Abstract
The Rispens (CVI988) vaccine is widely used to vaccinate chickens worldwide. We tested the protective effects of the Rispens vaccine against challenge with very virulent Marek's disease virus (vvMDV) at various intervals at, before or after vaccination. The experiment used commercial ISA Brown layers and vvMDV isolate 02LAR. The protective index (PI) was measured for vaccination challenge intervals (VCI) of −10, −5, 0, 5 and 10 days, with the negative values indicating challenge prior to vaccination. Chickens were challenged by injection with 400 plaque-forming units (PFU) of 02LAR and/or vaccinated with 3200 PFU of the Rispens vaccine virus at days 0, 5 and 10 of age, with appropriate negative controls injected with diluent only. The presence of visible Marek's disease tumours was assessed up to 56 days post challenge. MDV challenge in unvaccinated chickens resulted in tumours in 52% of chickens. The Rispens vaccine provided no significant protection when challenge preceded vaccination, with PIs of −4 and 21% for VCI of −5 and −10 days respectively. On the other hand, it provided PIs of 60, 85 and 100% at VCI of 0, 5 and 10 days respectively. The study also revealed that the vvMDV load in peripheral blood lymphocytes or feather tips at 14 and 21 days post infection as determined by quantitative real-time polymerase chain reaction, which can distinguish pathogenic MDV from the Rispens vaccine strain, was an accurate early predictor of Marek's disease incidence at 56 days post challenge. The load of Rispens virus in peripheral blood lymphocytes or feathers at the same times post vaccination did not offer similar predictive power.
Introduction
Marek's disease (MD) is an economically significant neoplastic disease of chickens that can cause severe losses to the poultry industry. Marek's disease virus (MDV) is an alphaherpesvirus of the genus Mardivirus that has three species, namely gallid herpesvirus type 2, gallid herpesvirus type 3 and meleagrid herpesvirus type 1, which are commonly referred to as MDV serotype 1 (MDV-1), MDV serotype 2 (MDV-2) and turkey herpesvirus (HVT) respectively (Osterrieder & Vautherot, Citation2004). Among all of the serotypes, only MDV-1 causes clinical disease in chickens; the other two species are non-pathogenic and widely used as vaccines to protect against MD (Schat & Calnek, Citation1978; Witter, Citation1982; Afonso et al., Citation2001). The pathogenic MDV-1 viruses may be further classified on the basis of virulence using a range of methods. One widely used classification system sub-divides MDV-1 into mild (m) MDV, virulent (v) MDV, very virulent (vv) MDV, and very virulent plus (vv+) MDV strain levels of virulence determined in standardized tests in naive and vaccinated chickens (Witter, Citation1997).
MD is the first oncogenic disease in any species successfully controlled by vaccination that was introduced in 1970 (Churchill et al., Citation1969). This vaccine was based on the oncogenic HPRS-16 strain of MDV-1 that had been attenuated by serial passages in chicken kidney cell culture (Churchill et al., Citation1969). This vaccine was replaced by the naturally avirulent HVT vaccine (FC126 strain), which was shown to be highly effective in preventing MD (Okazaki et al., Citation1970; Ianconescu et al., Citation1971; Purchase & Okazaki, Citation1971; Burmester et al., Citation1972). MDV isolates of increasing virulence have been isolated since the 1950s and, after the introduction of vaccines, this became associated with vaccine failure (Witter, Citation1998). When failure of protection with HVT occurred, a bivalent (HVT + MDV-2) vaccine was introduced in 1983 giving better protection against vvMDV (Calnek et al., Citation1983; Witter et al., Citation1984). Again in the early 1990s, major outbreaks occurred associated with emergence of a new pathotype of MDV, namely vv+MDV, and failure of bivalent vaccine protection (Witter, Citation1996, Citation1997). Rispens (CVI988) vaccine, an attenuated mild MDV-1, provided superior protection against vv+MDV (Witter et al., Citation1995). In earlier studies both HVT and Rispens provided excellent protection against MD (Vielitz & Landgraf, Citation1971; Bulow et al., Citation1976; Maas et al., Citation1982), but in later studies the Rispens vaccine provided better protection against highly virulent challenge strains of MDV (Witter et al., Citation1995). In Australia, Rispens was introduced in 1997 to control a major MD outbreak against which neither HVT nor MDV-2 were protective (Jackson, Citation2000).
