Abstract
To investigate the adaptation of avian infectious bronchitis virus (IBV) in a human cell line may be beneficial to understanding the potential mechanisms of coronavirus interspecies infection. The current study addressed the poor replication of IBV in the HeLa human cell line demonstrated in previous reports. We showed that IBV strains M41, H52, H120 and Gray could be propagated in HeLa cells with distinct cytopathic effect. The virus titre in freshly dispersed HeLa cells was 1000-fold higher than in cell monolayers. Trypsin was not the determinant for the viral replication, suggesting that the restriction of IBV replication in HeLa cells is the result of intracellular events rather than the binding to or fusion with host cells. These IBV strains replicated to an average titre of 103.4±0.2/0.1 ml median tissue culture infectious doses in freshly dispersed HeLa cells and maintained this titre for the first 12 passages. Then an approximately 10-fold increase (104.20±0.19/0.1 ml) occurred in passage 13, which was maintained to passage 16, after which there was another, bigger rise to 106.6±0.3/0.1 ml in passage 17. This titre was maintained until passage 24 when the experiment was terminated. The IBV M41 S1 gene was amplified and sequenced for passages 0, 5 and 21. There was only one amino acid replacement in the S1 protein, in passage 21. The presence of sialic acid on HeLa cells contributed to efficient virus replication, while human aminopeptidase N was not involved in the infection. Haemagglutinin activity gradually reduced with increased passages. These results indicated that the virus adaptation would probably be determined by host cell modification such as receptor glycosylation and different receptor utilization instead of viral gene mutation.
L'infection des cellules HeLa par le virus de la bronchite infectieuse aviaire dépend du statut cellulaire
L'étude de l'adaptation du virus de la bronchite infectieuse aviaire (IBV) à une lignée cellulaire humaine peut être favorable à la compréhension du mécanisme possible de l'infection inter espèce du coronavirus. Cette étude a abordé les difficultés de la réplication de l'IBV sur la lignée humaine HeLa démontré dans des rapports antérieurs. Plusieurs souches d'IBV M41, H52, H120 et Gray ont pu se répliquer sur HeLa avec un effet cytopathogène (CPE) net. Le titre du virus dans les cellules fraîchement dispersées a été 1000 fois supérieur à celui dans les cellules sous forme de tapis. La trypsine n'est pas déterminante pour la réplication virale suggérant que la restriction de la réplication de l'IBV dans les cellules HeLa est le résultat d'événements intracellulaires plutôt que la fixation aux cellules de l'hôte. Le titre du virus dans les cellules HeLa fraîchement dispersées a été de 103,4±0.2/0,1ml TCID50, ce titre s'est maintenu au cours des 12 premiers passages. Puis l'augmentation du titre d'environ un log (104,20±0,19/0,1ml) est apparue au 13ème passage, ce nouveau titre s'est maintenu du 13ème au 16ème passage, suivi d'une autre augmentation plus importante pour atteindre 106,6±0.3/0,1ml au 17ème passage et ce jusqu'au 24ème passage à la fin de l'expérimentation. Le gène S1 de l'IBV a été amplifié et séquencé pour les passages 0, 5 et 21. Il y a seulement le remplacement d'un acide aminé pour le gène S1 au passage 21. La présence de l'acide sialique dans les cellules HeLa a contribué à une réplication efficace du virus, alors que l'aminopepdidase N humaine n'a pas été impliquée dans l'infection. L'activité HA a été graduellement réduite au fur et à mesure des passages. Ces résultats ont montré que l'adaptation du virus serait probablement déterminée par la modification de la cellule hôte comme la glycosilation du récepteur et l'utilisation de récepteur différent plutôt que de la mutation du gène viral.
Infektion von Hela-Zellen mit dem Virus der infektiösen Bronchitis ist abhängig vom Zellstadium
Die Untersuchung der Adaptation von aviärem infektiösen Bronchitis Virus (IBV) an eine humane Zelllinie kann förderlich sein für das Verständnis des möglichen Mechanismus der Interspezies-Coronavirusinfektion. Die vorliegende Studie befasst sich mit der in der Literatur beschriebenen Schwierigkeit der IBV-Replikation in der humanen HeLa-Zelllinie. Mehrere IBV-Stämme (M41, H52, H120 und Gray) konnten in Verbindung mit einem deutlichen zytopathischen Effekt in den HeLa-Zellen vermehrt werden. In frisch ausgesäten HeLa-Zellen war der Virustiter 1000 Mal höher als im Zellmonolayer. Da Trypsin nicht der determinierende Faktor für die Virusvermehrung ist, läßt sich vermuten, dass die Begrenzung der IBV-Virusreplikation in HeLa-Zellen eher auf intrazelluläre Vorgänge als auf die Bindung an die Wirtszelle zurückzuführen ist. In frisch ausgesäten HeLa-Zellen erreichte das Virus einen Titer von 103,4±0,2KID50/0,1 ml und behielt diesen Titer über die ersten 12 Passagen. In der 13. Passage erfolgte ein zehnfacher Titeranstieg (104,2±0,19KID50/0,1 ml), der auf diesem Niveau von Passage 13 bis 16 blieb, gefolgt von einem stärkeren Titeranstieg auf 106,6±0,3KID50/0,1 ml in der 17. Passage. Dieser Titer wurde bis zur 24. Passage, nach der das Experiment beendet wurde, gehalten. Das S1-Gen des IBV aus den Passagen 0, 5 und 21 wurde amplifiziert und sequenziert. Dabei wurde in der 21. Passage nur ein Nukleinsäureaustausch im S1-Gen festgestellt. Das Vorkommen von Sialinsäure auf den HeLa-Zellen trug wirksam zur Virusvermehrung bei, während die humane Aminopeptidase N nicht am Infektionsgeschehen beteiligt war. Die Hämagglutinationsaktivität nahm mit zunehmender Passagezahl graduell ab. Diese Ergebnisse zeigen, dass die Virusadaptation wahrscheinlich durch eine Wirtszelländerung wie die Rezeptorglykosilierung und geänderte Rezeptornutzung und nicht durch virale Genmutation bestimmt wird.
