Abstract
The genetic diversity of the 16S rRNA and ompA genes of Riemerella anatipestifer was investigated. A 16S rRNA gene-based PCR was able to amplify all 18 Taiwanese strains and 10 reference strains. The identity of 16S rRNA sequence of these strains and seven other sequences retrieved from GenBank was 95.0–100.0%. The percentage identity of the ompA sequence of the 15 Taiwanese strains and eight reference strains amplified in this study and two other sequences retrieved from GenBank was 88.1–100.0%. Phylogenetic analysis based on the 16S rRNA gene showed that all the R. anatipestifer strains fell into a single cluster. It is concluded that the 16S rRNA gene-based PCR is suitable for the screening of R. anatipestifer infections. Phylogenetic analysis of the ompA of R. anatipestifer resulted in three different clusters, while seven clusters were found when the derived amino acid sequence was the basis of analysis. No apparent cluster was found using the criteria of host, isolate serotype, the year or location of isolation.
Variation génétique des gènes ompA et ARNr 16S de Riemerella anatipestifer
Il a été étudié la diversité génétique des gènes ompA et ARNr 16S de Riemerella anatipestifer. Une PCR basée sur le gène ARNr 16S a été capable d'amplifier les 18 souches taiwanaises et les 10 souches de référence. L'identité de la séquence de l'ARNr 16S de ces souches et de sept autres séquences récupérées à la GenBank a été de 95,0–100%. Le pourcentage d'identité de la séquence ompA des 15 souches taiwanaises et des huit souches de référence amplifiées dans cette étude et de deux autres séquences récupérées à la GenBank a été de 88,1–100,%. L'analyse phylogénétique basée sur le gène ARNr 16S a montré que toutes les souches de R. anatipestifer appartenaient à un seul groupe. Il a été conclu que la PCR basée sur le gène ARNr 16S est appropriée au screening des infections à R. anatipestifer. L'analyse phylogénétique de l’ ompA de R. anatipestifer a abouti à trois groupes différents, alors que sept groupes ont été mis en évidence quand l'analyse a été basée sur la séquence dérivée en acides aminés. Aucun groupe évident n'a été trouvé en utilisant les critères : hôte, sérotype de la souche, année ou localisation de l'isolement.
Genetische Variation des ompA- und des 16SrRNS-Gens von Riemerella anatipestifer
Die genetische Vielfalt des 16SrRNS- und des ompA-Gens von Riemerella anatipestifer wurde untersucht. Eine auf dem 16SrRNS-Gen basierende PCR war in der Lage, alle 18 taiwanesischen Isolate sowie 10 Referenzstämme zu amplifizieren. Die Übereinstimmung der 16SrRNS-Sequenz dieser und sieben anderer von der Genbank abgerufenen Stämme betrug 95,0–100,0 %. Die auf dem 16SrRNS-Gen beruhende phylogenetische Analyse zeigte, dass alle diese R. anatipestifer-Stämme zu einem Cluster gehörten. Daraus wird geschlossen, dass die auf dem 16SrRNS-Gen basierende PCR für die Überprüfung auf R. anatipestifer-Infektionen geignet ist. Die phylogenetische Analyse des ompA von R. anatipestifer ließ drei verschiedene Cluster erkennen, während bei der Analyse der daraus resultierenden Aminosäuresequenzen sieben Cluster gefunden wurden. Bei dem Versuch der Einteilung hinsichtlich Wirtstier, Serotyp des Isolats, Jahr und Ort der Isolierung waren keine Cluster ersichtlich.
