Abstract
Replication of a recombinant herpesvirus of turkey vaccine expressing infectious laryngotracheitis virus genes (rHVT-LT) was evaluated in specific pathogen free (SPF) and commercial broiler chickens after various vaccination protocols (amniotic route at embryonation day [ED] 18; intra-embryonic route at ED 19; and subcutaneous at 1 day of age [s.c.]). Three experiments were conducted: in the first experiment, replication of rHVT-LT vaccine was chronologically evaluated and compared with the replication of herpesvirus of turkey (HVT) in SPF chickens; in the second experiment, the effect of different in ovo vaccination procedures on rHVT-LT vaccine replication was evaluated in SPF chickens; and in the third experiment, the effect of different in ovo vaccination procedures on rHVT-LT vaccine replication was evaluated in commercial broiler chickens with maternal antibodies against HVT and infectious laryngotracheitis virus (LTV). rHVT-LT vaccine replicated in chickens after in ovo (ED 18 and ED 19) or s.c. administration at a similar level. In vivo replication of rHVT-LT vaccine was slower than HVT vaccine. However, in vivo both rHVT-LT and HVT vaccines replicated at similar levels. Both vaccines were consistently detected in the spleen and feather pulp and at lower frequency in the lung. The frequency of samples with detectable levels of rHVT-LT DNA was lower in broiler chickens than in SPF chickens, probably due to interactions with maternal antibodies. Differences between SPF chickens and broiler chickens were found also in the transcription of the LTV glycoprotein I gene (gI). In SPF chickens, in ovo inoculation resulted in a higher number of spleen samples with detectable gI transcripts than s.c. inoculation. In broiler chickens, however, no differences in the level of gI transcripts in spleen samples were found between chickens vaccinated in ovo and those vaccinated by the s.c. route. Transcription of LTV gI gene in lung samples was very low in both SPF and broiler chickens. Further studies to evaluate the mucosal immune response elicited by rHVT-LT in chicken with and without maternal antibodies are warranted.
Introduction
Laryngotracheitis (LT) is an acute respiratory tract infection of chickens that may result in severe production losses due to mortality and reduced egg production (Guy & Bagust, Citation2003). Successful control of LT based on immunization of chickens was first accomplished by application of virulent virus to the cloaca (Brandly & Bushnell, Citation1934). Subsequently, it was demonstrated that immunity could be provided by immunization of chickens with attenuated modified live vaccines (ML-LT vaccines) administered via the infraorbital sinuses, intranasal instillation, feather follicles, eye drop, and orally through drinking water (Guy & Bagust, Citation2003) ML-LT vaccines have been prepared by sequential passage of infectious laryngotracheitis virus (LTV) in tissue cultures (Gelenczei & Marty, Citation1965) and embryonated chicken eggs (CEO) (Samberg & Aronovici, Citation1969).
Administration of CEO ML-LT vaccines via drinking water or by spray is rapid and allows mass vaccination but has important drawbacks. Administration of CEO ML-LT vaccines by the drinking water route can result in a high proportion of chickens that fail to develop protective immunity (Robertson & Egerton, Citation1981). Successful vaccination via the drinking water is dependent upon the vaccine virus making contact with susceptible nasal epithelial cells, via aspiration of vaccine virus through external nares or choanae; however, this occurs infrequently in chickens vaccinated by the drinking water (Robertson & Egerton, Citation1981). Incorrect administration of CEO ML-LT vaccines by spray may result in adverse reactions as a result of insufficient attenuation of vaccine virus, deep penetration of the respiratory tract due to the small droplet size of the spray (Purcell & Surman, Citation1974), or excessive dose (Clarke et al., Citation1980).
CEO ML-LT vaccines have been associated with a variety of adverse effects including insufficient attenuation, production of latently infected carriers (Bagust & Johnson, Citation1995), and increased virulence as a result of bird-to-bird passage (Brandly & Bushnell, Citation1934). ML-LT vaccine viruses have been shown to spread readily from vaccinated to non-vaccinated chickens (Guy et al., Citation1989; Devlin et al., Citation2008). The spread of CEO ML-LT vaccine viruses is a potential safety hazard, as spread to non-vaccinated birds results in bird-to-bird passage and possible reversion of vaccine virus to virulence (Brandly & Bushnell, Citation1934). Spread of vaccine viruses may be prevented by biosecurity measures that prevent flock-to-flock and farm-to-farm spread, and by using vaccination methods that ensure simultaneous infection with vaccine virus of all susceptible birds on a farm.
In recent years, the disease has occurred with increased frequency, primarily in broiler chickens, despite increased usage of CEO ML-LT vaccines. LT outbreaks in broiler populations are believed to occur most commonly as a consequence of CEO ML-LT vaccine virus spillover from breeder/layer operations due to close proximity and lax biosecurity (Dufour-Zavala, Citation2008). Vaccination of broiler flocks during LT outbreaks generally is accomplished using CEO ML-LT vaccines administered in drinking water, even though this practice may perpetuate these outbreaks (Guy et al., Citation1991). Vaccination of individual birds in broiler flocks is not practiced because it is cost prohibitive; mass vaccination of broiler flocks is accomplished via drinking water or by in ovo delivery of vaccine. Tissue culture ML-LT vaccine, although considered to be safe, is not effective when mass administered in the drinking water or in spray.