After vaccination, MD vaccine viruses establish a persistent infection, which reduces early viraemia following challenge with pathogenic MDV, and protects against tumour formation and mortality, thus reducing the economic consequences of infection with MDV (Morimura et al., Citation1998; Baaten et al., Citation2004). Although both MD vaccines and selection for host resistance are effective at reducing lymphoma formation and decrease viral replication, they do not completely prevent host infection and spread of the virus (Cole, Citation1968; Okazaki et al., Citation1970; Rispens et al., Citation1972; Islam et al., Citation2007). The mechanisms of vaccinal protection at the tissue, cellular and molecular levels are not yet completely understood. However, based on current knowledge the following process can explain vaccine-induced immunity with challenge occurring within 3 days after hatch as is typical in the field (Schat & Nair, Citation2008). As early as 3 days after vaccination or challenge, innate immune effector mechanisms are operative with natural killer cells activated, producing interferon-γ and killing limited numbers of virus-infected B cells. At this stage, other cells of the innate immune system such as macrophages also produce interferon-γ. Interferon-γ can decrease virus replication and activate macrophages to commence the transcription of inducible nitric oxide synthase, producing nitric oxide between 3 and 7 days post vaccination, therefore limiting viral replication, possibly in combination with antibody-dependent cell-mediated cytotoxocity forming part of the MDV-specific adaptive immune response. The combinations of these effector mechanisms push the challenge virus into latency, and memory cytotoxic T lymphocytes of the adaptive response are able to eliminate reactivated virus-infected cells rapidly (Schat & Nair, Citation2008). Due to the time involved for host responses to vaccination or challenge to occur, the interval between vaccination and challenge has been shown to be an important determinant of vaccination success, with protection increasing with vaccination challenge intervals (VCI) up to 7 days in the case of HVT vaccination (Islam et al., Citation2007, Citation2008).
Investigation of the kinetics of vaccine and challenge virus in the same host will advance understanding of the mechanisms of vaccinal protection and of the effects of vaccination on the evolution of virulence. Such studies have already been done for pathogenic MDV-1 in hosts co-infected with HVT and MDV-2 vaccines using serotype-specific real-time quantitative polymerase chain reaction (qPCR) assays (Islam et al., Citation2006, Citation2008; Renz et al., Citation2012; Walkden-Brown et al., Citation2013). However, there are no equivalent data reported for vaccinal MDV-1 due to an absence of effective quantitative tests that can differentiate between pathogenic and vaccinal MDV-1 in the same host. However, Renz et al. (Citation2013) recently developed an assay to differentiate between Australian pathogenic and vaccinal MDV-1 that enabled investigation of the kinetics of co-infection between these viruses.
In light of the above we designed such a co-infection study that also enables the first investigation of the effect of VCI on protection against challenge in birds vaccinated with Rispens CVI 988 and the correlation of early vaccinal and pathogenic MDV load with the subsequent incidence of MD. This work can contribute to improved understanding of vaccinal protection provided by Rispens CVI 988, and can also assist with determination of vaccination and the future MD status of vaccinated flocks using qPCR measurement of MDV load in peripheral blood lymphocytes (PBL), dust or feathers. This paper reports the vaccinal protection and MD prediction aspects of the study and tests the following hypotheses:
Vaccination with Rispens CVI988 5 or 10 days after MDV challenge will not provide significant protection.
Vaccination 5 or 10 days prior to, or simultaneous with, challenge will provide significant protection and the level of protection will be positively correlated with VCI.
Early measures (up to 21 days) of pathogenic and vaccinal MDV load in PBL, feathers and dust will be good predictors of subsequent MDV status.
