Abstract
The prevalence of infection with avian influenza (AI) virus varies significantly between taxonomic Orders and even between species within the same Order. The current understanding of AI infection and virus shedding parameters in wild birds is limited and largely based on trials conducted in mallards (Anas platyrhynchos). The objective of the present study was to provide experimental data to examine species-related differences in susceptibility and viral shedding associated with wild bird-origin low-pathogenicity avian influenza (LPAI) viruses in multiple duck species and gulls. Thus mallards, redheads (Aythya americana), wood ducks (Aix sponsa), and laughing gulls (Leucophaeus atricilla) were inoculated experimentally with three wild mallard-origin LPAI viruses representing multiple subtypes. Variation in susceptibility and patterns of viral shedding associated with LPAI virus infection was evident between the duck and gull species. Consistent with the literature, mallards excreted virus predominantly via the gastrointestinal tract. In wood ducks, redheads, and laughing gulls, AI virus was detected more often in oropharyngeal swabs than cloacal swabs. The results of this study suggest that LPAI shedding varies between taxonomically related avian species. Such differences may be important for understanding the potential role of individual species in the transmission and maintenance of LPAI viruses and may have implications for improving sampling strategies for LPAI detection. Additional comparative studies, which include LPAI viruses originating from non-mallard species, are necessary to further characterize these infections in wild avian species other than mallards and provide a mechanism to explain these differences in viral excretion.
Introduction
Although avian influenza (AI) viruses have been reported from over 100 wild avian species (Stallknecht & Shane, Citation1988; Olsen et al., Citation2006; Stallknecht & Brown, Citation2008), the vast majority of virus isolations have been associated with species utilizing wetlands and aquatic environments (Webster et al., Citation1992; Stallknecht & Brown, Citation2007). To date, most AI virus isolations have been reported from anseriform and haradriiform species (Stallknecht, Citation2003; Stallknecht & Brown, Citation2008), which together are considered to form the primordial reservoir for AI virus. Several decades of surveillance indicate that the prevalence of AI virus infection varies significantly between different genera and species within these two taxonomic orders (Stallknecht, Citation2003). Within Anseriformes, the majority of AI virus isolations are from dabbling ducks in the Genus Anas (Olsen et al., Citation2006) and higher isolation rates have been reported from mallards (Anas platyrhynchos) than any other avian species (Hinshaw et al., Citation1980; Stallknecht & Shane, Citation1988; Stallknecht et al., Citation1990; Krauss et al., Citation2004; Munster et al., Citation2005; Olsen et al., Citation2006; Stallknecht & Brown, Citation2007). AI virus is frequently isolated from other genera of ducks, geese and swans, but at a lower prevalence and less consistently (Stallknecht et al., Citation1990; Olsen et al., Citation2006; Stallknecht et al., Citation2007). The epidemiology and host range of AI in the Order Charadriiformes is much less understood than for the Anseriformes. Within Charadriiformes, most AI viruses have been isolated from species in two families, Scolopacidae and Laridae (Stallknecht & Brown, Citation2007). Since the first isolation of AI virus from a wild bird in 1961 during an outbreak of highly pathogenic AI virus of H5N3 subtype in common terns (Sterna hirundo) (Becker, Citation1966), AI viruses have been routinely isolated from gulls and less frequently from terns (Olsen et al., Citation2006; Stallknecht & Brown, Citation2008). AI viruses of H13 and H16 subtypes are almost exclusively maintained in gull populations, and rarely detected in other wild avian taxa (Fouchier et al., Citation2005, Citation2007; Velarde et al., Citation2010). This variation in prevalence may be explained by species-related differences in habitat utilization and behaviour, which collectively affect the likelihood of exposure to AI virus (Olsen et al., Citation2006; Garamszegi & Moller, Citation2007; Munster et al., Citation2007). Prevalence may also be affected by differences in species susceptibility or variations in shedding patterns that affect transmission efficiency; both of which, as in the case of H13 and H16 viruses (Fouchier et al., Citation2007), may be influenced by AI virus adaptation to specific taxonomic groups or species.
