 40 : 106 – 114 .
- Horrocks , M and Rechtman , RB . 2009 . Sweet potato (Ipomoea batatas) and banana (Musa sp.) microfossils in deposits from the Kona Field System, Island of Hawaii . Journal of Archaeological Science , 36 : 1115 – 1126 .
- Horrocks , M and Wozniak , JA . 2008 . Plant microfossil analysis reveals disturbed forest and a mixed-crop, dryland production system at Te Niu, Easter Island . Journal of Archaeological Science , 35 : 126 – 142 .
- Horrocks , M , Campbell , M and Gumbley , W . 2007a . A short note on starch and xylem of Ipomoea batatas (sweet potato) in archaeological deposits from northern New Zealand . Journal of Archaeological Science , 34 : 1441 – 1448 .
- Horrocks , M , Nichol , SL , Augustinus , PC and Barber , IG . 2007b . Late Quaternary environments, vegetation and agriculture in northern New Zealand . Journal of Quaternary Science , 22 : 267 – 279 .
- Horrocks , M , Smith , IWG , Nichol , SL , Shane , PA and Jackman , G . 2008a . Field survey, sedimentology and plant microfossil analysis of sediment cores from possible cultivation sites at Tolaga Bay, eastern North Island, New Zealand . Journal of the Royal Society of New Zealand , 38 : 131 – 147 .
- Horrocks , M , Smith , IWG , Nichol , SL and Wallace , R . 2008b . Sediment, soil and plant microfossil analysis of Maori gardens at Anaura Bay, eastern North Island, New Zealand: comparison with descriptions made in 1769 by Captain Cook's expedition . Journal of Archaeological Science , 35 : 2446 – 2464 .
- Jones , KL . 1983a . An archaeologically defined ‘hamlet’ (N89-90/222) in inland Tolaga Bay . New Zealand Archaeological Association Newsletter , 26 : 7 – 20 .
- Jones , KL . 1983b . Joseph Banks' “fence of poles” at Cook's Cove, East Coast, North Island, New Zealand . Journal of the Polynesian Society , 92 : 531 – 536 .
- Jones , KL . 1986 . Polynesian settlement and horticulture in two eastern North Island river catchments . New Zealand Journal of Archaeology , 8 : 5 – 32 .
- Jones , KL . 1990 . Settlement chronology on river terrace landforms: a New Zealand case study . Geoarchaeology , 5 : 255 – 273 .
- Jones KL 2007. Horticultural complexes on stony soils of the eastern North Island: An aerial interpretation. In: Anderson A, Green K, Leach F ed. Vastly ingenious: the archaeology of Pacific material culture, in honour of Janet M. Davidson. Dunedin, Otago University Press. Pp. 97–116.
- Jones , KL and Law , RG . 1987 . Prehistoric population estimates for the Tolaga Bay vicinity, East Coast, North Island, New Zealand . New Zealand Journal of Archaeology , 9 : 81 – 114 .
- Koelbloed , KK and Kroeze , JM . 1965 . Anthoceros species as indicators of cultivation . Boor en Spade , 14 : 104 – 109 .
- Lowe , DJ and de Lange , WP . 2000 . Volcano-meteorological tsunamis, the c. AD 200 Taupo eruption (New Zealand) and the possibility of a global tsunami . The Holocene , 10 : 401 – 407 .
- Lowe , DJ , McFadgen , BG , Higham , TFG , Hogg , AG , Froggatt , PC and Nairn , IA . 1998 . Radiocarbon age of Kaharoa Tephra, a key marker for the late-Holocene stratigraphy and archaeology in New Zealand . The Holocene , 8 : 487 – 495 .
- Lowe , DJ , Newnham , RM , McFadgen , BG and Higham , TFG . 2000 . Tephras and New Zealand archaeology . Journal of Archaeological Science , 27 : 859 – 870 .
- Lowe DJ, Newnham RM, McCraw JD 2002. Volcanism and early Maori society in New Zealand. In: Torrence R, Grattan J ed. Natural disasters and cultural change. London, Routledge. Pp. 126–161.
- Loy , TH , Spriggs , M and Wickler , S . 1992 . Direct evidence for human use of plants 28,000 years ago: starch residues on stone artefacts from the northern Solomon Islands . Antiquity , 66 : 898 – 912 .
- McFadgen , BG . 1980 . Age relationship between a Maori plaggen soil and Moa-hunter sites on the west Wellington coast . New Zealand Journal of Geology and Geophysics , 23 : 249 – 256 .
- McFadgen , BG . 1982 . Dating New Zealand archaeology by radiocarbon . New Zealand Journal of Science , 25 : 379 – 392 .
- McFadgen , BG . 1985 . Late Holocene stratigraphy of coastal deposits between Auckland and Dunedin, New Zealand . Journal of the Royal Society of New Zealand , 15 : 27 – 65 .