Materials and Methods
Experimental design
The experiment involved 600 ISA Brown chickens of a single age group in an incomplete 2 × 2 × 3 × 3 factorial design replicated twice at the isolator level using a total of 24 isolators. Two isolators contained unvaccinated and unchallenged controls external to the design. The control birds were injected with the diluents of both viruses. The factors and levels in the design were: challenge virus, two levels (i.e. unchallenged or challenged with vvMDV isolate 02LAR at 400 plaque-forming units (PFU)/bird); vaccine virus, two levels (i.e. unvaccinated or vaccinated with Rispens CVI988 at 3200 PFU/bird); challenge day, three levels (i.e. at days 0, 5 and 10 of age); and vaccination day, three levels (i.e. at days 0, 5 and 10 of age). Based on challenge and vaccination days there were five different VCI; that is, −10, −5, 0, 5 and 10 days.
There were 12 treatment combinations selected from the complete factorial design as shown in .
Table 1. Summary of treatments showing treatment abbreviations and vaccination challenge interval.
Experimental chickens and management
The experimental chickens were newly hatched female ISA Brown commercial layer chickens. The chickens were unvaccinated but came from a Rispens CVI988-vaccinated parent flock so would have had maternal antibody directed against MDV-1. There were 25 chickens placed in each isolator, initially giving 50 chicks per treatment combination.
Chickens were fed ad libitum on commercial layer starter then finisher (Ridley Agricultural Products, Tamworth, Australia). After 2 days of 24 h light, initially chicks were exposed to a 12 h light:12 h dark lighting cycle in positive-pressure isolators containing autoclaved rice hulls in scratch trays and hanging twine for environmental enrichment. Temperatures declined from 35 to 21°C during the experiment at a rate of 1°C every second day. Birds were inspected twice daily for general well-being and sick birds were euthanized if deemed to be suffering excessively. The experiment was approved by the University of New England Animal Ethics Committee (AEC No. UNE 10/057).
Vaccines, vaccination and challenge virus
The Rispens vaccine (Vaxsafe®RIS, strain Rispens CVI988; Bioproperties Pty Ltd, Ringwood, Vic, Australia) was injected subcutaneously in 200 µl supplied diluent at a dose of 3200 PFU per chicken. Unvaccinated and control chickens were injected with the diluent alone. The challenge Australian MDV 02LAR was also administered subcutaneously in 200 µl diluent. 02LAR was grown to passage 4 on chick embryo fibroblasts before titration and storage in liquid nitrogen until use. Before use the virus was thawed at 37°C in a water bath and diluted with M199 media containing 10% foetal calf serum and antibiotics/antimycotics (all sourced from Invitrogen, Mount Waverley, Victoria, Australia). Unchallenged and control chickens were injected with the respective diluent alone. 02LAR had been pathotyped previously as vvMDV (Renz et al., Citation2012; Walkden-Brown et al., Citation2013). Details of the viruses used in this experiment are shown in .
Table 2. Details of viruses used in the experiment.
Marek's disease diagnosis and scoring
Diagnosis of MD was based on gross pathology observed on post-mortem examination of all dead and euthanized chickens. Carcasses were checked for nodular lesions on the skin. Breast and thigh muscles were inspected for discrete lymphoid tumours or diffuse infiltration. The thymus was inspected for atrophy and scored 0 to 3 in ascending order of severity (0 = normal, 3 = complete or almost complete atrophy). After opening the carcass, the liver, spleen, gonads, kidney, proventriculus, mesenteries, gastrointestinal tract, heart and lungs were examined for gross enlargement and discrete or diffuse MD lesions. The bursa of Fabricius was examined and scored for atrophy as for the thymus. Tumorous enlargement of the spleen and bursa of Fabricius were recorded as gross MD lesions. Chickens without visible MD tumours were treated as negative. Histopathological confirmation was not carried out. All MD lesions were scored 1 to 3 subjectively for severity on an ascending scale based on the size and extent of the lesion.