Although multiple experimental trials have been conducted with low-pathogenicity avian influenza (LPAI) viruses in wild birds, most of them used mallards or Pekin ducks (Anas platyrhynchos domesticus), a mallard-type domestic duck, as experimental models (Bahl & Pomeroy, Citation1977; Kida et al., Citation1980; Cooley et al., Citation1989; Mundt et al., Citation2009; Jourdain et al., Citation2010). Species-related variation is known to occur (Bahl & Pomeroy, Citation1977; Morales et al., Citation2009; Mundt et al., Citation2009) and, therefore, results obtained from mallards cannot be extrapolated to all Anseriformes and Charadriiformes species. In this manner, the current knowledge of susceptibility and viral shedding of AI viruses in their natural host is still very limited. A deeper understanding of AI virus transmission and maintenance in the wild bird reservoir system is needed to help interpret field surveillance results, guide future AI virus sampling efforts, and define risks of virus spilling over into domestic birds.
The objective of the present study was to evaluate susceptibility and patterns of viral shedding in multiple duck and gull species inoculated experimentally with mallard-origin LPAI viruses. Four indigenous North American species were included in this study: mallards, redheads (Aythya americana), wood ducks (Aix sponsa), and laughing gulls (Leucophaeus atricilla). These species were selected to represent the variation in reported AI virus prevalence (Olsen et al., Citation2006) commonly observed in wild aquatic bird species. Although behaviour and habitat utilization vary greatly between these species, it is currently unknown whether the observed prevalence differences are associated with individual or combined effects of differences in contact and species associations, susceptibility, or reduced transmission or detection related to different patterns of viral shedding.
Materials and Methods
Birds
Three Anseriformes and one Charadriiformes species were included in the present study. Ten-week-old to 16-week-old mallards, redheads, and wood ducks were purchased from a commercial breeder (Chenoa Waterfowl, Martin, Tennessee, USA). Nestling laughing gulls were hand-caught in McIntosh County, Georgia at approximately 7 to 10 days of age, by personnel of Georgia Department of Natural Resources. The gulls were raised under confined conditions at the College of Veterinary Medicine, The University of Georgia (UGA), until approximately 12 weeks of age. Gulls used in this investigation were acquired through the Southeastern Cooperative Wildlife Disease Study (SCWDS), UGA, under federal permit. For each species, 20 birds were included in the experimental trial, and both males and females were represented in approximately equal numbers.
Viruses
Three wild bird-origin LPAI viruses representing different haemagglutinin subtypes were used in this study: A/mallard/MN/355779/00 (H5N2), A/mallard/MN/182761/98 (H7N3), and A/mallard/MN/199106/99 (H3N8). All viruses were originally isolated in specific pathogen free embryonated chicken eggs (ECE) from cloacal swabs collected from mallards. Viral stocks were propagated by second passage in 9-day-old to 11-day-old ECE, and the infectious titre of the stock was calculated by determining the modal egg infectious dose (EID50) (Reed & Muench, Citation1938; Woolcock, Citation2008). To prepare the viral inocula, infective allantoic fluid was diluted in sterile brain–heart-infusion (BHI) medium to yield a final titre of 106EID50 per 0.1 ml (single-bird inoculum). The back titres were determined by titration in ECE after the ducks and gulls were inoculated and varied from 105.27 to 106.27 EID50/0.1 ml. A 0.1 ml volume of sterile BHI medium was used as a sham inoculum.