La infección de células HeLa con virus de bronquitis infecciosa aviar es dependiente del estado celular
Para estudiar la adaptación del virus de bronquitis infecciosa aviar (IBV) en líneas celulares humanas sería beneficioso entender el posible mecanismo que determina la infección interespecie de los coronavirus. El presente estudio se centra en la dificultad de la replicación de IBV en la línea celular humana HeLa, previamente demostrada en otros artículos. Varias cepas de IBV M41, H52, H120 y Gray pudieron propagarse en HeLa con distintos efectos citopáticos (CPE). El título vírico en células HeLa frescas disgregadas fue 1000 veces mayor que en las monocapas celulares. La tripsina no es el determinante de la replicación vírica lo cual sugiere que la restricción de la replicación de IBV en HeLa es el resultado de eventos que suceden intracelularmente y no durante la unión del virus a las células del huésped. El título vírico en células HeLa frescas disgregadas fue de TCID50 de 103.4±0.2/0.1ml y se mantuvo durante los primeros 12 pases. Entonces, el título se incrementó 10 veces (104.20±0.19/0.1ml) en el pase 13 y este nuevo nivel se mantuvo entre los pases 13 al 16, seguido de otro incremento mayor hasta 106.6±0.3/0.1ml en el pase 17 y que ya se mantuvo hasta el pase 24 cuando el ensayo terminó. Se amplificó y secuenció el gen S1 de IBV en los pases 0, 5 y 21. Tan sólo se observó una sustitución aminoacídica en el gen S1 en el pase 21. La presencia de ácido siálico en células HeLa contribuyó a la replicación efectiva del virus, mientras que la aminopeptidasa N humana no estuvo involucrada en la infección. La actividad HA se redujo de manera gradual a medida que los pases aumentaron. Estos resultados indicaron que la adaptación del virus podría estar determinada por modificaciones de la célula del huésped como la glicosilación del receptor y por el uso de distintos receptores y no por mutaciones de genes víricos.
Introduction
Coronaviruses recently caused great attention because the aetiologic agent of severe acute respiratory syndrome was determined to be a new coronavirus, designated as severe acute respiratory syndrome-coronavirus. The epidemiological evidence supports an animal origin of the virus and leads to particular concern about coronaviruses breaking host–species barriers and becoming zoonotic (Kan et al., Citation2005).
Avian infectious bronchitis virus (IBV) is the prototype member of Coronaviridae (order Nidovirales, genus Coronavirus) (Cavanagh, Citation2001). It is a highly infectious pathogen of the domestic fowl, has over 25 serotypes, and replicates primarily in the chicken respiratory tract (Cavanagh, Citation2003). IBV, like other coronaviruses, displays limited host range specificity. Although it has been isolated from other avian species reared near domestic fowl, and there is a very similar virus in pheasants (Cavanagh et al., Citation2002), the chicken is thought to be the primary natural host. Furthermore, most IBV strains usually grow poorly in cell culture except in primary chicken kidney cells (Cunningham & Spring, Citation1965; Otsuki et al., Citation1979). However, isolation of IBV from species other than the chicken has shown that the host range of IBV has extended beyond the chicken, like other coronaviruses such as bovine coronavirus and severe acute respiratory syndrome-coronavirus (Cavanagh, Citation2005).
Studies on virus growth in cell culture are helpful for understanding the interaction between viruses and cells, and the mechanisms of virus interspecies infection. Only a few IBV strains, such as the Beaudette (IBV-42) and Holte, have been shown to replicate in mammalian cell lines such as Vero and BHK cells after adaptation (Fang et al., Citation2005). At least two reports have demonstrated that IBV strains could not be propagated in HeLa cells, a human cell line (Brown & Cunningham, Citation1971; Otsuki et al., Citation1979). In this report, we have investigated potential factors that might block IBV infection of HeLa cells.
Materials and Methods
IBV propagation in chicken embryo and HeLa cells
IBV strains M41, H52, H120, Gray, Holte, Connecticut and M52-19 (a Beaudette52-19 strain) (purchased from the China Institute of Veterinary Drug Control) were propagated in 10-day-old to 11-day-old embryonated specified pathogen free chicken eggs. The allantoic fluid was harvested at 48 to 72 h after inoculation, and clarified by centrifugation at low speed (1000×g) for 15 min at 4°C. The virus-containing supernatant was filter sterilized, and stored at −70°C.
HeLa cells from the China Centre for Type Culture Collection (GDC009) were used to propagate IBV strains. The cells were grown in an atmosphere of 37°C, 85% relative humidity and 5% CO2 in Dulbecco's Modified Eagle's Medium growth medium supplemented with 10% foetal calf serum (Hyclone), with appropriate antibiotics (penicillin 100 IU/ml and streptomycin 100 µg/ml) in a 100-ml cell culture flasks. Allantoic fluid (1 ml) containing 5×103.4 median egg infectious doses of IBV was inoculated into confluent HeLa monolayers or cells freshly digested HeLa with 0.25% trypsin (1:250) for each flask, and the infected cells were maintained in Dulbecco's Modified Eagle's Medium maintenance medium with 2% foetal calf serum. When over 80% cells showed cytopathic effect (CPE), including cells rounding, congregating and detaching from the substrate, the culture was harvested. After freezing and thawing three times, the virus-containing culture was clarified by centrifugation and stored for further passage. Passage involved adding 1/10 volume of virus to the cell culture medium in culture flasks.