Variación genética de los genes ompA y 16S rARN de Riemerella anatipestifer
Se investigó la diversidad genética de los genes 16S rARN y ompA de Riemerella anatipestifer. Se utilizó una PCR basada en el 16S rRNA, con la cual fue posible amplificar 18 cepas Taiwanesas y 10 cepas de referencia. La identidad de la secuencias de 16S rARN de estas cepas y de otras siete cepas obtenidas del GenBank fue del 95.0–100.0%. El porcentaje de identidad de la secuencia de ompA de 15 cepas Taiwanesas y de ocho cepas de referencia amplificadas en este estudio y de otras dos secuencias obtenidas del GenBank fue del 88.1–100.0%. Los análisis filogenéticos basados en el gen 16S rARN mostraron que todas las cepas de R. anatipestifer se encontraban en un mismo grupo o cluster. Se concluye que la PCR basada en el gen 16S rARN es adecuada para la detección de infecciones por R. anatipestifer. Los análisis filogenéticos de ompA de R. anatipestifer resultaron en tres grupos diferentes, mientras que se formaron siete grupos cuando la secuencia de aminoácidos fue la base del análisis. No se formaron grupos o clusters utilizando los criterios de huésped, aislado, serotipo o año o localización del aislamiento.
Introduction
Riemerella anatipestifer infection is a serious worldwide problem of natural waterfowl and domestic turkey, duck, and goose populations (Glunder & Hinz, Citation1989; Sandhu, Citation2003). The infection typically exacts a severe economic cost, especially when poultry is also infected with other microorganisms. Once the disease invades duck and goose flocks, it can become endemic. Eradication can be difficult, with repeated infectious episodes possible.
Infections caused by R. anatipestifer are characterized by the systemic development of exudative serositic lesions, and should be differentiated from fowl cholera, and infections caused by Escherichia coli, Streptococcus faecium, and Salmonella spp. (Sandhu, Citation2003). Identification and typing of R. anatipestifer involves phenotypic, biochemical, and molecular characterization (Rimler & Nordholm, Citation1998; Huang et al., Citation1999).
At least 21 serotypes of R. anatipestifer have been isolated (Loh et al., Citation1992; Pathanasophon et al., Citation1995; Sandhu, Citation2003). Strong variations of virulence as assessed by mortality and morbidity rates in outbreaks have been reported between serotypes and within a given serotype (Brogden et al., Citation1982), consistent with a presumed high degree of genetic diversity among different isolates or strains.
The definitive diagnosis of R. anatipestifer infection requires isolation and identification of R. anatipestifer. R. anatipestifer is identified on the basis of growth, morphologic and biochemical characteristics. However, R. anatipestifer is characterized more by the absence than by the presence of specific phenotypic properties (Hinz et al., Citation1998). No selective and/or indicative media have been established for R. anatipestifer. Addition of gentamicin to culture media for the isolation of R. anatipestifer is not always successful in inhibiting the growth of other organisms present in the specimens (Rimler et al., Citation1998). A genetic diagnostic tool that can detect all the R. anatipestifer isolates or strains is obviously needed.
Recently, the predominant immunogenic outer membrane protein OmpA was characterized for its possible use in the serodetection of R. anatipestifer infections (Subramaniam et al., Citation2000). Use of 16S rRNA gene rrs has been successful in classifying R. anatipestifer as belonging to the Flavobacteriaceae in rRNA superfamily V (Segers et al., Citation1993; Subramaniam et al., Citation1997). However, the potential of ompA- or rrs-based diagnostic assays has been muted by a lack of information concerning the variation of these two genes. Indeed, a GenBank search for R. anatipestifer reveals only two ompA and seven 16S rRNA genes. The objectives of the present study were to examine the genetic diversity of 16S rRNA and ompA of R. anatipestifer, and to develop a PCR-based diagnostic test for this organism.
Materials and methods
Bacterial strains and genomic DNA extraction
The R. anatipestifer strains included 18 Taiwanese field isolates () and 10 serotype reference strains (TRa1-TRa10; a gift of Dr. Chao-Fu Chang, Department of Veterinary Medicine, National Taiwan University). Serotype specific rabbit antiserum was produced against each reference strain according to the methods described by Rimler et al. (Citation1998). The serotype of the Taiwanese field isolates was determined by the standard plate agglutination methods using the serotype specific antisera. The strains were grown on trypticase soy agar (Merck & Co., Whitehouse Station, NJ) supplemented with 5% sheep blood at 37°C for 24 h in an atmosphere containing 5% CO2. Genomic DNA was extracted using the QIAamp kit (Qiagen, Valencia, CA). The concentration and quality of the genomic DNA were established by optical density measurements at 260 and 280nm.