Recently, two recombinant, virus-vectored vaccines (r-LT vaccines) for immunization of chickens against LT have been produced and are licensed for use in the USA (rHVT-LT and rFPV-LT) (Tong et al., Citation2001; Davison et al., Citation2006; Johnson et al., Citation2010). The rHVT-LT vaccine currently is licensed for in ovo administration; the rFPV-LT vaccine currently is licensed only for wing-web inoculation of breeder/layer chickens. r-LT vaccines provide opportunities for development of alternative LT control procedures; they induce immunity without producing disease or latent carriers, and they potentially can be used for exclusion or eradication of LT from production sites (Bagust & Johnson, Citation1995). In addition, they can be administered in ovo and therefore could be useful for the broiler industry. The use of r-LT vaccines in broilers has increased within the past several years but their efficacy does not seem to be as high as that conferred by ML-LT vaccines (Guy et al., Citation2011). In ovo vaccination with either rHVT-LT or rFPV-LT did not confer as much protection as CEO vaccines administered at 2 weeks in broiler chickens challenged with LTV at 3 weeks (Guy et al., Citation2011). Reduced protection could be due to insufficient LTV antigen expression, poor mucosal-induced immune responses, and/or reduced replication of the vector vaccine. Genetic modification of Marek's disease virus (MDV) can result in decreased ability of the recombinant virus to replicate in vivo (Morgan et al., Citation1998; Schumacher et al., Citation2000; Cui et al., Citation2004; Lupiani et al., Citation2004; Gimeno et al., Citation2005).
The objectives of the present study were to evaluate in vivo replication of rHVT-LT vaccine and to optimize vaccination protocols. Three protocols were evaluated in this study, in ovo vaccination by the amniotic route at embryonation day (ED) 18, in ovo vaccination by the intra-embryonic route at ED 19, and subcutaneous (s.c.) inoculation at 1 day of age.
Materials and Methods
Chickens
For Experiments 1 and 2, Sunrise specific pathogen free (SPF) chickens (Sunrise farm, Catskill, New York, USA) were used. For Experiment 3, commercial broiler chickens with maternal antibodies against LTV and MDV were used (Tyson Foods, Eastover, North Carolina, USA). Chickens did not receive any vaccination other than the ones evaluated in the present study.
Viruses
rHVT-LT and herpesvirus of turkey (HVT) from two different commercial sources were used following manufacturer recommendations. rHVT-LT is a recombinant herpesvirus of turkeys that contains the ILT virus glycoprotein D (gD) and glycoprotein g (gI) genes inserted into a unique xhoI site converted to a PacI site in the BamHI #10 fragment of the HVT genome (US Patent No. 5,853,733). The LTV gD and gI genes are in the opposite transcriptional orientation to the open reading frame A within the BamHI #10 fragment of the HVT genome. The ILTV gD and gI genes are expressed as overlapping transcripts from their own respective endogenous LTV promoters, and share their own endogenous polyadenylation signal (US Patent No. 5,853,733).
Vaccine titration
Virus titration was performed in 35 mm plates with a confluent monolayer of secondary chicken embryo fibroblasts (CEF) cells. Five 10-fold dilutions of the two reconstituted vaccines were made in Liebovitz-McCoy media (Sigma-Aldrich, Inc., St Louis, Missouri, USA). Of each dilution, 500 μl was added to each plate with CEF cells with 1.5 ml Liebovitz-McCoy media (Sigma-Aldrich, Inc.). Two plates were used for each dilution. Infected cultures were incubated at 37°C and 5% CO2 and virus growth was evaluated daily. Media were removed and substituted by fresh media after 24 h initially and every 48 h afterwards. Plaques were microscopically counted at 3, 4, 5, 6, and 7 days. Virus titres were calculated as plaque-forming units (PFU) per millilitre and expressed as PFU per chicken dose.
Detection of maternal antibodies (enzyme-linked immunosorbent assay)
Maternal antibody titres against LTV were measured by the Synbiotics ProFLOK® LT ELISA kit (Synbiotics, San Diego, California, USA) following the manufacturer's recommendations. This kit detects antibodies elicited by wild-type virus infection and by ML-LT-vaccine but not by rHVT-LT vaccine.
Experimental design
Three experiments were conducted to evaluate replication of rHVT-LT in chickens. In all three experiments, chickens sampled at each time point were selected randomly based on the wing band numbers. Five spare chickens were included in each treatment group to account for possible early mortality. Unused chickens were euthanized at the end of the experiment. In Experiment 1, 100 Sunrise SPF embryos were inoculated at ED 18 via the amniotic route with the recommended dose or either rHVT-LT or HVT (50 embryos per vaccine), and 100 Sunrise SPF embryos were inoculated at ED 19 via the intra-embryonic route with the recommended dose of either rHVT-LT or HVT (50 embryos per vaccine). All in ovo injections were performed manually. Thirty chickens of each vaccinated group and 30 unvaccinated chickens were wing-banded at 1 day of age and placed in Horsfall–Bauer isolators for the remainder of the experiment. Five chickens from each treatment group were euthanized at 1, 2, 3, 4, and 5 weeks and samples of the spleen, lung, and feather pulp (FP) were collected for DNA extraction.