A growth curve was produced by virus titration with conventional enzyme-linked immunosorbent assay (ELISA) (Peng et al., Citation2006) and median tissue culture infectious doses (TCID50) test according to the method of Reed & Muench (Citation1938). Briefly, 500 µl medium was taken every 6 h from the infected flasks. For ELISA, 20-fold diluted virus-containing culture medium was coated onto 96-cell microtitre plates. Specific binding was detected by rabbit antiserum (1:600) to IBV (prepared in our laboratory), and horseradish-peroxidase-conjugated goat antibody to rabbit IgG (1:5000). The signal was developed by adding 3,3′,5,5′-tetramethylbenzidine (TMB) substrate, and optical density values at the wavelength of 630 nm were recorded.
The culture of the infected cells was precipitated by 10.00% (w/v) PEG 8000 with 2.33% (w/v) NaCl overnight at 4°C, and centrifuged at 10 000×g for 30 min (SCR20BC; Hitachi). The pellet was suspended and further centrifuged in a continuous sucrose gradient, 30–60% (w/v) at 35 000×g for 3 h (OptimaÔ LE-80K Ultracentrifuge; Beckman Coulter). The white virus band was collected and dialysed for 24 h in phosphate-buffered saline (PBS). The final virus suspension was aliquoted and stored at −70°C.
Hyperimmune serum preparation
Two female New Zealand White rabbits were primed with purified M41, described above, involving 0.16 mg virus protein and an equal volume of Freund's complete adjuvant inoculated subcutaneously, and boosted with the same amount of purified virus and incomplete Freund's adjuvant at 2-week intervals. One week after the fourth injection, rabbits were bled and the sera were collected. The antiserum titre was determined by ELISA, and the specificity was determined by testing the antibody reaction with IBV, and other viruses such as avian influenza virus subtype H9N2, Newcastle disease virus and pseudorabies virus.
Sodium dodecyl sulphate-polyacrylamide gel electrophoresis and Western blot analysis
Cell culture or purified virus were separated by 10% sodium dodecyl sulphate-polyacrylamide gel electrophoresis and transferred to nitrocellulose membranes using a semi-dry Western blotting method (Kyhse-Anderson, Citation1984). Non-specific binding sites were blocked by incubation with 5% non-fat dry milk in PBS–0.1% Tween overnight at 4°C. Three washes were followed between every two steps. The membranes were incubated with rabbit anti-IBV antibodies for 1 h at 37°C. A horseradish-peroxidase-conjugated goat anti-rabbit immunoglobulin (Pierce) was added and kept at 37°C for 30 min. After three washes with PBS–0.1% Tween and two washes with PBS, a 3,3′- diaminobenzidine (DAB) substrate was used to visualize the bound antibodies.
Neuraminidase treatment of IBV and HeLa cells
The procedure was as previously described, with some modification (Schultze et al., Citation1992). The clarified IBV-containing culture after the fourth passage in HeLa cells was 10-fold serially diluted beginning at 1:5, treated with Vibrio cholerae neuraminidase (Sigma) at a final concentration of 30 mU/ml for 30 min at 3°C and put onto freshly digested HeLa cells in a 96-well cell culture plate at eight wells per dilution. Cells mock-infected or infected with untreated IBV were used as controls.
For cell treatments, V. cholerae neuraminidase at 10 mU was added to 6×106 freshly digested HeLa cells in a 1.5-ml microfuge tube, and incubated at 37°C for 1 h. The enzyme was removed by centrifugation, and the cells were washed three times with PBS. The treated cells were seeded equally into 10 wells of 24-well plates, and the untreated cells were used as controls. The virus diluted as previously described was inoculated into the wells. CPE occurrence was observed and the TCID50 was assessed by the Reed–Muench method.
Effect of trypsin on IBV replication in HeLa cells
The fourth passage of IBV in HeLa cells was 10-fold serially diluted and inoculated onto HeLa cell sheets at the ratio of 1:10 (v/v) and the cells were maintained in Dulbecco's Modified Eagle's Medium containing trypsin but deprived of foetal calf serum. To compare the effect of cell dispersers trypsin and ethylenediaminetetracetic acid (EDTA), the cells were respectively digested with 0.02% EDTA and trypsin, and the virus was titrated for the TCID50 as described previously.
IBV S1 gene sequencing
To detect IBV replication, the partial spike (S1) gene (1025 nucleotides) of IBV was amplified by reverse transcription-polymerase chain reaction (RT-PCR) after different passages according to the manufacturer's recommendation (Takara). The specific primers for IBV S1 gene were designed according to IBV H52 sequence (accession number AF3523151) as follows: forward, 5′-CTATGTAGTGCTGTTTTG-3′ (nucleotides 42 to 59); reverse, 5′-CCTTGAAGAGGACCGTAA-3′ (nucleotides 1049 to 1066). Total RNA was extracted by Trizol (Sangon) and the RT-PCR was run 30 min at 50°C and 2 min at 94°C for one cycle, then 30 cycles of 30 sec at 94°C, 30 sec at 55°C, 1.5 min at 72°C, followed by 15 min at 72°C (PTC-100™ Programmable Thermal Controller; MJ Research, Inc.). The RT-PCR products were cloned into pMD18-T vector (Takara) and custom-sequenced with automated cycle sequencing (Sangon).