Table 1. The characteristics of the 18 Riemerella anatipestifer Taiwanese field strains and 10 serotype reference strains used in this study
Specific 16S rRNA gene- based PCR development
The 16S rRNA gene sequences of 10 species of Flavobacteriaceae obtained from GenBank included Capnocytophaga haemolytica (X97247 and U41349), Cellulophaga lytica (AB032513 and D12666), Coenonia anatine (AF118419 and Y17612), Flavobacterium salegens (M92279), Gelidibacter mesophilus (AJ344134 and AJ344133), Tenacibaculum cellulophagum (AF469612), Weeksella virosa (M93152), Zobellia galactanovora (AF208293), Riemerella anatipestifer (U60101, U60102, U60103, AF118416 and AF118417) and Riemerella columbina (AF181448). The sequences were aligned and analyzed by the clustal method of the Laser gene MegAlign II program (DNAStar, Madison, WI). Two primers based on the conserved region of the 16S rRNA gene were designed and used to carry out the specific PCR. The sense primer was RA20F2 (5′-CAGCTTAACTGTAGAACTGC-3′) and the antisense primer was RA20R4 (5′-TCGAGATTTGCATCACTTCG-3′). The expected PCR product size was 662 bp. The PCR procedure was also modified by the gradient annealing temperature program described above as a means of checking the best annealing condition. The formula of the reaction mixture used was the same as that of the ompA PCR. The PCR amplification programs consisted of 35 cycles of 94°C for 30 seconds, annealing temperature for 30 seconds, and 74°C for 1 min. The annealing temperatures ascended from 58.9°C to 68.2°C.
Sensitivity and specificity of the 16S rRNA gene- based PCR
Sensitivity of the PCR procedure was evaluated by using serially diluted amounts of genomic DNA obtained from R. anatipestifer strain PT9101-G1, and by the extraction of genomic DNA in specific colony forming units (CFU) of bacteria. PCR specificity was ascertained using three isolates each of Escherichia coli and Pasteurella multocida obtained from Taiwanese ducks, the Ornithobacterium rhinotracheale reference strain ATCC 51464, and genomic DNA extracted from the trachea, liver, spleen, kidney, lung, intestine, brain, and meninges of healthy two-week-old ducklings.
16S rRNA sequence analyses
Genomic DNA was extracted from all the R. anatipestifer strains or isolates, and the dsDNA was adjusted to 100 ng/μl prior to the specific PCR. The PCR product was column purified (Microcon 100; Amicon, Beverly, Mass.), and cycle sequencing was subsequently performed with the Big Dye terminator kit (Perkin-Elmer Applied Biosystems, Foster City, CA) as recommended by the manufacturer on a model 9700 thermocycler with the preprogrammed Big Dye cycle sequencing parameters on the instrument. The sequences of the PCR products were compiled, and sequence comparison and alignments conducted using the SeqMan program (DNAStar, Madison, WI). Clustal multiple alignment algorithms were used to calculate the similarity and divergence of the sequences. The NJ phylogenetic tree was generated using the Molecular Evolutionary Genetics Analysis (MEGA) program (version 2.1; available at http://www.megasoftware.net) with 10,000 bootstrap replicates. If the bootstrap value of a branching point was smaller than 70%, bootstrap values were re-calculated by the quartet puzzling method with the HKY substitution model for nucleotide sequences (Hasegawa et al., Citation1985) and the JTT substitution model for amino acid sequences (Jones et al., Citation1992). Eight other R. anatipestifer 16s rRNA gene sequences (strain 4005, AF118416; strain 4340, AF118417; strain 4280, AF118418; strain ATCC11845T (T=Type strain), U60101; strain 389/89, U60102; strain 743/85, U60103; and strain 30/90, U60104), and one R. columbina 16s rRNA gene sequence (AF181448) obtained from Genbank were also included in the analysis.