Experimental design
For each of the four species, 20 birds were divided randomly into three treatment groups and one negative control group, with five birds in each group. The groups were housed separately in biocontainment isolation units ventilated under negative pressure with high efficiency particulate air filters on intake and exhaust. Food and water were provided ad libitum. After a 4-day acclimatization period, treatment or negative control groups were inoculated via choanal cleft with one of the three LPAI viruses or sterile BHI medium, respectively. Birds were observed two times a day for behavioural change or clinical signs. Cloacal and oropharyngeal (OP) swabs were collected from all birds at 0, 1, 2, 3, 4, 5, 8, 12, 16, and 21 days post inoculation (d.p.i.). Blood samples for serological testing were collected from the right jugular vein at 0, 12, and 21 d.p.i. The trial was terminated at 21 d.p.i., when birds were euthanized by carbon dioxide inhalation, and a full necropsy was performed. Experimental trials were performed in a BSL-Ag2+ facility at the Poultry Diagnostic and Research Center, UGA. General animal care was provided and experimental sampling was performed according to an animal care and use protocol approved by the Institutional Animal Care and Use Committee at UGA.
Virus isolation
Cloacal and OP swabs were collected in vials containing 2 ml of sterile BHI medium with antimicrobial drugs (100 µg/ml gentamicin, 100 u/ml penicillin, and 5 µg/ml amphotericin B) and were stored at –70°C until further testing. Virus isolations were performed in 9-day-old to 11-day-old specific pathogen free ECE and, after incubation, amnioallantoic fluid (AAF) was tested for the presence of haemagglutination activity using standard protocols (Killian, Citation2008; Woolcock, Citation2008).
RNA extraction and real-time RT-PCR
RNA was extracted from cloacal and OP swab material and from all of the AAF samples that tested positive for the presence of haemagglutination activity after virus isolation. The MagMAX™-96 AI/ND Viral RNA Isolation Kit (Ambiron, Austin, Texas, USA) and the Thermo Electron KingFisher magnetic particle processor (Thermo Electron Corporation, Waltham, Massachusetts, USA) were used for the RNA extraction. RNA was tested for AI virus by quantitative real-time reverse transcription-PCR (rRT-PCR) targeted to the influenza matrix gene as described (Spackman & Suarez, Citation2008). Samples with a cycle threshold (Ct) value equal to or less than 40.00 on rRT-PCR were considered positive.
Serology
Serum samples were stored at –20°C until tested for the presence of influenza A antibodies using the agar gel immunodiffusion (AGID) test (Swayne et al., Citation2008), and a commercial blocking enzyme-linked immunosorbent assay (bELISA) AI virus antibody test kit (FlockChek™ AI MultiS-Screen antibody test kit; IDEXX Laboratories, Westbrook, Maine, USA). The AGID test was performed using reported procedures (Swayne et al., Citation2008). The commercial bELISA was performed following the manufacturer's instructions.
Histopathological analyses
Samples of the cerebrum, cerebellum, heart, lung, trachea, liver, spleen, oesophagus, proventriculus, ventriculus gizzard, small intestine, large intestine, pancreas, adrenal gland, ovaries/testis, kidney, bursa, pectoral muscle, nasal turbinates and sinus were collected from all the animals during necropsy, and fixed in 10% neutral buffered formalin for histopathological examination. After fixation, the tissues were processed and embedded in paraffin, and 5 µm sections were stained with haematoxylin and eosin using standard histopathology protocols. Turbinates were decalcified with Kristensen's decalcifying solution (Kristensen, Citation1948) before being processed for histopathologic examination as described for the other tissues.
Statistical analyses
The kappa coefficient with 95% confidence interval was used to compare agreement between virus isolation and rRT-PCR results. Interpretation of κ was performed based on Landis & Koch (Citation1977).
Results
None of the birds had pre-existing antibodies to AI virus or were excreting virus at the start of the experiment, as demonstrated by negative serological and virus isolation results for samples collected at 0 d.p.i. The birds in the negative control group remained seronegative throughout the trial and virus was not detected in swab samples collected at any time point from this group. Morbidity or mortality was not observed in any of the three species of ducks in any of the treatment groups. However, three laughing gulls, one in each treatment group, died during the trial. Based on post-mortem examination, the mortality in these three gulls was related to complications due to aspergillosis, pododermatitis, and septic arthritis. As a result of these deaths, each of the three gull treatment groups had a total of four birds. The results and analysis presented below reflect this change in sample size.