In Experiment 2, 30 Sunrise SPF embryos were inoculated at ED 18 via the amniotic route and another 50 embryos were inoculated at ED 19 via the intra-embryonic route with the recommended dose of rHVT-LT. All in ovo injections were performed manually. In addition, another 30 Sunrise SPF chicks were inoculated by the s.c. route at 1 day of age with rHVT-LT. Thirty chickens of each vaccinated group and 30 unvaccinated chickens were wing-banded at 1 day of age and placed in Horsfall–Bauer isolators for the remainder of the experiment. Five chickens from each treatment group were euthanized at 3 weeks and samples of the spleen, lung, and FP were collected for nucleic acid extraction.
Experiment 3 had a similar design to Experiment 2. The difference was that commercial broiler chickens were used instead of Sunrise SPF chickens and they were raised in isolated floor-pens (BSL-2). Chicks used in this experiment came from flocks of two different ages (30 weeks and 50 weeks). In each treatment group, there were 30 chickens from each origin. Maternal antibody levels were measured for each chicken by enzyme-linked immunosorbent assay (ELISA) at 2 weeks of age. In addition, maternal antibody levels were measured at hatch in 50 unvaccinated chicks that were not included in the study.
For every experiment, an additional subset of 100 eggs was injected with dye at the time of injection and deposition sites determined. Sites of dye deposition were defined as intra-embryo if dye was deposited in the embryo, as allantoic if the dye was found in the allantois, and as amnion if the dye was found in the amniotic fluid. The site of vaccine disposition demonstrated that the majority of vaccine delivered at ED 18 went into the amnion while the majority of the vaccine at ED 19 was delivered intra-embryo. Compartment targeting precision, however, was lower than that seen in automatic injections. In Experiments 1 and 2, the vaccine deposition site was amnion in 72% of the ED 18 vaccinated eggs and intra-embryo in 67% of the ED 19 vaccinated eggs. In Experiment 3, the vaccine deposition site was amnion in 74% of the ED 18 vaccinated eggs and intra-embryo in 85% of the ED 19 vaccinated eggs.
Real-time polymerase chain reaction
Samples of the lung and spleen were collected directly into cell lysis solution (PURAGENE® DNA isolation kit; Gentra Systems, Inc. Minneapolis, Minnesota, USA). Feathers with fleshy calamus were collected from the alar tracts and FP was extracted as reported earlier (Kuhnlein et al., Citation2006). Real-time polymerase chain reaction (PCR) assay was performed as described previously (Gimeno et al., Citation2008).
Real-time reverse transcriptase-PCR
RNA was extracted using the Perfect Pure RNA tissue kit (5-Prime Inc., Gaithersburg, Maryland, USA) following the manufacturer's recommendations. Real-time reverse transcriptase-PCR was performed to evaluate transcription of LTV gI, HVT glycoprotein B (gB), and HVT ICP4 genes. Each sample was amplified with two primer sets specific for LTV gI, HVT gB, HVT ICP4 genes and for the housekeeping gene 28S rRNA. Primer sets were designed using the OligoPerfect™ Designer software program (Invitrogen, Carlsbad, California, USA) (Genbank accession numbers U28832 and AF291866.1) or have been reported elsewhere (Abdul-Careem et al., Citation2008). Primer sets are shown in . Amplifications were carried out in 25 μl reactions using Brilliant SYBR Green Q-PCR Master Mix (Biocrest-Stratagene, Cedar Creek, Texas, USA) in a Mx3005 Stratagene (Stratagene, La Jolla, California, USA) machine. The cycle profile was as follows: 1 cycle of 50°C for 30 min; one cycle of 95°C for 10 min; 50 cycles of 95°C for 10 sec and 56°C for 1 min; and one cycle of 95°C for 1 min, 55°C for 30 sec, and 95°C for 30 sec. Each sample was run in triplicate and the Ct was averaged from triplicates. The average Ct value was subtracted from the total number of cycles (50 – average Ct). To correct for differences in RNA levels between samples within an experiment, a 28S correction factor for each sample was calculated using the formula:
(50 – overall mean Ct 28S rRNA-specific product from all samples)/(50 – average Ct for 28S rRNA-specific product for each sample)
28S correction factor X (50 – Ct value for viral gene) X viral gene slope/28S gene slope)
Table 1. Oligonucleotide primers used for real-time PCR.
Statistical analysis
Data were analysed with the statistical program Statistica (Stat Soft, Tulsa, Oklahoma, USA). Comparisons between two groups were conducted by Student's t test. Comparisons among more than two groups were conducted by a one-way analysis of variance (ANOVA) test. The Scheffé test was used as a post hoc analysis. The level of significance considered was P <0.05.