OmpA sequence analyses
Two primers were designed and used to carry out the polymerase chain reaction (PCR) for R. anatipestifer (data not shown). The designation of the primers was based on the conserved region of the ompA sequences derived from strains ATCC11845 (accession number AF104937) and CVL110/89 (AF104936) (Subramaniam et al. Citation2000). Only fifteen of the 18 Taiwanese strains and eight of the 10 reference strains could be amplified by the PCR. Those strains that could not be detected by the PCR included two reference strains (TRa 4 and TRa 8) and three Taiwanese field strains (PT0002-G1, TY8301-G, and PT-9404-D) (data not shown). The PCR products of the 15 Taiwanese and eight reference strains were sequenced and analysed as per the methods described in the section of 16S rRNA sequence analyses.
Results
Specific 16S rRNA gene PCR development
Among the annealing temperatures tested, 67.2°C produced the best result and was subsequently adopted for the specific PCR for R. anatipestifer identification.
All 18 Taiwan and 10 reference strains were successfully amplified with a 665 bp DNA product (data not shown). The detection limit of the PCR was 0.6 pg/ml of R. anatipestifer genomic DNA template and the genomic DNA extracted from 350 CFU of R. anatipestifer (). PCR did not amplify genomic DNA extracted from E. coli, P. multocida, O. rhinotracheale and organ emulsions from healthy ducklings.
Figure 1. PCR of 10 fold serial diluted Riemerella anatipestifer DNA templete using 16rrs gene primers 16rrs-5′-2 and 16rrs-3′-4. Left Figure: Lane M, marker; Lane 1, 6×104 pg/ml; Lane 2, 6×103 pg/ml; Lane 3, 6×102 pg/ml; Lane 4, 6×101 pg/ml; Lane 5, 6×100 pg/ml; Lane 6, 6×10−1 pg/ml; Lane 7, 6×10−2 pg/ml. Right Figure: Lane M, marker; Lane 1, 3.5×107 cfu; Lane 2, 3.5×107 cfu; Lane 3, 3.5×107 cfu; Lane 4, 3.5×107 cfu; Lane 5, 3.5×107 cfu; Lane 6, 3.5×107 cfu; Lane 7, 3.5×107 cfu; Lane 8, negative control.
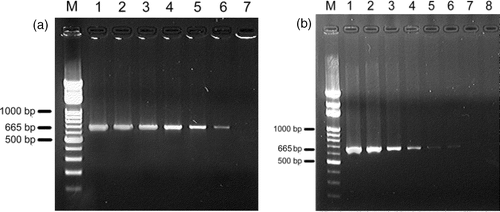
16S rRNA sequence analysis
Using the RA20F2 and RA20R4 primer set, the 665 bp PCR products of the 18 Taiwanese strains and 10 reference strains were sequenced and submitted to GenBank. The assigned accession numbers are shown in . These sequences were aligned and compared with 16S rRNA sequences of seven other R. anatipestifer strains and one R. columbina strain retrieved from GenBank. The gene identity of R. anatipestifer was 95.0%–100% among all R. anatipestifer strains, with a divergence of only 0–4.4% (data not shown). Among all other R. anatipestifer strains the largest divergence (4.4%, representing a 26 nt difference) was found between TRa1 and TRa7. Divergence of R. columbina to the R. anatipestifer strains was 2.3–5.6%. Phylogenetic tree lineage based on the 16S rRNA gene sequences did not support the existence of different lineages or serotypes in the strains. In addition, the R. anatipestifer strains did not cluster in the phylogenetic tree lineage according to the time or the location of isolation. After removing six shorter sequences (TRa10, AY 612173; TRa4, AY 612182; TW9401, AY 612186; TW9601-5, AY 612187;TRa9, AY612184; TW9001, AY612185) another phylogenetic analysis based on the 1250 bp obtained the similar result ().
Figure 2. Phylogenetic tree based on the nucleotide sequence of PCR products amplified from the 16S rRNA gene of Riemerella anatipestifer strains or related isolates. Numbers at branching points represent the percentage of 10,000 bootstrap values calculated by the MEGA program with the Kimura 2-parameter distance optional for nucleotide sequences. Scale bar indicates the number of nucleotide substitutions per site.