Viral shedding
The pattern of viral shedding, including route and duration, varied between duck and gull species and individual virus isolates. Based on virological results ( and ) and/or serological results (), all three LPAI virus strains replicated in each of the four species included in this study. However, not all birds were infected by the H3N8 (wood ducks and redheads) or the H7N3 (all species). The agreement rate between the results obtained with rRT-PCR and those observed on virus isolation was 91% (κ=0.81), indicating a high association between these two tests. The high virus isolation success rate may reflect the fact that these birds were challenged with egg-adapted viruses.
Figure 1. Viral shedding pattern of four different wild bird species, as determined by either virus isolation or real time RT-PCR of oropharyngeal (OP) and cloacal (Clo) swabs. Birds were inoculated via choanal cleft with an infectious dose of 106EID50 (0.1mL) of either one of the LPAI viruses: A/mallard/MN/355779/00(H5N2); A/mallard/MN/199106/99(H3N8); A/mallard/MN/182761/98(H7N3). dpi, days post inoculation.
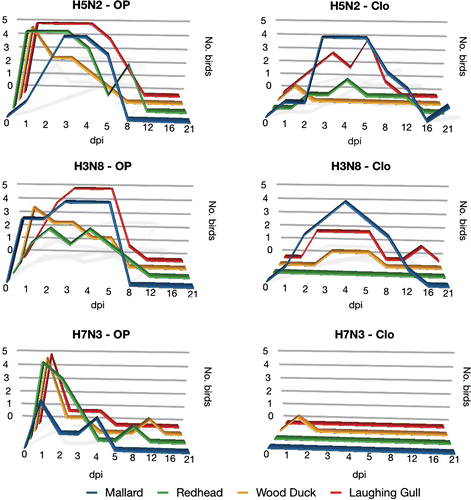
Table 1. Virus isolation and rRT-PCR results of oropharyngeal and cloacal swabs from four species of wild birds experimentally inoculateda with three different low pathogenic avian influenza (LPAI) viruses.
Table 2. Seroconversion at 12 or 21 d.p.i. from four species of wild birds experimentally inoculateda with three different LPAI viruses.
With infected mallards, virus isolation and rRT-PCR primarily detected AI virus in cloacal swabs. The H7N3, however, was unique in this species in that the virus detection was restricted to OP swabs. With all other species (wood duck, redhead, and laughing gull), positive VI or rRT-PCR results were predominantly associated with OP swabs. This was especially pronounced in wood ducks in which cloacal shedding was limited to only one bird in each treatment group.
The duration of viral shedding, as detected by virus isolation and rRT-PCR also varied between species and, with the exception of the H7N3 virus, was detected the longest in mallards; positive cloacal swabs could be detected for 21 and 12 days for H5N2 and H3N8, respectively. Shedding was detected up to 12 days for wood ducks (H7N3, OP swab) and redheads (H5N2, cloacal swab), and up to 16 days in laughing gulls (H3N8, OP swab).
Serology
The frequency of seroconversion varied between virus isolates. All birds in each of the four species seroconverted after exposure to the H5N2 virus. Seroconversion rates for the H3N8 and H7N3 treatment groups were 74% and 63%, respectively (). The agreement between the results of the AGID test and the bELISA was 88% (κ=0.77), indicating a substantial association between these two tests.
Necropsy and histopathological analysis
No gross or histopathological lesions associated with AI virus infection were observed in any of the treatment or negative control groups.
Discussion
In the present study, the frequency of infection and patterns of viral shedding varied between LPAI viruses and duck and gull species. In general, mallards and laughing gulls had higher frequencies of infection than wood ducks and redheads, suggesting they may be more permissive hosts for mallard-origin LPAI viruses. This was most apparent for the A/mallard/MN/355779/00 (H5N2) and A/mallard/MN/199106/99 (H3N8) LPAI virus treatment groups. The A/mallard/MN/182761/98 (H7N3) virus was the least infective of the viruses for all four species. Based on these results, the H7N3 LPAI virus appears to be a less fit virus for replication in wild avian reservoir hosts; a surprising observation considering the H7N3 LPAI virus was originally isolated from a mallard. The reason for the poor infectivity of the H7N3 LPAI virus is not known; however, several potential explanations include adaptation of the virus to eggs during the propagation of stock, poor adaptation to the new host species, and/or differences in host susceptibility.