Sampling
Blood samples, feathers and isolator dust were collected weekly throughout the experiment commencing at 7 days post infection (d.p.i.). Where the vaccine and challenge virus had different d.p.i., chicks were sampled separately for each virus so they could be compared at the same d.p.i. These samples were used to quantify both viruses in PBL, feather tips and dust respectively. Detailed results on the kinetics of the two viruses in these materials will be reported separately but the association between viral load in them up to 21 d.p.i. and the incidence of MD at 56 days post challenge (d.p.c.) is reported in this paper. For blood and feather samples, five birds from each isolator were individually identified with wing tags at the beginning of the experiment and blood and feather samples collected from them serially. Blood samples (approximately 300 µl) were collected into 1.5 ml microfuge tubes pre-loaded with 150 µl of 3% sodium citrate prior to separation of PBL using Ficoll Paque™ PREMIUM (Amersham Biosciences, Uppsala, Sweden). Feathers were sampled initially (7 and 14 d.p.i.) by plucking three to five small feathers from the wing and subsequently (>14 d.p.i.) from the axillary tract. Feathers were stored at −20°C until further processing. Weekly dust samples were collected from the exhaust duct of each isolator and stored at −20°C until further processing. Individual sera were collected at 21 and 56 d.p.i. from all individually tagged chickens and stored at −20°C until further processing.
DNA extraction, differential MDV-1 real-time PCR assays and enzyme-linked immunosorbent assay
DNA was extracted from PBL using the automated X-tractor Gene (Corbett Robotics, Qiagen Corbett, Sydney, NSW, Australia) according to the manufacturer's instructions and reagents. Feather tips were cut into small pieces using a surgical blade and DNA was extracted using the ISOLATE genomic DNA mini kit (Bioline, Alexandria, NSW, Australia) following the instructions given by the manufacturer. Approximately 5 mg dust was collected into a sterile Eppendorf tube and DNA was extracted using the ISOLATE genomic DNA mini kit (Bioline) following the instructions given by the manufacturer. All extracted DNA samples were stored at −20°C until further analysis.
Prior to real-time qPCR, DNA was quantified by spectrophotometric analysis using a NanoDrop® ND-1000 UV–Vis spectrophotometer (NanoDrop® Technologies, Wilmington, DE, USA), and diluted to a concentration of 5 ng/µl1 for use in the qPCR assays. For determination of viral load in various tissues, two TaqMan® qPCR assays were performed using a Rotor Gene 3000 real-time PCR instrument (Qiagen Corbett). One test only detects Rispens CVI988 MDV-1 while the complimentary test only detects wild-type pathogenic MDV-1 (Renz et al., Citation2013). A single reaction was run for each PBL sample and duplicate reactions were run for feather and dust samples. For determination of MDV-specific antibody titre in serum samples, an indirect enzyme-linked immunosorbent assay adapted from that of Zelnik et al. (Citation2004) was used.
Vaccinal protective index
The protective index (PI) provided by the Rispens vaccine against challenge with 02LAR at various VCI was calculated as:
Statistical analysis
Analyses were performed using JMP10 (SAS Institute NC, USA). Two separate analyses were performed. Analysis 1 included only the treatments with effective VCI () and tested the effect of VCI. Analysis 2 included all treatments and tested the effect of treatment as shown in . Where the chicken was the experimental unit measured, categorical data such as mortality (died/survived) or presence of MD (positive/negative) were analysed using the Pearson chi-square statistic test in the case of two-way tables. Mortality data were also analysed using survival analysis (Kaplan–Meier method). The vaccinal PI was calculated for each isolator, and treatment effects were tested using analysis of variance after fitting linear models for analyses 1 and 2. MDV load in PBL, feather and dust were log transformed [Log10(y + 1)] prior to analysis. MD titre data were also analysed after log transformation. Treatment effects were tested using analysis of variance after fitting linear models for analyses 1 and 2. Differences between different levels within a significant main effect were tested using Student's t test. Association between MD incidence and MDV load in different tissues were tested by pairwise correlation and linear regression analysis of specific associations. Two separate analyses were done; one excluding unchallenged treatments and the other excluding unchallenged treatments and unvaccinated treatments. Least-squares means and standard errors of the mean are presented for continuous variables. A significance level of P < 0.05 is used throughout.