Haemagglutination and the haemagglutination-inhibition test
IBV-containing materials were treated with lecithinase C (Sigma) at a final concentration 0.1 U/ml for 120 min at 37°C and the haemagglutination titre was tested with 0.5% chicken erythrocytes. Four haemagglutination units of IBV were added to two-fold serially diluted antisera starting from 1:100 and the serum haemagglutination (haemagglutination-inhibition) titre was assayed (King, Citation1988).
Neutralization test
Two-fold serially diluted antiserum was incubated with an equal volume of 200 TCID50 IBV for 1 h at 37°C. Then the mixture and freshly dispersed HeLa cells were added to the wells of 96-well plates using eight wells per dilution. The neutralization titre was determined according to the Reed–Muench Method (Reed & Muench, Citation1938).
Human aminopeptidase N blocking assay
Newly digested HeLa cells were pretreated for 1 h at 37°C with two-fold serial dilutions of monoclonal antibody WM15 to human aminopeptidase N (Biolegend), ranging from 1:20 to 1:2560. Then the cell–antibody mixture was challenged by IBV (100 TCID50). After 1 h co-incubation, cells in fresh maintenance medium containing the previous dilutions of antibody WM15 were seeded into 96-well plates with six wells per antibody dilution. The inhibition titres were determined as described in neutralization test. Cells infected with IBV pretreated by normal mouse serum and mock-infected cells were used as controls (Yeager et al., Citation1992).
Statistical analyses
Student's t-test and analysis of variance were used to test the significant difference between groups. P>0.05 was taken as no significant difference between the compared groups.
Results
IBV propagation in HeLa cells
Seven IBV strains were tested for growth in HeLa cells. Among them, the strains M41, H52, H120 and Gray could propagated in HeLa cells. CPE, shown by cell rounding, congregating and detaching from the substrate, was produced in infected cells from the very first passage in both HeLa cell monolayers and freshly trypsinized cells (). However, IBV propagated more efficiently in freshly digested HeLa cells than in cell monolayers, demonstrated by 1-day earlier occurrence of CPE and a 1000-fold higher TCID50. The virus-containing allantoic fluid (5×103.4 median egg infectious doses) was titrated in parallel with that from cell monolayers and freshly dispersed cells. The TCID50 reached 104.1±0.17/0.1 ml in freshly digested HeLa cells but only 101.0±0.21/0.1 ml in cell monolayers. Furthermore, the infected cell monolayers usually developed CPE at 72 h, while the infected freshly dispersed cells experienced CPE at 48 h post inoculation.
Figure 1. Cytopathic effect in IBV-infected HeLa cells. Confluent HeLa monolayers in 100-ml cell culture flasks were inoculated with 1 ml allantoic fluid containing 5×103.4 median egg infectious doses of IBV 41. After 72, CPE was observed. 1a: Mock-infected HeLa cells showing normally growing cells and intact monolayer. 1b: IBV-infected HeLa cells showing CPE: cell rounding, congregating and detaching from the substrate.
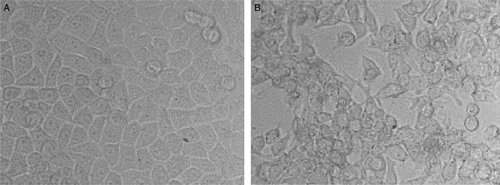
Strains M41, H52, H120 and Gray grew similarly in freshly dispersed cells, and the average TCID50 for M41, H52, H120 and Gray was 103.8±0.4/0.1 ml in the first five passages. For the strains Holte, Connecticut, and M52-19 strains, the infected cells were blindly passaged for three times and CPE was not observed.
The virus growth curve was produced with ELISA for the fifth passage. As shown in , virus replication began to be detected at 18 h post infection and the titre was kept at a low level until 42 h. Then viral replication experienced a rapid increase until 72 h post inoculation, when titration was terminated because the cells underwent severe CPE and detached ().
Figure 2. Growth curve of IBV M41 in HeLa cells. The cell culture supernatant was titrated with ELISA every 6 h post infection until 72 h, when the cells exhibited extensive CPE and detached from the substrate. OD, optical density.
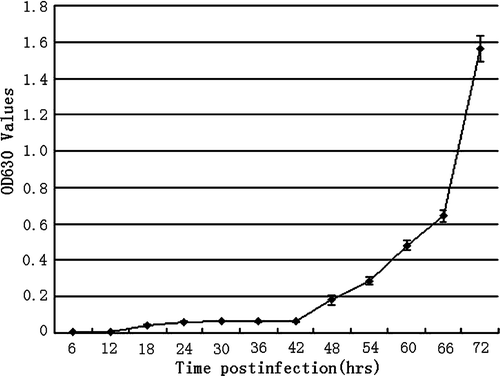
Because the four strains M41, H52, H120 and Gray replicated similarly in freshly digested HeLa cells in the first five passages, M41 was chosen to grow for 19 more passages to follow the viral adaptation. The culture supernatant was collected and the TCID50 was tested for every passage. The TCID50 at the level of 103.4±0.2/0.1 ml did not apparently change for the first 12 passages. The TCID50 then had its first increase from 103.6±0.1/0.1 ml in passage 12 to 104.2±0.2/0.1 ml in passage 13 (P<0.01). From passage 13 to 16, the virus titre remained at similar levels (104.2±0.2/0.1 ml). Then a rapid and high rise in the TCID50 occurred from 104.1±0.3/0.1 ml in passage 16 to 106.7±0.1/0.1 ml in passage 17. The titre remained level over the next seven passages, which was 106.6±0.3/0.1 ml on average for passages 17 to 24 when the experiment was terminated. In the meanwhile, M41 was grown in HeLa monolayers and the virus titre averaged 100.9±0.1/0.1 ml for five passages. The experiments were repeated three times ().