Four continuous variables were studied (viral DNA load, LTV gI transcripts, HVT ICP4 transcripts, and HVT gB transcripts). Three type of comparisons were done for each of the continue variable studied: comparison between various treatment groups at one time point and in one given tissue; comparisons within one treatment group at one time point between several tissues; and comparisons within one treatment group in one single tissue between time points.
Results
Vaccine titres
Vaccination was conducted following manufacturer recommendations and one full dose of either rHVT-LT or HVT vaccines was administered. Titration of the vaccine showed that 1 ml of rHVT-LT vaccine contained 1.6 x 106 PFU and 1 ml of HVT contained 8 x 106 PFU. Plaques could be detected in the plates inoculated with HVT as early as 3 days post inoculation (d.p.i.) and they were counted at 5 d.p.i. On the other hand, plaques were not detected until 5 d.p.i. in plates inoculated with rHVT-LT and they were counted at 7 d.p.i.
Evaluation of maternal antibody in broiler chickens from 30-week-old and 50-week-old dams
Maternal antibody levels against LTV were evaluated by ELISA in 100 1-day-old broiler chicks (50 from 30-week-old dams and 50 from 50-week-old dams) that were not included in the study. No significant differences were found between chicks from 30-week-old dams and chicks from 50-week-old dams (a). Maternal antibody levels against LTV were also measured in every chicken used in Experiment 3 at 2 weeks of age. Maternal antibody levels at 2 weeks were low for both groups and no significant differences were detected between chickens coming from 30-week-old dams and those coming from 50-week-old dams (b).
Figure 1. Level of maternal antibodies against LTV measured by ELISA. 1a: Level of maternal antibodies against LTV in 50 unvaccinated 1-day-old chicks derived from a 30-week-old parent flock and 50 unvaccinated 1-day-old chicks derived from a 50-week-old parent flock. 1b: Level of maternal antibodies against LTV in broiler chickens used in Experiment 3 at 2 weeks of age. There were 30 rHVT-LT-vaccinated 2-week-old chickens derived from the same 30-week-old parent flock and 30 rHVT-LT-vaccinated 2-week-old chickens derived from the same 50-week-old parent flock. Results are presented as the mean±standard error (SE)±standard deviation (SD) in a box and whisker plot graphic.
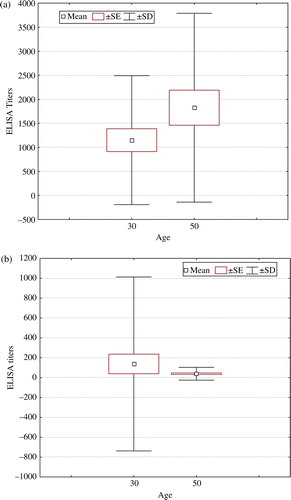
There were no statistically significant differences between broiler chickens coming from 30-week-old and 50-week-old dams regarding vaccine DNA and LTV gI, HVT gB, and HVT ICP4 transcripts (). To simplify the presentations of the results, data from the two groups of broilers were pooled as no differences were found between them in the level of maternal antibodies or in any of the features evaluated in the present study.
Table 2. Comparisons of vaccine DNA load and transcription o f LTV gI, HVT gB, and HVT ICP4 genes in various tissues at 3 weeks of age in broilers coming from 30-week-old and 50-week-old flocks.a
Chronological evaluation of rHVT-LT and HVT DNA load in the spleen, feather pulp, and lung of SPF chickens (Experiment 1)
Recombinant HVT-LT and HVT DNA load was evaluated at 1, 2, 3, 4, and 5 weeks of age in the spleen, FP, and lung () of SPF chickens after i n ovo administration at ED 18 or ED 19. HVT and rHVT-LT DNA were found in most samples (80 to 100%) regardless of the type of tissue, treatment group, and time point. Exceptions to that were FP samples at weeks 3 and 5 in the group vaccinated with rHVT-LT at ED 18 (60%); spleen samples at week 5 in the group vaccinated with rHVT-LT at ED 18 (40%); and lungs at week 5 in the groups vaccinated with rHVT-LT at ED 18 (40%), rHVT-LT at ED 19 (20%), or HVT at ED 18 (60%).
Table 3. Chronological evaluation of rHVT-LT DNA load in the spleen, FP, and lung of SPF chickens (Experiment 1).a
Differences in the viral DNA load among treatment groups were not statistically significant at weeks 1, 2, and 4 for any of the studied tissues. Statistically significant lower DNA load than other treatment groups were found at weeks 3 and 5 in the FP of chickens vaccinated with rHVT-LT at ED 18; at week 5 in the spleen of chickens vaccinated with rHVT-LT at ED 18; and at week 5 in the lung of chickens vaccinated with rHVT-LT at ED 19.
Effect of age and route of inoculation on rHVT-LT replication in SPF chickens (Experiment 2)
rHVT-LT DNA load and transcription of LTV gI, HVT gB, and HVT ICP4 genes () were evaluated in various tissues at 3 weeks of age after administration of rHVT-LT at ED 18 and ED 19 or s.c. at 1 day of age. As in Experiment 1, rHVT-LT DNA was found in most tested samples (80 to 100%). An exception to this was the lung of SPF chickens vaccinated at ED 19 (60%). No statistically significant differences in the load of vaccine DNA were found among treatment groups or among tissues ().