Results
Application of treatments
All vaccination and challenge treatments were successfully applied and maintained, as determined by MDV-1 differential real-time qPCR. All of the control chickens were negative for Rispens and pathogenic MDV-1 in PBL, feather and dust at all sampling days. Challenged birds were positive for pathogenic MDV-1 and vaccinated birds were positive for Rispens.
Mortality/survival
The first MD tumours were detected at 31 d.p.c. In total 17.7% birds died in the experiment, of which 13.8% died with gross MD lymphomas. All of the negative controls chickens had zero mortality with MD. Of the 18 chickens that died or were euthanized without gross MD tumours, nine were non-starters/dehydrated, five had yolk sac infection and four were accidentally injured or killed (crushed behind feeder).
Analysis 1 revealed significant effects of VCI (P < 0.0001) for both mortality and mortality with MD lesions. Significantly more birds died in the VCI −5 days treatment (55%) than in all other treatments. This was also the case for mortality with MD lesions (52.5%) (). Analysis 2 showed that overall treatment also had a significant effect (P < 0.0001) on both mortality and mortality with MD lesions. Unvaccinated birds challenged at different days showed higher mortality (27.5, 22.5 and 37.5% after challenge at days 0, 5 and 10 respectively) than unchallenged birds vaccinated at different days (7.7, 0 and 0% for day 0, 5 and 10 respectively) (). Mortality with MD lesions showed similar trends ().
Table 3. Effect of vaccination with Rispens CVI988 vaccine and challenge with vvMDV isolate 02LAR in various time combinations on total mortality, mortality with MD, incidence of MD to 56 d.p.c. and PI by VCI and treatment in commercial ISA Brown chickens
Survival analysis showed a significant effect of VCI (P < 0.0001; ). There was no significant effect of day of challenge in the challenge-only treatments (P = 0.38; , middle panel) whereas there was a significant effect of day of vaccination in vaccination-only treatments (P = 0.0001; , right panel) due mainly to birds that died from miscellaneous causes other than MD.
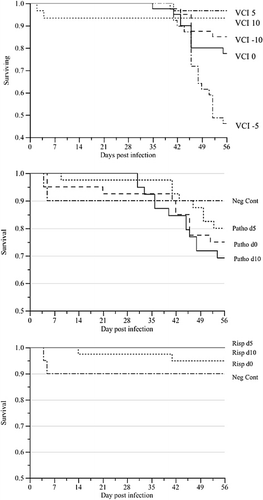
Incidence of Marek's disease and vaccinal protective index
The combined incidence of gross MD lesions in chickens that died with MD or had MD lesions on post-mortem after euthanasia at 56 d.p.c. is summarized by treatment and VCI in . Overall, there was a significant effect of VCI on MD incidence (P = 0.001), with a higher incidence in treatments VCI −10 days (59%) and VCI −5 days (45%) than VCI 0 days (23.1%), VCI 5 days (6.7%) and VCI 10 days (0%). VCI had a significant effect on PI (P = 0.002), with VCI 0, 5 and 10 days showing significantly higher PIs (60.4, 84.8 and 100% respectively) than VCI −5 days (−3.6%) and VCI −10 days (20.9%) (). Not surprisingly, treatment in analysis 2 also had an overall significant effect on MD incidence (P < 0.0001). The incidence ranged from no MD in unchallenged birds through to a mean of 52.6% in challenged but unvaccinated birds ().
On an individual isolator basis there was a significant (P = 0.0008) negative linear association between VCI and MD incidence (R2 = 0.77; a) and a significant (P = 0.0009) positive linear association between VCI and PI (R2 = 0.77; b).