Figure 3. Virus titre (TCID50) change of IBV in HeLa cells with the increasing passage. 3a: IBV M41 was inoculated into freshly dispersed HeLa cells and incubated for 24 passages. The culture supernatant was collected and the TCID50 was tested for every passage. The TCID50 at the level of 103.4±0.2/0.1 ml did not apparently change for the first 12 passages, jumped to 104.2±0.2/0.1 ml for passages 13 to 16, and to 106.6±0.3/0.1 ml for passages 17 to 24 when the experiment was terminated. The experiments were repeated three times. 3b: IBV M41 was inoculated into HeLa cell monolayers and the virus titre averaged 100.9±0.1/0.1 ml over five passages.
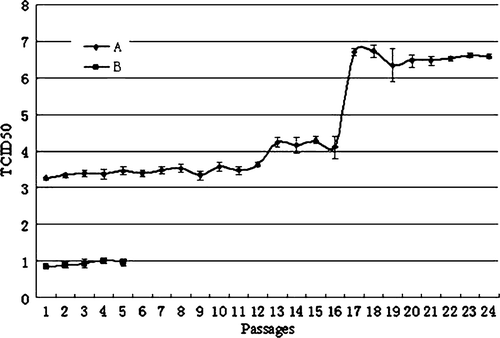
IBV S1 gene evolution
The S1 gene of IBV M41 from embryo allantoic fluid (P0) and HeLa cell culture for passages 5 (P5) and 21 (P21) was amplified with RT-PCR (). Two reactions for each passage were sequenced. Agarose gel electrophoresis showed a DNA fragment with the expected size of 1025 base pairs. Wilbur-Lipman DNA alignment with the Meglign program in DNAstar software pack indicated that there is 99.8% similarity with the IBV M41 S1 reference sequence (AY561712). Furthermore, S1 sequences for AY561712, P0, and P5 are the same. However, one nucleotide mutation (A728G) (numbering from the start codon ATG of S1) was observed in S1 from P21 leading to one amino acid replacement (Y242C). Passages 8 and 21 were chosen randomly to perform the Western blot and the results confirmed IBV replication. The most abundant viral N protein was detected for both passages ().
Figure 4. IBV identification by RT-PCR and Western blot assay. 4a: The S1 fragment of the IBV S protein amplified by RT-PCR. The S1 fragment (1025 nucleotides) was amplified from infected allantoic fluid (lane 2) and the fifth passage of IBV in HeLa cells (H5) (lane 3), but not from normal HeLa cells (lane 4) and distilled water (lane 5). Lane 1 represents the DNA ladder (DL2000). 4b: Western blot analysis of IBV propagated in HeLa cells. The viral proteins were separated by sodium dodecyl sulphate-polyacrylamide gel electrophoresis (10%), transferred to nitrocellulose membrane and analysed by western blotting with rabbit antiserum to IBV. Lane 1, purified virus from HeLa cell culture of passage 21; lane 2, passage 8; lane 3, uninfected cell culture. The arrow shows the most abundant viral protein, the nucleoprotein protein (N), with a molecular mass of 45 kDa.
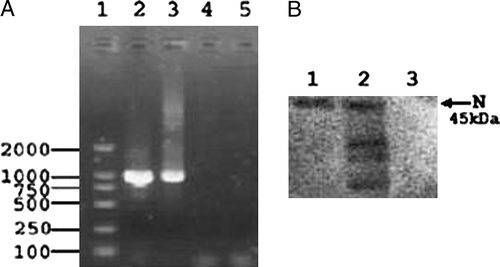
Trypsin effect on IBV replication in HeLa cells
The cytotoxic effect of trypsin with different concentrations was compared using 5 µg/ml, 10 µg/ml, 15 µg/ml, 20 µg/ml, 40 µg/ml, and 60 µg/ml. All the concentrations of trypsin above 5 µg/ml produced a toxic effect on HeLa cells, shown by the poor appearance of the cell monolayers with many detached cells and much cellular debris floating in the media. Therefore 5 µg/ml trypsin was used for the subsequent experiment. Ten-fold serially diluted IBV was used as the inoculum and CPE in the infected cells was hardly observed no matter whether trypsin was present or absent, suggesting that the trypsin was not able to enhance the virus replication in HeLa cell monolayers. However, virus replication was sufficiently improved by cellular digestion manipulation. This finding was further confirmed by the fact that both EDTA-dispersed and trypsin-dispersed cells supported virus replication similarly. IBV M41 after the fourth passage in HeLa cells was separately titrated in EDTA-digested and trypsin-digested cells. The virus produced a titre (TCID50) of 103.9±0.3/0.1 ml in trypsin-dispersed cells, and of 103.8±0.1/0.1 ml in EDTA-dispersed cells.
However, neuraminidase (NA) treatment of both cells and virus was able to affect the virus replication. NA-treated virus could remove sialic acid containing components on the virus surface and improved virus attachment into the host cells. As expected, the infectivity of the treated virus to freshly dispersed cells was improved. The TCID50 was increased by 16-fold from 103.4±0.1/0.1 ml for untreated control to 104.6±0.1/0.1 ml for NA-treated virus. On the other hand, NA treatment would destroy the sialic acid-containing receptor and decrease the virus entry efficiency. In fact, when NA treated the freshly dispersed cells, the TCID50 was reduced by 13-fold from 103.4±0.1/0.1 ml for untreated cells to 102.3±0.3/0.1 ml for NA treated cells.