Table 4. Effect of age and route of vaccination on the DNA load of rHVT-LT and on the transcription of LTV gI gene in the spleen, FP, and lung of SPF chickens (Experiment 2) and broiler chickens (Experiment 3) at 3 weeks of age.a
Transcription of the LTV gI gene differed between tissues and among groups and was much lower than frequency of chickens with detectable rHVT-LT DNA. SPF chickens receiving vaccine in ovo had more gI transcripts in the spleen (60 to 80%) than those receiving the vaccine s.c. at 1 day of age (0%) (). Transcription of LTV gI in the lungs was low in all treatment groups (0 to 20%).
Transcription of HVT gB was low in the spleen and lung of all treatment groups. HVT gB transcripts were detected in 40% and 20% of the spleen samples in the groups vaccinated at ED 18 and ED 19, respectively. No HVT gB transcripts were detected in the spleens of chickens vaccinated s.c. or in any lung samples regardless of the treatment group ().
HVT ICP4 transcripts were detected in 40% and 80% of the chickens vaccinated at ED 18 and ED 19, respectively, but it was not detected in the spleens of chickens vaccinated s.c. Only 20% of the lungs in each of the treatment groups had HVT ICP4 transcripts ().
Effect of various in ovo vaccination protocols on rHVT-LT replication in broiler chickens (Experiment 3)
rHVT-LT DNA load and transcription of LTV gI, HVT gB, and HVT ICP4 genes () were evaluated in various tissues at 3 weeks of age after administration of rHVT-LT at ED 18 and ED 19 or s.c. at 1 day of age in broiler chickens. Contrasting with Experiments 1 and 2, rHVT-LT DNA was found in fewer tested samples. The low percentage of positive samples was mainly due to the low frequency of positive lungs (20 to 50%). The frequency of positive samples in other tissues, although lower than in the previous experiment, was significantly higher than in the lung (70 to 80% of spleen samples and 70 to 90% of FP samples). No statistically significant differences in the load of rHVT-LT DNA were found among treatment groups (). The lung had less rHVT-LT DNA load than other tissues in all groups but differences were only statistically significant within the group of chickens that received the vaccine at ED 18.
No difference in LTV gI transcripts were found in the spleen of broiler chickens among treatment groups (40 to 60%). As in SPF chickens, transcription of LTV gI in the lung of broilers chickens was low (20 to 30%).
Transcription of the HVT gB gene was detected in few samples (0 to 10% of spleens and 10 to 20% of lungs). No statistically significant differences were detected among treatment groups or between tissues.
Transcription of the HVT ICP4 gene was detected in more spleen samples than lung samples (40 to 60% vs. 20 to 30%). No statistically significant differences were found in the gI transcripts among groups or among tissues ().
Discussion
The present work demonstrates that rHVT-LT vaccine replicated in the chickens after in ovo (ED 18 and ED 19) vaccination or s.c. administration at 1 day of age at similar level. Our results also demonstrated that even though in vitro replication of rHVT-LT vaccine was slower than HVT vaccine, replication of rHVT-LT vaccine in vivo was similar to that of HVT vaccine in most tissues at most time points (even though they were administered at different doses). Vaccine viruses were consistently detected in the spleen and FP (regardless of the administration age/route) and at lower frequency in the lung. The frequency of samples with detectable levels of rHVT-LT DNA was lower in broiler chickens than in SPF chickens, probably due to interactions with maternal antibodies. Differences between SPF chickens and broiler chickens were found also in the transcription of LTV gI transcripts. In SPF chickens, in ovo inoculation resulted in a higher number of spleen samples with detectable gI transcripts than s.c. inoculation. In broiler chickens, however, no differences in the level of gI transcripts in spleen samples were found between chickens vaccinated in ovo and those vaccinated by the s.c. route. That was also true for the transcription of HVT gB and ICP4 genes. Expression of LTV gI, HVT gB, and HVT ICP4 transcripts in lung samples was very low in both SPF and broiler chickens.
In previous experiments, we have demonstrated that protection conferred by r-LT vaccines was lower than protection conferred by ML-LT vaccines (Guy et al., Citation2011). In particular, rHVT-LT conferred the lowest protection in that study (Guy et al., Citation2011). In the present work, we have demonstrated that the reduced protection is not due to lack of replication of rHVT-LT. rHVT-LT replicated after in ovo (ED 18 and ED 19) and s.c. administration at similar levels. Recombinant HVT-LT DNA load was also comparable with that achieved after HVT vaccination in most tissues and most time points even though rHVT-LT was administered at much lower dose than HVT. Differences between the load of HVT and rHVT-LT were found at 5 weeks of age in the spleen and FP of chickens inoculated at ED 18 and in the lung of those inoculated at ED 19. The relevance of reduced vaccine load at 5 weeks in some of the tissues on protection is unclear and deserves further evaluation. Lower protection against LVT challenge, however, could be related to reduced expression of LTV viral antigens in the respiratory tract leading to low mucosal immunity. In this study, LTV and HVT transcripts in the lung were lower than in the spleen at all evaluated time points. We did not evaluate the expression of LTV or HVT antigens by immunohistochemistry and therefore the exact location of rHVT-LT, whether in bronchial-associated lymphoid tissue or in lung parenchyma, was not determined. In addition, transcripts were only evaluated at one time point (3 weeks of age) and it cannot be ruled out whether higher expression of LTV antigens could have been found earlier than 3 weeks. Further studies evaluating viral antigen expression at different times are warranted to demonstrate viral protein expression and site of vaccine replication.