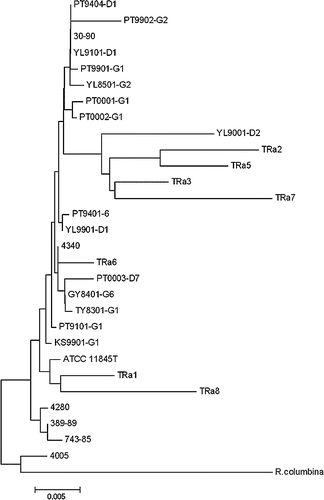
Omp A sequence analysis
The 1164 bp PCR products of the 15 Taiwanese and eight reference strains were sequenced and submitted to GenBank. The assigned accession numbers of Taiwanese strains are summarized in . The 23 sequences were aligned with the two available sequences (AF104936 and AF 104937) obtained from GenBank. The similarity of the nucleotide sequences of these strains was 88.1–100.0%, and the divergence was 0–13.0% (data not shown). The largest nucleotide sequence divergence was found between strain KS9901-G and the serotype-2 reference strain TRa2. Most of the variation occurred between nucleotides 415 and 804. When the nucleotide sequences of these R. anatipestifer strains was transformed into the corresponding amino acid sequences, aligned, and compared, the identity was 89.1–100%. The largest divergence (12.1%) was found between strain YL9101-D1 and TRa2. Of 387 amino acid residues, 68 (17.6%) showed variation among different strains. Variation in 32 residues only appeared in one strain, another 36 residues showed variation in multiple strains. About 92% (33/36) of this variations occurred between amino acid residues 141–268, especially in two hypervariable regions. Region A comprised amino acids 188–208 (10 variations/21 residues) and region B comprised amino acids 229–268 (16 variations/40 residues) ( ).
Figure 3. Alignment of the amino acid sequences of ompA of the Riemerella anatipestifer strains using the Clustal method with weighted residue weight table. Only residues different to the majority are shown.
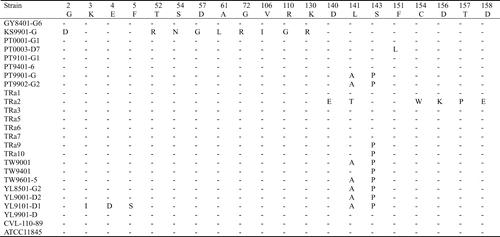
Phylogenetic tree analysis of the ompA nucleotide sequences clustered the R. anatipestifer strains into three genogroups (). Genogroup I contained 13 strains, genogroup II was comprised of 10 strains, and genogroup III contained two strains (CVL-110.89 and KS9901-G). Strains of the same serotype or host could be found in both groups. As well, both the Taiwanese and other strains could be grouped together ().
Figure 4. The neighbour-joining phylogenetic tree based on the nucleotide sequence of PCR products amplified from ompA of Riemerella anatipestifer strains or related isolates. Numbers at branching points represent the percentage of 10,000 bootstrap values calculated by the MEGA program with the Kimura 2-parameter distance optional for nucleotide sequences. The bootstrap values within brackets were calculated by the quartet puzzling method with the HKY substitution model for nucleotide sequences. Scale bar indicates the number of nucleotide substitutions per site.
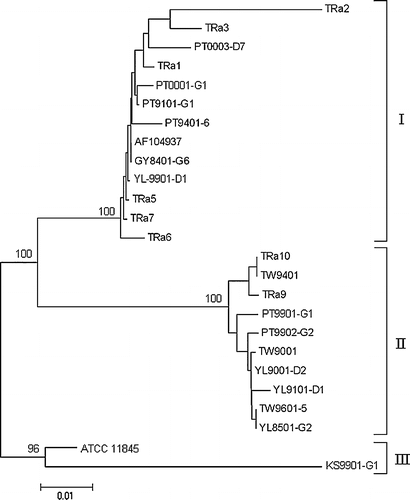
When the phylogenetic tree was constructed according to the amino acid sequence of OmpA, the R. anatipestifer strains or isolates formed seven clusters. Strains in the genogroup I were further divided into a five amino acid sequence cluster (III–VII), while strains of genogroup II and III still each formed a single cluster (I and II) (). No apparent pattern related to the cluster was found.