Haemagglutination activity of IBV
The IBV HeLa cell culture after the first passage was tested for haemagglutination (HA) activity. The HA titres of M41, H52, H120 and Gray were 1:8, 1:4, 1:8 and 1:8, respectively, while Holte, Connecticut and M52-19 infected cells did not show any HA titre. The HA activity was gradually reduced with increased passages. The HA titres of M41 for the first (H1) and second (H2) passages were 1:8 and 1:4, respectively. No HA activity was detected from the third (H3) through fifth passages (H4 and H5). Fifty-fold concentrated virus from the fifth passage (H5) showed a HA titre of 1:8.
All the first five passages (H1 to H5) for M41 were inoculated back into chicken egg embryos with each passage for 10 embryos. The allantoic fluid for each passage was pooled to test HA activity. The titres of all passages (H1 to H5) were 27 (1:128). Furthermore, all the HA activity could be inhibited by the antiserum to IBV M41. haemagglutination-inhibition titre of the antiserum for H1 to H5 was 1:1600.
Virus inhibition assay
Antiserum against IBV M41 could neutralize IBV infectivity in HeLa cells, and the neutralization titre reached 1:336. However, all the dilutions (1:20 to 1:2560) of monoclonal antibody WM15 against human aminopeptidase N could not inhibit CPE produced by IBV in HeLa cells.
Discussion
Compared with some other coronaviruses, IBV is less readily grown in cell culture. The most susceptible cells are chicken kidney cells or chicken embryo kidney cells, in which many strains can be propagated and produce distinct CPE (Cunningham & Spring, Citation1965; Otsuki et al., Citation1979). Some chicken embryo kidney cell-adapted strains such as IBV-41, Iowa-97 and IBV-42 were able to grow and develop snycytial type CPE in the primate cell line VERO after 21 blind passages (Cunningham et al., Citation1972; Coria & Ritchie, Citation1973). One mechanism of this adaptation might be at the cost of abundant mutation accumulation. For example, it was demonstrated that 49 amino acid mutations occurred during the adaptation of Beaudette strain from chicken embryo to Vero cells. Furthermore, the majority of the mutations were accumulated and fixed before passage 7 (Fang et al., Citation2005). Among 49 amino acid mutations, 26 (53%) substitutions were located in the spike (S) glycoprotein. As is known, S is post-translationally cleaved into two subunits, S1 and S2. S1 is the determinant of IBV cell tropism (Casais et al., Citation2003; Cavanagh and Davis, Citation1986) and contains three highly variable regions within amino acids 38 to 67, 91 to 141, and 274 to 387 (Lee & Jackwood, Citation2001). During the above research, 73% (19/26) of S gene mutations occurred in the S1 subunit and 89% (17/19) of the S1 gene mutations were located within the three highly variable regions.
However, the current study demonstrated that the S1 gene did not experience a rapid mutation and selection during the host adaptation. Partial S1 gene from nucleotides 42 to 1066, which covered majority of highly variable regions from nucleotides 114 to 1161, was amplified from the infected HeLa cell culture at passage 0, 5, and 21 and sequenced respectively. The results showed that S1 was surprisingly stable in HeLa cells. Only one nucleotide mutation A728G resulting in amino acid replacement Y242C was discovered for the virus from passage 21. This mutation was confirmed by sequencing two reactions of PCR product and the recombinant plasmid. Because viral infection is an interaction between the virus and host cells, IBV adaptation in HeLa would be possibly dependent on some host determinants such as cell status, receptor glycosylation and alternative receptor utilization besides the viral evolution.
In fact, this study revealed that cell status is an important factor to impact viral replication efficiency. IBV propagated at a low level in cell monolayers, but significantly more efficiently in freshly dispersed cells based on the evidence from CPE observation, virus titration, growth curve description, S1 gene amplification and sequencing, neutralization assays with antiserum, HA and haemagglutination-inhibition tests. In the HeLa monolayer, IBV M41 produced a very low titre with TCID50 of 100.9±0.1/0.1 ml for five passages. However, the virus yielded a TCID50 of 103.4±0.2/0.1 ml in freshly dispersed HeLa cells. This titre was maintained for the first 12 passages.
Because we used trypsin to disperse cell monolayers, and trypsin was reported to be able to markedly enhance infectivity of various viruses in vitro such as rotavirus (Birch et al., Citation1983), influenza viruses (Appleyard & Maber, Citation1974), Sendai virus (Ohuchi & Homma, Citation1976), reovirus (Spendlove et al., Citation1970) and enable IBV strains to produce plaques in chicken embryo fibroblasts cells (Otsuki & Tsubokura, Citation1981), it was possible that trypsin had promoted IBV replication in freshly digested HeLa cells. However, no matter whether trypsin was present or absent during the virus growth, IBV developed the similar titres. Meanwhile, both trypsin-dispersed and EDTA-dispersed HeLa cells could similarly support IBV replication. As it is known, the freshly digested cells undergo active growth. Therefore, the above evidence suggests that the restriction of IBV replication in HeLa is the result of intracellular events rather than the binding to host cells or S-induced membrane fusion. A similar phenomenon was found for porcine parvovirus (Ridpath & Mengeling, Citation1988).
Virus growth in HeLa cells was followed for 24 continuous passages. In passage 13, the virus titre experienced an approximately 10-fold increase (104.2±0.2/0.1 ml). This new titre level was maintained from passages 13 to 16. Then a bigger increase occurred, which was 106.6±0.3/0.1 ml in passage 17. This level was maintained until passage 24 when the experiment was terminated.