In previous work, we have demonstrated that efficacy of rHVT-LT vaccine is enhanced with simultaneous in ovo administration of a rFPV-LT (Guy et al., Citation2011). A synergistic protective enhancement between HVT and rFPV-MD has been reported previously for protection against Marek's disease (Nazerian et al., Citation1996; Lee et al., Citation2003). The mechanisms by which those viruses elicited enhanced protection when administered together are poorly understood. However, those results suggested that r-LT vaccines have the potential of improvement when administered in combination with other viruses. Expression of different LTV genes by the two vectors or a strong innate immune response elicited by a combination of both viruses might be involved on the enhanced protection. Further studies to better understand mucosal immune responses elicited by rHVT-LT by itself or in combination with other vaccines are warranted.
Based on the results of the present study, FP seems to be the most suitable sample to monitor rHVT-LT vaccine replication in the chickens by real-time PCR as it is very reliable and minimally invasive. In this work, blood samples were not evaluated. However, in previous work it has been demonstrated that blood is not an adequate sample to detect HVT (Cortes et al., Citation2011). FP samples can be collected in cell lysis solution (Cortes et al., Citation2011), frozen (Cortes et al., Citation2011) or on FTA® cards (Cortes et al., Citation2009). Plaque assay from splenocytes or buffy coats cells could also be used for virus detection. However, differences in the in vitro growth between HVT and rHVT-LT need to be considered if detection by plaque assay is conducted. rHVT-LT took longer to grow in CEF than HVT. The HVT plaques were detected microscopically at 3 d.p.i. and counted at 5 d.p.i. for CEF. However, none or very few plaques could be detected in plates cultured with rHVT-LT at 3 d.p.i. The number of rHVT-LT plaques increased at 5 d.p.i. and were counted at 7 d.p.i.
The differences in the titres of vaccines between rHVT-LT vaccine and HVT vaccine were remarkable. Regardless of the differences in administered PFU, rHVT-LT reached similar levels to HVT in chickens by 1 week of age. The low initial PFU numbers, however, may have a negative effect if the vaccine dose is further reduced during administration. Many factors can reduce the vaccine titres during the handling and administration of the vaccine (i.e. lack of vaccine refrigeration, mixing with antibiotics, and using the reconstituted vaccine over prolonged periods of time). Dilution of vaccines to reduce vaccination cost is also a common practice within the broiler chicken industry. Administration of doses lower than the minimum protective dose (1000 PFU as per USA requirements) will have a negative impact not only on the protection against LTV but also against Marek's disease (Landman & Verschuren, Citation2003; Baigent et al., Citation2007; Gimeno et al., Citation2011). In the present study we did not evaluate replication of vaccines during the first week after vaccination, and therefore in vivo differences between rHVT-LT and HVT at early stages cannot be ruled out. Our results also cannot rule out possible interactions between rHVT-LT and HVT in vivo, if administered together.
The frequency of samples with detectable levels of rHVT-LT DNA was lower in broiler chickens than in SPF chickens. This could be due to genetic differences (Bacon & Witter, Citation1993, Citation1994a, Citationb) or due to the presence of maternal antibodies against MDV and LTV. In the present study, we confirmed the presence of maternal antibody against LTV and quantified it by ELISA. However, we did not measure maternal antibodies against MDV. Broiler chickens used in this study came from dams vaccinated in ovo with HVT + SB-1 and it is highly likely that they had maternal antibodies against MDV. Maternal antibodies have been shown to play a major role in reducing Marek's disease vaccine efficacy (Lee & Witter, Citation1991; Lee et al., Citation2003). Vaccines strains deriving from very virulent serotype 1 MDV and a recombinant FPV expressing MDV gB were highly protective in chickens lacking maternal antibodies against MDV but protected poorly in chickens with maternal antibodies against MDV (Lee et al., Citation2003). Cell-associated HVT seems to be less affected by the presence of maternal antibodies against MDV than the cell-free HVT, but inhibition of cell-associated HVT has also been reported (Calnek & Smith, Citation1972; King et al., Citation1981). Protection studies in chickens with maternal antibodies against MDV and LTV will be necessary to determine whether reduced replication is associated with reduced efficacy of rHVT-LT vaccines in chickens with maternal antibodies.
The present study has demonstrated that rHVT-LT vaccine replicates in the chicken after in ovo and s.c. administration. Expression of LTV antigens in the lung was much lower than in the spleen and this could be a drawback in inducing mucosal immune responses against LTV in the respiratory tract. Further studies to evaluate mucosal immune responses elicited by ML-LT vaccines and r-LT vaccines in chickens with and without maternal antibodies are warranted.