Seroconversion was related directly with the ability of the viruses to replicate. The higher rate of seroconversion was observed in the H5N2 groups, which also had a higher rate of viral shedding. Although a substantial association was observed between the results of the AGID test and the bELISA, lack of sensitivity of the AGID test for waterfowl has been reported (Slemons & Easterday, Citation1972; Cattoli & Capua, Citation2007; Swayne & Halvorson, Citation2008).
In this study, mallards had the most extensive viral shedding based on numbers of bird shedding the virus and the duration of shedding. This observation is consistent with historic surveillance results of higher isolation rates from mallards, and supports the important role that this species plays in the natural history of AI. The mallard is distributed worldwide and, as previously mentioned, more AI viruses have been isolated from this species than any other wild avian species (Hinshaw et al., Citation1980; Stallknecht et al., Citation1990; Krauss et al., Citation2004; Munster et al., Citation2005; Olsen et al., Citation2006; Stallknecht & Brown, Citation2007; Hesterberg et al., Citation2009). Mallards in the H5N2 and H3N8 treatment groups had an extended period of viral shedding, primarily via the cloaca. This is consistent with reported experimental and field results from this species (Ellström et al., Citation2008; Costa et al., Citation2010). Interestingly, as opposed to mallards, viral shedding in wood ducks, redheads, and laughing gulls was predominately via the oropharynx, suggesting the importance of respiratory tract replication of LPAI viruses in these species. Another study with experimental AI virus infection reported viral shedding up to 23 days via the oropharynx in Franklin's gulls (Leucophaeus pipixcan) (Bahl & Pomeroy, Citation1977). The results of the present study suggest that the traditional paradigm that replication of LPAI viruses in waterfowl occurs primarily in cells lining the lower intestinal tract (Slemons & Easterday, Citation1977; Webster et al., Citation1978; Kida et al., Citation1980) may not be applicable to all avian species, even within the Anseriformes, but corroborate the opinion that the replication sites of AI viruses vary according to the host species and virus strain (Kida et al., Citation1980).
Based on these observations, cloacal swabs would represent the ideal sampling method for surveillance studies of mallards, as reported (Ellström et al., Citation2008) and may have relevance to other closely related species in the genus Anas. However, for avian species other than dabbling ducks, a combination of OP and cloacal swabs might be more appropriate and increase the likelihood of detection in waterfowl.
Interestingly, the difference in tissue tropism (respiratory versus intestinal tract) of LPAI viruses observed in the present study may be in some way related to the previously reported variation on susceptibility to Eurasian H5N1 HPAI virus among these species. For instance, high susceptibility to Eurasian H5N1 HPAI virus has been reported in wood ducks and laughing gulls, and moderate susceptibility has been observed in redheads (Brown et al., Citation2006, Citation2007; Keawcharoen et al., Citation2008). In the present experimental trial, wood ducks, laughing gull and redheads had predominantly oropharyngeal shedding of AI viruses. Mallards, however, have been shown to be less susceptible to an Eurasian H5N1 HPAI infection (Brown et al., Citation2006) and, in the present study, primarily cloacal viral shedding was observed in mallards. Although AI virus receptor distribution, among other factors, may contribute to this host species variation, additional studies are required to elucidate possible mechanisms involved in this process.