Figure 5. Phylogenetic tree based on the deduced amino acid sequence of PCR products amplified from ompA of Riemerella anatipestifer strains or related isolates. Numbers at branching points represent percentage of 10,000 bootstrap values calculated by the MEGA program with the Poisson correction distance optional for amino acid sequences. The bootstrap values within brackets were calculated by the quartet puzzling method with the JTT substitution model for amino acid sequences. Scale bar indicates the number of amino acid substitutions per site.
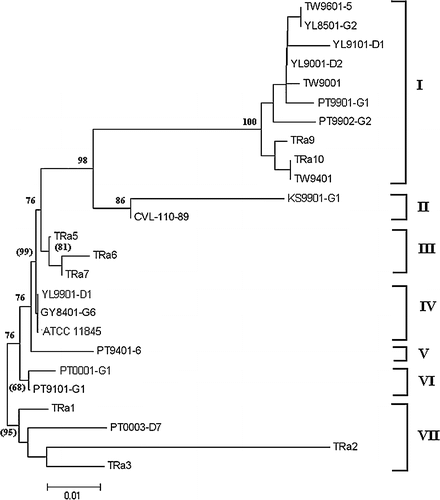
Discussion
The 16S rRNA gene is considered a stable fragment on the chromosome of all procaryotes. This gene is typically applied to the determination of phylogenetic relationship among bacteria. The benefits of using 16S rRNA sequence comparison for phylogenetic analysis and classification are that 16S rRNA is highly conserved among all organisms due to the antiquity of the protein-synthesizing process. Only one type of ribosome exists and the molecule is functionally constant and moderately well conserved across broad phylogenetic distances. The number of informative sequence positions makes ribosomal RNA an excellent molecule for comparison of various hierarchial levels and the analysis can be performed at the level of both rRNA and DNA (DeLong et al., Citation1989; Olsen et al., Citation1994). Based on 16S rRNA gene sequence analyses, R. anatipestifer belongs to the family Flavobacteriaceae in rRNA superfamily V (Subramaniam et al., Citation1997). Divergences in the 1402 bps of 16S rRNA gene sequences among four R. anatipestifer strains (accession numbers U60101–U60104) are small, being within 14 nt (0.5–1%) (Subramaniam et al., Citation1997).
Presently, the divergences within a 665 bps of 16S rRNA gene sequences among 18 Taiwan R. anatipestifer isolates and the sequences retrieved from GenBank are only 0–4.4%. Among them two serotypes (TRa7 and TRa 8) have up to a 26 nt difference to other R. anatipestifer strains. Ryll et al. (Citation2001) showed 99% sequence similarity between two R. anatipestifer and six R. anatipestifer-like strains. When four published sequences were included in the analysis, the similarity became 98–99%. The increased 16S rRNA sequence information gained in this study makes it clear that the 16S rRNA genes of R. anatipestifer are more varied than previously known. However, this heterogeneity does not alter the phylogenetic relationship of the R. anatipestifer strains, when compared to other bacteria (data not shown). In a notable exception, the divergence of the sequence of ATCC strain 11845 (accession number U10877) provided by Chua (1994) (http://www.ncbi.nlm.nih.gov/entrez/viewer.fcgi?db=nucleotide&val=506692) reached 13.4–17%. The sequence data of ATCC 11845T (T= type strain, accession number U60101) reveals a divergence of only 0.5–3.5%, rendering the 16S rRNA sequence data of U10877suspicious. Hence the sequence U10877 was excluded in the analysis. Similar findings on sequence data of U10877 have been described by Ryll et al. (Citation2001).