We further explored the host factors that could have contributed to this IBV adaptation. IBV is one of the viruses with HA activity, like transmissible gastroenteritis virus, another coronavirus, and influenza virus. Sialic acid-containing receptors on the cell surface can render cells sensitive to IBV, which preferentially utilizes the α2,3-linked sialic acid (Winter et al., Citation2006). The NA treatment of host cells such as chicken erythrocytes (Schultze et al., Citation1992, Citation1993), primary chick embryo kidney cells, and Vero cells (Winter et al., Citation2006), rendered these cells resistant, to some extent, to IBV infection in a NA dose-dependent way (Winter et al., Citation2006). Our results agreed with the above findings. When NA at 10 mU per 6×106 cells was used to treat freshly dispersed cells, the virus TCID50 was reduced by 13-fold from 103.40±0.10/0.1 ml for untreated HeLa to 102.26±0.25/0.1 ml for NA-treated HeLa.
On the other hand, NA could remove sialic acid from the virus surface, which would block the attachment of IBV to host cells and improve the virus attachment to cells (Schultze et al., Citation1992, Citation1993). This study confirmed that NA treatment of IBV at a dose of 30 mU/ml resulted in a 16-fold (101.2) increase of TCID50/0.1 ml. Although IBV infection depended on the sialic acid-containing component, the sialic acid is probably not a unique receptor of IBV based on the broad distribution of sialic acid in various organs and cells, and the fact that IBV HA activity gradually disappeared with the viral adaptation in HeLa cells. It was demonstrated that there was an apparent difference in HA activity of IBV grown in HeLa cells and in chicken embryo. The viral HA activity to chicken erythrocytes was significantly decreased with increased passages in HeLa cells. A similar phenomenon was observed for other IBV strains propagated in cultured cells such as chicken kidney cells (King, Citation1984), chicken embryo kidney cells and VERO cells (Cunningham et al., Citation1972). However, this process is reversible. When the HeLa-adapted IBV was inoculated back to the chicken embryo, IBV HA activity was restored. Meanwhile, the virus titre was gradually increased during the process of continuous passage in HeLa. This finding suggests that the viral adaptation in HeLa was probably accompanied by alternative receptor utilization. Actually, two lines of evidences lend support to this hypothesis. Transmissible gastroenteritis virus can interact with two cellular receptors, aminopeptidase N and sialic acid. Transmissible gastroenteritis virus binding to sialic acid is required in enteropathogenesis, while binding to porcine aminopeptidase N is required for the infection of cultured cells (Krempl et al., Citation2000). Although the natural IBV receptor is not identified, it is reported that feline aminopeptidase N can be used as a common cellular receptor by group 1 coronaviruses of human, dogs and pigs and group 3 member IBV (Tresnan et al., Citation1996; Miguel et al., Citation2002). Our study demonstrated that monoclonal antibody WM15 to human aminopeptidase N could not prevent IBV infection in HeLa. The receptor of IBV, other than sialic acid, therefore remains to be investigated.
Collectively, this study demonstrated that several IBV strains (M41, H52, H120 and Gray) could be propagate in HeLa cells with distinct CPE. More efficient replication in freshly dispersed HeLa is probably the result of intracellular events rather than the binding to host cells. The virus adaptation would probably be determined by host cell modification such as receptor glycosylation and different receptor utilization instead of viral gene mutation. Besides, this study might represent an alternative adaptation pattern for coronaviruses where the virus persists at a low level in interspecies cells and breaks the replication restriction when the proper cell status exists and experiences further adaptation.
Acknowledgements
This work was supported by the China National Basic Research Program (China ‘973’ Program) (2003CB514122).
References
- Appleyard , G. and Maber , H.B. 1974 . Plaque formation by influenza viruses in the presence of trypsin . Journal of General Virology , 25 : 351 – 357 .
- Birch , C.J. , Rodger , S.M. , Marshall , J.A. and Gust , I.D. 1983 . Replication of human rotavirus in cell culture . Journal of Medical Virology , 11 : 241 – 250 .
- Brown , J.L. and Cunningham , C.H. 1971 . Immunofluorescence of avian infectious bronchitis virus and Newcastle disease virus in singly and dually infected cell cultures . Avian Diseases , 15 : 923 – 935 .
- Casais , R. , Dove , B. , Cavanagh , D. and Britton , P. 2003 . Recombinant avian infectious bronchitis virus expressing a heterologous spike gene demonstrates that the spike protein is a determinant of cell tropism . Journal of Virology , 77 : 9084 – 9089 .
- Cavanagh , D. 2001 . A nomenclature for avian coronavirus isolates and the question of species status . Avian Pathology , 30 : 109 – 115 .
- Cavanagh , D. 2003 . Severe acute respiratory syndrome vaccine development: experience of vaccination against avian infectious bronchitis coronavirus . Avian Pathology , 32 : 567 – 582 .
- Cavanagh , D. 2005 . Coronaviruses in poultry and other birds . Avian Pathology , 34 : 439 – 448 .
- Cavanagh , D. and Davis , P.J. 1986 . Coronavirus IBV: removal of spike glycopolypeptide S1 by urea abolishes infectivity and haemagglutination but not attachment to cells . Journal of General Virology , 67 : 1443 – 1448 .
- Cavanagh , D. , Mawditt , K. , Welchman , Dde B. , Britton , P. and Gough , R.E. 2002 . Coronaviruses from pheasants (Phasianus colchicus) are genetically closely related to coronaviruses of domestic fowl (infectious bronchitis virus) and turkeys . Avian Pathology , 31 : 81 – 93 .
- Coria , M.F. and Ritchie , A.E. 1973 . Serial passage of 3 strains of avian infectious bronchitis virus in African green monkey kidney cells (VERO) . Avian Diseases , 17 : 697 – 704 .
- Cunningham , C.H. and Spring , M.P. 1965 . Some studies of infectious bronchitis virus in cell culture . Avian Diseases , 14 : 182 – 192 .