The observations in the present study suggest that different avian species can have dissimilar roles in the transmission of LPAI viruses, and may explain the variation in prevalence observed in wild bird populations (Stallknecht & Shane, Citation1988; Olsen et al., Citation2006). The variation in shedding patterns (predominantly cloacal or OP) observed may have been related to viral origin (mallard) or the route of inoculation (via choanal cleft). However, this variation may also reflect true inter-species differences that enhance or limit viral transmission. For example, cloacal shedding may represent the ideal transmission mode in mallard populations where transmission primarily occurs through a faecal/oral route involving contaminated water (Stallknecht & Brown, Citation2007). In contrast, enhanced OP shedding in gulls may represent an efficient source for transmission within breeding gull populations (Velarde et al., Citation2010). PAI viruses are not commonly reported from wood ducks and redheads (Olsen et al., Citation2006), and this may be relate to an inefficient means of viral transmission via OP shedding under conditions of small flock size (wood ducks) or utilization of larger and deeper aquatic habitats (redheads). Further comparative studies are necessary to explain these variations and to elucidate the reservoir potential of different Anseriformes and Charadriiformes species for LPAI viruses, as well as to provide a better understanding of the evolution of LPAI viruses in their natural hosts.
Acknowledgements
The authors thank the personnel of the Southeastern (SCWDS) Cooperative Wildlife Disease Study for their technical assistance, especially Ginger Goekjian, Rebecca Poulson, and Jennifer Smith, and the Georgia Department of Natural Resources personnel for collecting the gulls. The authors deeply appreciate Dr. Renata Casagrande for her help on bird handling and sample collection. Funding for the present work was provided through a cooperative agreement between the Southeast Poultry Research Laboratory and the SCWDS, and through the continued sponsorship of SCWDS member state and federal agencies.
References
- Bahl , A.K. and Pomeroy , B.S. 1977 . Experimental exposure of Franklins' gulls (Larus pipixcan) and mallards (Anas platyrhynchos) to a turkey influenza A virus A/Turkey/Minn/BF/71 (Hav6Neq2) . Journal of Wildlife Diseases , 13 : 420 – 426 .
- Becker , W.B. 1966 . The isolation and classification of tern virus: influenza virus A/Tern/South Africa/1961 . The Journal of Hygiene , 64 : 309 – 320 .
- Brown , J.D. , Stallknecht , D.E. , Beck , J.R. , Suarez , D.L. and Swayne , D.E. 2006 . Susceptibility of North American ducks and gulls to H5N1 highly pathogenic avian influenza viruses . Emerging Infectious Diseases , 12 : 1663 – 1670 .
- Brown , J.D. , Stallknecht , D.E. , Valeika , S. and Swayne , D.E. 2007 . Susceptibility of wood ducks to H5N1 highly pathogenic avian influenza virus . Journal of Wildlife Diseases , 43 : 660 – 667 .
- Cattoli , G. and Capua , I. 2007 . Diagnosing avian influenza in the framework of wildlife surveillance efforts and environmental samples . Journal of Wildlife Diseases , 43 : S35 – S39 .
- Cooley , A.J. , Van Campen , H. , Philpott , M.S. , Easterday , B.C. and Hinshaw , V.S. 1989 . Pathological lesions in the lungs of ducks infected with influenza A viruses . Veterinary Pathology , 26 : 1 – 5 .
- Costa , T.P. , Brown , J.D. , Howerth , E.W. and Stallknecht , D.E. 2010 . The effect of age at infection on avian influenza viral shedding in mallards (Anas platyrhynchos) . Avian Diseases , 54 : 581 – 585 .
- Ellström , P. , Latorre-Margalef , N. , Griekspoor , P. , Waldenstrom , J. , Olofsson , J. Wahlgren , J. 2008 . Sampling for low-pathogenic avian influenza A virus in wild Mallard ducks: oropharyngeal versus cloacal swabbing . Vaccine , 26 : 4414 – 4416 .
- Fouchier , R.A. , Munster , V. , Wallensten , A. , Bestebroer , T.M. , Herfst , S. Smith , D. 2005 . Characterization of a novel influenza A virus hemagglutinin subtype (H16) obtained from black-headed gulls . Journal of Virology , 79 : 2814 – 2822 .