The sequence of primers RA20F2 and RA20R4 has only a single nt difference to PT-0001-G and YL8501-G2. Nonetheless, their use proved to be useful for the detection of all the Taiwanese field isolates and references strains tested. Also, a few other bacteria species tested in this study were all negative by PCR. However, the number of strains tested of R. anatipestifer and other species is still limited in number, thus more tests might need to prove the usefulness of the diagnostic PCR. The species we listed are the only organisms of Flavobacteriaceae for which the 16S rRNA sequences are available in GenBank. This criterion of primer design should be appropriate. After using the BLAST tool on the NCBI website (http://www.ncbi.nlm.nih.gov/BLAST/) to search for similar sequences, the results revealed the specificity of the primers was very high. But, it has been shown that although 16S rRNA sequencing can be used routinely to distinguish and establish relationships between genera and well-resolved species, very recently diverged species may not be recognizable (Fox et al., Citation1992). Judging from the near identical primer sequences, PCR would not be able to differentiate R. anatipestifer and R. columbina. Thus, PCR might also be useful for the screening of R. columbina infections in pigeons.
Outer membrane proteins generally play an important role in virulence and induce a strong antibody response (Puohiniemi et al., Citation1990; Weiser & Gotschlich, Citation1991). In concert with a previous report (Subramaniam et al., Citation2000) we found that most of the sequence variation of ompA was localized to the N terminus. Some of the R. anatipestifer isolates or strains could not be detected by this study. An explanation may lie with the limited information available at the time of primer design. Now, with the information gained from the determination of 23 additional sequences, the use of PCR incorporating a primer designed at the conserved region flanking the variable region encompassing nt 421–792 might well be useful for the diagnosis of avian R. anatipestifer infections and the study of the genetic variation of OmpA. It has been reported previously that sequence variations of ompA do not necessarily alter antigenic characteristics (Subramaniam et al., Citation2000). Consistent with this, we found presently that R. anatipestifer strains or isolates of the same serotype are found in different genogroups. Although OmpA protein has been suggested to play an important role in host adaptation (Davies and Lee, Citation2004), we found R. anatipestifer strains of the same host (duck or goose) in different genogroups. No cluster of R. anatipestifer strains of the same place or year of isolation was noted. Subramaniam et al. (Citation2000) have suggested that OmpA is valuable for the serodetection of R. anatipestifer infections, independent of their serotypes. However, judging from our present finding of a greater variation of ompA, the diagnostic value of the protein product is unclear. Clearly, the significance of classifying R. anatipestifer based on genetic or amino acid sequences requires further study.
The authors would like to thank Dr. Chao-Fu Chang at National Taiwan University for providing the reference strains. This study was supported by grants 88-ST-1.3-BQ-03 (4) and 89-BT-2.2-BQ-70 from Council of Agricultural, Taiwan, ROC.
References
- Brogden , KA , Rhoades , KR and Rimler , RB . (1982) . Serologic types and physiologic characteristics of 46 avian Pasteurella anatipestifer cultures . Avian Diseases , 26 : 891 – 896 .
- Davies , RL and Lee , I . (2004) . Sequence diversity and molecular evolution of the heat-modifiable outer membrane protein gene (ompA) of Mannheimia (Pasteurella) haemolytica, Mannheimia glucosida, and Pasteurella trehalosi . Journal of Bacteriology , 186 : 5741 – 5752 .
- DeLong , EF , Wickham , GS and Pace , NR . (1989) . Phylogenetic stains: ribosomal RNA-based probes for the identification of single cells . Science , 243 : 1360 – 1363 .
- Fox , GE , Wisotzkey , JD and Jurstshuk , P . (1992) . How close is close: 16S rRNA sequence identity may not be sufficient to guarantee species identity . International Journal of Systematic Bacteriology , 42 : 166 – 170 .
- Glunder , G and Hinz , KH . (1989) . Isolation of Moraxella anatipestifer from embryonated goose eggs . Avian Pathology , 18 : 351 – 355 .
- Hasegawa , M , Kishino , H and Yano , T . (1985) . Dating of the human-ape splitting by a molecular clock of mitochondrial DNA . Journal of Molecular Evolution , 22 : 160 – 174 .
- Hinz , K-H , Ryll , M , Kohler , B and Glunder , G . (1998) . Phenotypic characteristics of Riemerella anatipestifer and similar micro-organisms from various hosts . Avian Pathology , 27 : 33 – 42 .