- Cunningham , C.H. , Spring , M.P. and Nazerian , K. 1972 . Replication of avian infectious bronchitis virus in African green monkey kidney cell line VERO . Journal of General Virology , 16 : 423 – 427 .
- Fang , S.G. , Shen , S. , Tay , F.P. and Liu , D.X. 2005 . Selection of and recombination between minor variants lead to the adaptation of an avian coronavirus to primate cells . Biochemical and Biophysical Research Communications , 336 : 417 – 423 .
- Kan , B. , Wang , M. , Jing , H. , Xu , H. , Jiang , X. , Yan , M. , Liang , W. , Zheng , H. , Wan , K. , Liu , Q. , Cui , B. , Xu , Y. , Zhang , E. , Wang , H. , Ye , J. , Li , G. , Li , M. , Cui , Z. , Qi , X. , Chen , K. , Du , L. , Gao , K. , Zhao , Y.T. , Zou , X.Z. , Feng , Y.J. , Gao , Y.F. , Hai , R. , Yu , D. , Guan , Y. and Xu , J. 2005 . Molecular evolution analysis and geographic investigation of severe acute respiratory syndrome coronavirus-like virus in palm civets at an animal market and on farms . Journal of Virology , 79 : 11892 – 11900 .
- King , D.J. 1984 . Observations on the preparation and stability of infectious bronchitis virus hemagglutination antigen from virus propagated in chicken embryos and chicken kidney cell cultures . Avian Diseases , 28 : 504 – 513 .
- King , D.J. 1988 . A comparison of infectious bronchitis virus hemagglutination-inhibition test procedures . Avian Diseases , 32 : 335 – 341 .
- Krempl , C , Ballesteros , ML , Zimmer , G , Enjuanes , L , Klenk , HD and Herrler , G. 2000 . Characterization of the sialic acid binding activity of transmissible gastroenteritis coronavirus by analysis of haemagglutination-deficient mutants . Journal of General Virology , 81 : 489 – 496 .
- Kyhse-Anderson , J. 1984 . Electroblotting of multiple gels: a simple apparatus without buffer tank for rapid transfer of proteins from polyacrylamide to nitrocellulose . Jounal of Biochemical and Biophysical Methods , 10 : 203 – 209 .
- Lee , C.W. and Jackwood , M.W. 2001 . Origin and evolution of Georgia 98 (GA98), a new serotype of avian infectious bronchitis virus . Virus Research , 80 ( 1–2 ) : 33 – 39 .
- Miguel , B. , Pharr , G.T. and Wang , C. 2002 . The role of feline aminopeptidase N as a receptor for infectious bronchitis virus . Archives of Virology , 147 : 2047 – 2056 .
- Ohuchi , M. and Homma , M. 1976 . Trypsin action on the growth of Sendai virus in tissue culture cells. IV. Evidence for activation of sendai virus by cleavage of a glycoprotein . Journal of Virology , 18 : 1147 – 1150 .
- Otsuki , K. and Tsubokura , M. 1981 . Plaque formation by avian infectious bronchitis virus in primary chick embryo fibroblast cells in the presence of trypsin . Archives of Virology , 70 : 315 – 320 .
- Otsuki , K. , Noro , K. , Yamamoto , H. and Tsubokura , M. 1979 . Studies on avian infectious bronchitis virus (IBV). II. Propagation of IBV in several cultured cells . Archives of Virology , 60 : 115 – 122 .
- Peng , B. , Chen , H. , Tan , Y. , Jin , M. , Chen , H. and Guo , A. 2006 . Identification of one peptide which inhibited infectivity of avian infectious bronchitis in vitro . Science in China. Series C, Life Sciences , 49 : 158 – 163 .
- Reed , L.J. and Muench , H. 1938 . A simple method of estimating fifty percent endpoints . American Journal of Hygiene , 27 : 493 – 497 .
- Ridpath , J.F. and Mengeling , W.L. 1988 . Uptake of porcine parvovirus into host and nonhost cells suggests host specificity is determined by intracellular factors . Virus Research , 10 : 17 – 27 .
- Schultze , B. , Cavanagh , D. and Herrler , G. 1992 . Neuraminidase treatment of avian infectious bronchitis coronavirus reveals a hemagglutinating activity that is dependent on sialic acid-containing receptors on erythrocytes . Virology , 189 : 792 – 794 .
- Schultze , B. , Enjuanes , L. , Cavanagh , D. and Herrler , G. 1993 . N-Acetyneuraminic acid plays a critical role for the haemagglutinating activity of avian infectious bronchitis virus and porcine transmissible gastroenteritis virus . Advance of Experimental Medical Biology , 342 : 305 – 310 .
- Spendlove , R.S. , McClain , M.E. and Lennette , E.H. 1970 . Enhancement of reovirus infectivity by extracellular removal or alteration of the virus capsid by proteolytic enzymes . Journal of General Virology , 8 : 83 – 94 .
- Tresnan , D.B. , Levis , R. and Holmes , K.V. 1996 . Feline aminopeptidase N serves as a receptor for feline, canine, porcine, and human coronaviruses in serogroup I . Journal of Virology , 70 : 8669 – 8674 .
- Winter , C. , Schwegmann-Wessels , C. , Cavanagh , D. , Neumann , U. and Herrler , G. 2006 . Sialic acid is a receptor determinant for infection of cells by avian infectious bronchitis virus . Journal of General Virology , 87 : 1209 – 1216 .
- Yeager , C.L. , Ashmun , R.A. , Williams , R.K. , Cardellichio , C.B. , Shapiro , L.H. , Look , A.T. and Holmes , K.V. 1992 . Human aminopeptidase N is a receptor for human coronavirus 229E . Nature , 357 : 420 – 421 .