- Fouchier , R.A.M. , Munster , V.J. , Keawcharoen , J. , Osterhaus , A.D.M.E. and Kuiken , T. 2007 . Virology of avian influenza in relation to wild birds . Journal of Wildlife Diseases , 43 : S7 – S14 .
- Garamszegi , L.Z. and Moller , A.P. 2007 . Prevalence of avian influenza and host ecology . Proceedings of the Royal Society B: Biological Sciences , 274 : 2003 – 2012 .
- Hesterberg , U. , Harris , K. , Stroud , D. , Guberti , U. , Basani , L. Pittman , M. 2009 . Avian influenza surveillance in wild birds in the European Union in 2006 . Influenza and Other Respiratory Viruses , 3 : 1 – 14 .
- Hinshaw , V.S. , Webster , R.G. and Turner , B. 1980 . The perpetuation of orthomyxoviruses and paramyxoviruses in Canadian waterfowl . Canadian Journal of Microbiology , 26 : 622 – 629 .
- Jourdain , E. , Gunnarsson , G. , Wahlgren , J. , Latorre-Margalef , N. , Bröjer , C. Sahlin , S. 2010 . Influenza virus in a natural host, the mallard: experimental infection data . PLoS ONE, , e , 5 : 8935
- Keawcharoen , J. , van Riel , D. , van Amerongen , G. , Bestebroer , T. , Beyer , W.E. van Lavieren , R. 2008 . Wild ducks as long-distance vectors of highly pathogenic avian influenza virus (H5N1) . Emerging Infectious Diseases , 14 : 600 – 607 .
- Kida , H. , Yanagawa , R. and Matsuoka , Y. 1980 . Duck influenza lacking evidence of disease signs and immune response . Infection and Immunity , 30 : 547 – 553 .
- Killian , M.L. 2008 . “ Hemagglutination assay for the avian influenza virus ” . In Avian Influenza Virus , 1st edn , Edited by: Spackman , E. 47 – 52 . Totowa, NJ : Humana Press .
- Krauss , S. , Walker , D. , Pryor , S.P. , Niles , L. , Chenghong , L. Hinshaw , V.S. 2004 . Influenza A viruses of migrating wild aquatic birds in North America . Vector-Borne and Zoonotic Diseases , 4 : 177 – 189 .
- Kristensen , H.K. 1948 . An improved method of decalcification . Stain Technology , 23 : 151 – 154 .
- Landis , J.R. and Koch , G.G. 1977 . The measurement of observer agreement for categorical data . Biometrics , 33 : 159 – 174 .
- Morales , A.C. Jr , Hilt , D.A. , Williams , S.M. , Pantin-Jackwood , M.J. , Suarez , D.L. Spackman , E. 2009 . Biologic characterization of H4, H6, and H9 type low pathogenicity avian influenza viruses from wild birds in chickens and turkeys . Avian Diseases , 53 : 552 – 562 .
- Mundt , E. , Gay , L. , Jones , L. , Saavedra , G. , Tompkins , S.M. and Tripp , R.A. 2009 . Replication and pathogenesis associated with H5N1, H5N2, and H5N3 low-pathogenic avian influenza virus infection in chickens and ducks . Archives of Virology , 154 : 1241 – 1248 .
- Munster , V.J. , Wallensten , A. , Baas , C. , Rimmelzwann , G.F. , Schutten , M. Olsen , B. 2005 . Mallards and highly pathogenic avian influenza ancestral viruses, northern Europe . Emerging Infectious Diseases , 11 : 1545 – 1551 .
- Munster , V.J. , Baas , C. , Lexmond , P. , Waldenstrom , J. , Wallensten , A. Fransson , T. 2007 . Spatial, temporal, and species variation in prevalence of influenza A viruses in wild migratory birds . PLoS Pathogens, , e , 3 : 61
- Olsen , B. , Munster , V.J. , Wallensten , A. , Waldenstrom , J. , Osterhaus , A.D.M.E. and Fouchier , R.A. 2006 . Global patterns of influenza a virus in wild birds . Science , 312 : 384 – 388 .