- Huang , B , Subramaniam , S , Chua , KL , Kwang , J , Loh , H , Frey , J and Tan , HM . (1999) . Molecular fingerprinting of Riemerella anatipestifer by repetitive sequence PCR . Veterinary Microbiology , 67 : 213 – 219 .
- Jones , DT , Taylor , WR and Thornton , JM . (1992) . The rapid generation of mutation data matrices from protein sequences . Computer Applications in the Biosciences , 8 : 275 – 282 .
- Loh , H , Teo , TP and Tan , HC . (1992) . Serotype of Pasteurella anatipestifer isolate from ducks in Singapore: a proposal of new serotype . Avian Pathology , 21 : 453 – 459 .
- Olsen , GJ , Woese , CR and Overbeek , R . (1994) . The winds of (evolutionary) change: breathing new life into microbiology . Journal of Bacteriology , 176 : 1 – 6 .
- Pathanasophon , P , Sawada , T and Tanticharoneyos , T . (1995) . New serotypes of Riemerella anatipestifer isolated from ducks in Thailand . Avian Diseases , 24 : 195 – 199 .
- Puohiniemi , R , Karvonen , M , Vuopio-Varjuka , J , Muotiala , A , Helander , IM and Sarvas , M . (1990) . A strong antibody response to the periplasmic C-terminal domain of the OmpA protein of Escherichia coli is produced by immunization with purified OmpA or with whole E. coli or Salmonella typhimurium bacteria . Infection and Immunity , 58 : 1691 – 1696 .
- Rimler RB Sandhu TS Glisson JR (1998) Riemerella anatipestifer infection In D.E. Swayne, J.R. Glisson, M.W. Jackwood, J.E. Pearson & W.M. Reed (Eds.) A Laboratory Manual for the Isolation and Identification of Avian Pathogens 4th edn (pp. 22–23) Kennett Square PA The American Association of Avian Pathologists
- Rimler , RB and Nordholm , GE . (1998) . DNA fingerprinting of Riemerella anatipestifer . Avian Diseases , 42 : 101 – 105 .
- Ryll , M , Christensen , H , Bisgaard , M , Christensen , J-P , Hinz , K-H and Kohler , B . (2001) . Studies on the prevalence of Riemerella anatipestifer in the upper respiratory tract of clinically healthy ducklings and charaterization of untypable strains . Journal of Veterinary Medicine Series B , 48 : 537 – 546 .
- Sandhu TS (2003) Riemerella anatipestifer infection In Y.M. Saif, H.J. Barnes, J.R. Glisson, A.M. Fadly, L.R. McDougald & D.E. Swayne (Eds.) Diseases of Poultry 11th edn (pp. 676–682) Ames Iowa State University Press
- Segers , P , Mannheim , W , Vancanneyt , M , De Brandt , K , Hinz , KH , Kersters , K and Vandamme , P . (1993) . Riemerella anatipestifer gen. nov. comb. nov., the causative agent of septicemia anserum exsudative, and its phylogenetic affiliation within the Flavobacterium-Cytophaga rRNA homology group . International Journal of Systematic Bacteriology , 43 : 768 – 776 .
- Subramaniam , S , Chua , KL , Tan , HM , Loh , H , Kuhnert , P and Frey , J . (1997) . Phylogenetic position of Riemerella anatipestifer based on 16S rRNA gene sequences . International Journal of Systematic Bacteriology , 47 : 562 – 565 .
- Subramaniam , S , Huang , B , Loh , H , Kwang , J , Tan , HM , Chua , KL and Frey , J . (2000) . Characterization of a predominant immunogenic outer membrane protein of Riemerella anatipestifer . Clinical & Diagnostic Laboratory Immunology , 7 : 168 – 174 .
- Weiser , JN and Gotschlich , EC . (1991) . Outer membrane protein A (OmpA) contributes to serum resistance and pathogenicity of Escherichia coli K-1 . Infection and Immunity , 59 : 2252 – 2258 .