- McFadgen BG 1994. Coastal stratigraphic evidence for human settlement. In: Sutton DG ed. The origins of the first New Zealanders. Auckland University Press. Pp. 195–207.
- McFadgen BG 2003a. Archaeology of the Wellington Conservancy: Wairarapa: A study in tectonic archaeology. Wellington, DOC.
- McFadgen BG 2003b. Pre-European archaeology of the coast. In: Goff JR, Nichol SL, Rouse HL ed. The New Zealand coast – Te tai o Aotearoa. Palmerston North, Dunmore Press. Pp. 265–296.
- McFadgen BG 2007. Hostile shores: catastrophic events in prehistoric New Zealand and their impact on Maori coastal communities. Auckland University Press.
- Mildenhall , DC and Byrami , ML . 2004 . A redescription of Podosporites parvus (Couper) Mildenhall emend. Mildenhall & Byrami from the Early Pleistocene, and late extinction of plant taxa in northern New Zealand . New Zealand Journal of Botany , 41 : 147 – 160 .
- Moore PD, Webb JA, Collinson ME 1991. Pollen analysis. 2nd edition. London, Blackwell Scientific.
- Newnham , RM , Lowe , DJ , McGlone , MS , Wilmshurst , JM and Higham , TFG . 1998 . The Kaharoa Tephra as a critical datum for earliest human impact in northern New Zealand . Journal of Archaeological Science , 25 : 533 – 544 .
- New Zealand Radiocarbon Database 2010. http://www.waikato.ac.nz/waikato/nzcd/.
- New Zealand Soil Bureau 1973. Map of parent rocks of New Zealand soils. 1:1,000,000. New Zealand Soil Survey Report 5.
- NIWA 2007. http://www.niwascience.co.nz/edu/resources/climate.
- Overland , A and Hjelle , KL . 2009 . From forest to open pastures and fields-cultural landscape development in western Norway inferred from two pollen records representing different spatial scales of vegetation . Vegetation History and Archaeobotany , 18 : 459 – 476 .
- Polack JS 1838. New Zealand. Being a narrative of travels and adventures during a residence in that country between the years 1831 and 1837. Volume II. London, Richard Bentley.
- Pullar , WA , Kohn , BP and Cox , JE . 1977 . Air-fall Kaharoa Ash and Taupo Pumice, and sea-rafted Loisels Pumice, Taupo Pumice, and Leigh Pumice in northern and eastern parts of the North Island, New Zealand . New Zealand Journal of Geology and Geophysics , 20 : 697 – 717 .
- Pullar , WA and Penhale , HR . 1970 . Periods of recent infilling of the Gisborne Plains Basin: associated marker beds and changes in shorelines . New Zealand Journal of Science , 20 : 49 – 53 .
- Schmidt MD 2000. Radiocarbon dating New Zealand prehistory using marine shell. Oxford, BAR International Series.
- Shane , P , Froggatt , P , Smith , I and Gregory , M . 1998 . Multiple sources for sea-rafted Loisels Pumice, New Zealand . Quaternary Research , 49 : 271 – 278 .
- Sunell , LA and Healey , PL . 1979 . Distribution of calcium oxylate crystals in corms of taro (Colocasia esculenta) . American Journal of Botany , 66 : 1029 – 1032 .
- Torrence R, Barton H ed 2006. Ancient starch research. Walnut Creek, CA, Left Coast Press.
- Walter R, Jacomb C, Brooks E 2011. Excavations at Cook's Cove, Tolaga Bay, New Zealand. Journal of Pacific Archaeology. 2: 1–28
- Ward , GK and Wilson , SR . 1978 . Procedures for comparing and combining radiocarbon age-determinations: a critique . Archaeometry , 20 : 19 – 31 .
- Wellman , HW . 1962 . Holocene of the North Island of New Zealand: a coastal reconnaissance . Transactions of the Royal Society of New Zealand , 1 : 29 – 99 .
- Wilmshurst , JM , Eden , DE and Froggatt , PC . 1999 . Late Holocene forest disturbance in Gisborne, New Zealand: a comparison of terrestrial and marine pollen records . New Zealand Journal of Botany , 37 : 523 – 540 .
- Wilmshurst , JM , Anderson , AG , Higham , TFG and Worthy , TH . 2008 . Dating the late prehistoric dispersal of Polynesians to New Zealand using the commensal Pacific rat . Proceedings of the National Academy of Sciences , 105 : 1676 – 1680 .
- Wright , IC , Gamble , JA and Shane , PA . 2003 . Submarine silicic volcanism of the Healy caldera, southern Kermadec arc (SW Pacific): 1 – volcanology and eruptive mechanisms . Bulletin of Volcanology , 65 : 15 – 29 .