- Reed , L.J. and Muench , H. 1938 . A simple method of estimating fifty percent endpoint . American Journal of Epidemiology , 27 : 493 – 497 .
- Slemons , R.D. and Easterday , B.C. 1972 . Host response differences among 5 avian species to an influenzavirus-A/turkey/Ontario/7732/66 (Hav5N?) . Bulletin of the World Health Organization , 47 : 521 – 525 .
- Slemons , R.D. and Easterday , B.C. 1977 . Type-A influenza viruses in the feces of migratory waterfowl . Journal of the American Veterinary Medical Association , 171 : 947 – 948 .
- Spackman , E. and Suarez , D.L. 2008 . “ Type A influenza virus detection and quantitation by real-time RT-PCR ” . In Avian Influenza Virus , 1st edn , Edited by: Spackman , E. 19 – 26 . Totowa, NJ : Humana Press .
- Stallknecht , D.E. 2003 . Wildlife population surveillance . Avian Diseases , 47 : 98 – 104 .
- Stallknecht , D.E. and Brown , J.D. 2007 . Wild birds and the epidemiology of avian influenza . Journal of Wildlife Diseases , 43 : S15 – S20 .
- Stallknecht , D.E. and Brown , J.D. 2008 . “ Ecology of avian influenza in wild birds ” . In Avian Influenza , 1st edn , Edited by: Swayne , D.E. 43 – 58 . Ames, IA : Blackwell Publishing .
- Stallknecht , D.E. and Shane , S.M. 1988 . Host range of avian influenza virus in free-living birds . Veterinary Research Communications , 12 : 125 – 141 .
- Stallknecht , D.E. , Shane , S.M. , Zwank , P.J. , Senne , D.A. and Kearney , M.T. 1990 . Avian influenza viruses from migratory and resident ducks of coastal Louisiana . Avian Diseases , 34 : 398 – 405 .
- Stallknecht , D.E. , Nagy , E. , Hunter , D.B. and Slemons , R.D. 2007 . “ Avian Influenza ” . In Infectious Diseases of Wild Birds , 1st edn , Edited by: Thomas , N.J. , Hunter , D.B. and Atkinson , C.T. 108 – 130 . Ames, IA : Blackwell Publishing .
- Swayne , D.E. and Halvorson , D.A. 2008 . “ Influenza ” . In Diseases of Poultry , 12th edn , Edited by: Saif , Y.M. , Fadly , A.M. , Glisson , J.R. , McDougald , L.R. , Nolan , L. and Swayne , D.E. 153 – 184 . Ames, IA : Blackwell Publishing .
- Swayne , D.E. , Senne , D.A. and Suarez , D.L. 2008 . “ Avian influenza ” . In Isolation, Identification, and Characterization of Avian Pathogens , 5th edn , Edited by: Dufour-Zavala , L. 128 – 134 . Jacksonville, FL : American Association of Avian Pathologists .
- Velarde , R. , Calvin , S.E. , Ojkic , D. , Barker , I.K. and Nagy , É. 2010 . Avian influenza virus H13 circulating in ring-billed gulls (Larus delawarensis) in Southern Ontario, Canada . Avian Diseases , 54 : 411 – 419 .
- Webster , R.G. , Yakhno , M. , Hinshaw , V.S. , Bean , W.J. and Murti , K.G. 1978 . Intestinal influenza: replication and characterization of influenza viruses in ducks . Virology , 84 : 268 – 278 .
- Webster , R.G. , Bean , W.J. , Gorman , O.T. , Chambers , T.M. and Kawaoka , Y. 1992 . Evolution and ecology of influenza A viruses . Microbiological Reviews , 56 : 152 – 179 .
- Woolcock , P.R. 2008 . “ Avian influenza virus isolation and propagation in chicken eggs ” . In Avian Influenza Virus , 1st edn , Edited by: Spackman , E. 35 – 46 . Totowa, NJ : Humana Press .