Acknowledgements
The authors thank Pfizer Animal Health for funding the present study.
References
- Abdul-Careem , M.F. , Hunter , D.B. , Lambourne , M.D. , Read , L.R. , Parvizi , P. and Sharif , S. 2008 . Expression of cytokine genes following pre- and post-hatch immunization of chickens with herpesvirus of turkeys . Vaccine , 26 : 2369 – 2377 .
- Bacon , L.D. and Witter , R.L. 1993 . Influence of B-haplotype on the relative efficacy of Marek's disease vaccines of different serotypes . Avian Diseases , 37 : 53 – 59 .
- Bacon , L.D. and Witter , R.L. 1994a . B haplotype influence on the relative efficacy of Marek's disease vaccines in commercial chickens . Poultry Science , 73 : 481 – 487 .
- Bacon , L.D. and Witter , R.L. 1994b . Serotype specificity of B-haplotype influence on the relative efficacy of Marek's disease vaccines . Avian Diseases , 38 : 65 – 71 .
- Bagust , T.J. and Johnson , M.A. 1995 . Avian infectious laryngotracheitis: virus–host interactions in relation to prospects for eradication . Avian Pathology , 24 : 373 – 391 .
- Baigent , S.J. , Smith , L.P. , Currie , R.J.W. & Nair , V.K. 2007 . Correlation of Marek's disease herpesvirus vaccine virus genome load in feather tips with protection, using an experimental challenge model . Avian Pathology , 36, 467–474.
- Brandly , C.A. and Bushnell , L.D. 1934 . A report of some investigations of infectious laryngotracheitis . Poultry Science , 13 : 212 – 217 .
- Calnek , B.W. and Smith , M.W. 1972 . Vaccination against Marek's disease with cell-free turkey herpesvirus: interference by maternal antibody . Avian Diseases , 16 : 954 – 957 .
- Clarke , J.K. , Robertson , G.M. and Purcell , D.A. 1980 . Spray vaccination of chickens using infectious laryngotracheitis virus . Australian Veterinary Journal , 56 : 424 – 428 .
- Cortes , A.L. , Montiel , E.R. and Gimeno , I.M. 2009 . Validation of Marek's disease diagnosis and monitoring of Marek's disease vaccines from samples collected in FTA cards . Avian Diseases , 53 : 510 – 516 .
- Cortes , A.L. , Montiel , E.R. , Lemiere , S. & Gimeno , I.M. 2011 . Comparison of blood and feather pulp samples for the diagnosis of Marek's disease and for monitoring Marek's Disease vaccination by real time PCR Avian Diseases , accepted for publication.
- Cui , X. , Lee , L.F. , Reed , W.M. , Kung , H.J. and Reddy , S.M. 2004 . Marek's disease virus-encoded vIL-8 gene is involved in early cytolytic infection but dispensable for establishment of latency . Journal of Virology , 78 : 4753 – 4760 .
- Davison , S. , Gingerich , E.N. , Casavant , S. and Eckroade , R.J. 2006 . Evaluation of the efficacy of a live fowlpox-vectored infectious laryngotracheitis/avian encephalomyelitis vaccine against ILT viral challenge . Avian Diseases , 50 : 50 – 54 .
- Devlin , J.M. , Browning , G.F. , Gilkerson , J.R. , Fenton , S.P. and Hartley , C.A. 2008 . Comparison of the safety and protective efficacy of vaccination with glycoprotein-G-deficient infectious laryngotracheitis virus delivered via eye-drop, drinking water or aerosol . Avian Pathology , 37 : 83 – 88 .
- Dufour-Zavala , L. 2008 . Epizootiology of infectious laryngotracheitis and presentation of an industry control program . Avian Diseases , 52 : 1 – 7 .
- Gelenczei , E.F. and Marty , E.W. 1965 . Strain stability and immunologic characteristics of a tissue-culture-modified infectious laryngotracheitis virus . Avian Diseases , 14 : 44 – 56 .
- Gimeno , I.M. , Cortes , A.L. and Silva , R.F. 2008 . Load of challenge Marek's disease virus DNA in blood as a criterion for early diagnosis of Marek's disease tumors . Avian Diseases , 52 : 203 – 208 .
- Gimeno , I.M. , Cortes , A.L. , Montiel , E.R. , Lemiere , S. & Pandiri , A.K. 2011 . Effect of diluting Marek's disease vaccines on the outcomes of Marek's disease virus infection when challenged with highly virulent Marek's disease viruses . Avian Diseases , in press.
- Gimeno , I.M. , Witter , R.L. , Hunt , H.D. , Reddy , S.M. , Lee , L.F. and Silva , R.F. 2005 . The pp38 gene of Marek's disease virus (MDV) is necessary for cytolytic infection of B cells and maintenance of the transformed state but not for cytolytic infection of the feather follicle epithelium and horizontal spread of MDV . Journal of Virology , 79 : 4545 – 4549 .
- Guy , J.S. & Bagust , T.J. 2003 . Laryngotracheitis . In Y.M. Saif , H.J. Barnes , A. Fadly , J.R. Glisson , L.R. McDougald & D.E. Swayne . Diseases of Poultry 11th edn 121 – 134 . Ames, Iowa Iowa State University Press .
- Guy , J.S. , Barnes , H.J. and Smith , L.G. 1991 . Increased virulence of modified-live infectious laryngotracheitis vaccine virus following bird-to-bird passage . Avian Diseases , 35 : 348 – 355 .
- Guy , J.S. , Barnes , H.J. , Munger , L.L. and Rose , L. 1989 . Restriction endonuclease analysis of infectious laryngotracheitis viruses: comparison of modified-live vaccine viruses and North Carolina field isolates . Avian Diseases , 33 : 316 – 323 .
- Guy , J.S. , Gimeno , I.M. & West , M. 2011 . Experimental evaluation of in ovo-administered recombinant virus-vectored laryngotracheitis virus vaccines for prevention of disease in broiler chickens . Avian Pathology , submitted.
- Johnson , D.I. , Vagnozzi , A. , Dorea , F. , Riblet , S.M. , Mundt , A. , Zavala , G. and Garcia , M. 2010 . Protection against infectious laryngotracheitis by in ovo vaccination with commercially available viral vector recombinant vaccines . Avian Diseases , 54 : 1251 – 1259 .
- King , D.D. , Page , D. , Schat , K.A. and Calnek , B.W. 1981 . Difference between influences of homologous and heterologous maternal antibodies on response to serotype-2 and serotype-3 Marek's disease vaccines . Avian Diseases , 25 : 74 – 81 .
- Kuhnlein , U. , Spencer , J.L. , Chan , M. , Praslickova , D. , Linher , K. , Kulenkamp , A. and Ansah , G. 2006 . Relationship between Marek's disease and the time course of viral genome proliferation in feather tips . Avian Diseases , 50 : 173 – 178 .
- Landman , W.J. and Verschuren , S.B. 2003 . Titration of Marek's disease cell-associated vaccine virus (CVI 988) of reconstituted vaccine and vaccine ampoules from Dutch hatcheries . Avian Dieases , 47 : 1458 – 1465 .
- Lee , L.F. and Witter , R.L. 1991 . Humoral immune responses to inactivated oil-emulsified Marek's disease vaccine . Avian Diseases , 35 : 452 – 459 .
- Lee , L.E. , Witter , R.L. , Reddy , S.M. , Wu , P. , Yanagida , N. and Yoshida , S. 2003 . Protection and synergism by recombinant fowl pox vaccines expressing multiple genes from Marek's disease virus . Avian Diseases , 47 : 549 – 558 .
- Lupiani , B. , Lee , L.F. , Cui , X. , Gimeno , I.M. , Anderson , A. Morgan , R.W. 2004 . Marek's disease virus-encoded Meq gene is involved in transformation of lymphocytes but is dispensable for replication . Proceedings of the National Acadamey of Science of the United States of America , 101 : 11815 – 11820 .
- Morgan , R.W. , Kent , J. & Anderson , A.S. 1998 . Marek's disease virus gC and meq mutants with dramatic reductions in tumour incidences and horizontal transmission . Avian Pathology , 27 , S88 – S89
- Nazerian , K. , Witter , R.L. , Lee , L.F. and Yanagida , N. 1996 . Protection and synergism by recombinant fowl pox vaccines expressing genes from Marek's disease virus . Avian Diseases , 40 : 368 – 376 .
- Purcell , D.A. and Surman , P.G. 1974 . Letter: Aerosol administration of the SA-2 vaccine strain of infectious laryngotracheitis virus . Australian Veterinary Journal , 50 : 419 – 420 .
- Robertson , G.M. and Egerton , J.R. 1981 . Replication of infectious laryngotracheitis virus in chickens following vaccination . Australian Veterinary Journal , 57 : 119 – 123 .
- Samberg , Y. & Aronovici , I. 1969 . The development of a vaccine against avian infectious laryngotracheitis. 1. Modification of a laryngotracheitis virus . Refuah Veterinarith , 26 , 54 – 59
- Schumacher , D. , Tischer , B.K. , Fuchs , W. and Osterrieder , N. 2000 . Reconstitution of Marek's disease virus serotype 1 (MDV-1) from DNA cloned as a bacterial artificial chromosome and characterization of a glycoprotein B-negative MDV-1 mutant . Journal of Virology , 74 : 11088 – 11098 .
- Swaggerty , C.L. , Pevzner , I.Y. , Kaiser , P. & Kogut , M.H. 2008 . Profiling pro-inflammatory cytokine and chemokine mRNA expression levels as a novel method for selection of increased innate immune responsiveness . Veterinary Immunology and Immunopathology , 126 , 35 – 42
- Tong , G.Z. , Zhang , S.J. , Meng , S.S. , Wang , L. , Qiu , H.J. Wang , Y.F. 2001 . Protection of chickens from infectious laryngotracheitis with a recombinant fowlpox virus expressing glycoprotein B of infectious laryngotracheitis virus . Avian Pathology , 30 : 143 – 148 .