Marek's disease virus-specific serology
Analysis 1 revealed that there was a significant effect of VCI (P = 0.006; ) and d.p.c. (P < 0.0001) on the log10 anti-MDV IgY titre with no significant interaction between these effects (P = 0.1). VCI 10 days showed significantly higher IgY titre (2.05 ± 0.21) than all other VCI, except VCI 5 days where the titre level was non-statistically lower than VCI 10 days. Analysis 2 showed that there was a significant effect of treatment (P < 0.0001) and d.p.c. or vaccination (P < 0.0001) on the log10 MDV IgY titre, with significant interaction between these effects (P = 0.0006; ). There were no significant differences between Rispens-only treatments with vaccine administered at different ages. The Rispens-only treatments also showed significantly higher IgY titre than pathogenic-only treatments at each of the equivalent times of infection.
Relationship between Marek's disease incidence at 56 d.p.c. and MDV load in PBL, feathers, dust, immune organ weights and MD serology
In the analysis where unchallenged isolators are excluded, the pathogenic MDV load in PBL on 14 d.p.c. (R2 = 0.69, P < 0.0001) and 21 d.p.c. (R2 = 0.69, P < 0.0001) were significantly positively associated with MD incidence at 56 d.p.c., but there was no significant relationship at 7 d.p.c. (R2 = 0.20, P = 0.09) (, first row). On the other hand, Rispens load in PBL of chickens on 7, 14 and 21 days post vaccination (d.p.v.) had no significant relationship with MD incidence by 56 d.p.c. with a trend towards a negative association ().
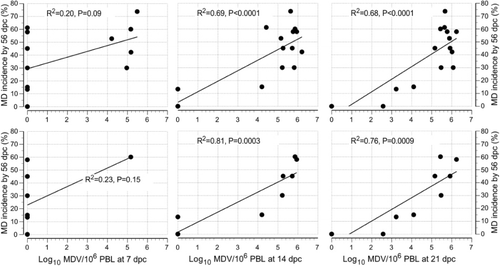
Table 4. Pairwise correlation of MD incidence (MD%) at day 56 with viral load in various tissues, immune organ weight and MD antibody titre of different challenge days.
Pathogenic MDV loads in feather tips on 14 d.p.c. (R2 = 0.26, P = 0.04) and 21 d.p.c. (R2 = 0.70, P < 0.0001) were found significantly positively associated with MD incidence at 56 d.p.c., but there was a significant negative relationship at 7 d.p.c. (R2 = 0.27, P = 0.04) (, first row). On the other hand, Rispens load in feather tips of chickens at 7, 14 and 21 d.p.v. had no significant association with MD incidence to 56 d.p.c. with a trend towards a negative association ().
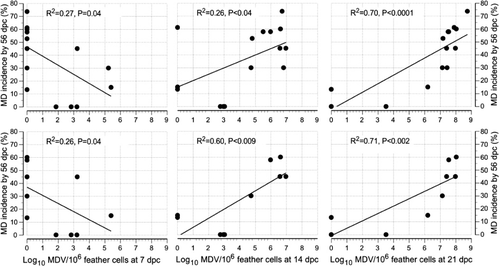
There were no significant associations between pathogenic MDV load in isolator exhaust dust at 7, 14 and 21 d.p.c. and MD incidence to 56 d.p.c. (). There was also no significant relationship between Rispens load in dust and MD incidence to 56 d.p.c. with a trend towards a negative association at 7 and 14 d.p.v. and positive association at 21 d.p.v.
There were also no significant relationships found between MD incidence up to 56 d.p.c. and splenic or bursal weights at 14 and 56 d.p.c. as well as MD antibody titre at 21 and 56 d.p.c. ().
In the analysis where unchallenged isolators and unvaccinated isolators were excluded, MD incidence to 56 d.p.c. was found to be significantly positively associated with pathogenic MDV load at 14 d.p.c. (R2 = 0.81, P = 0.0003) and 21 d.p.c. (R2 = 0.76, P = 0.0009) but not at 7 d.p.c. (R2 = 0.23, P = 0.15) (, second row). On the other hand it was not associated with Rispens load in PBL of chickens at 7, 14 and 21 d.p.v. with a trend towards a negative association (). MD incidence at 56 d.p.c. was positively associated with pathogenic MDV load in feather tips on 14 d.p.c. (R2 = 0.60, P = 0.009) and 21 d.p.c. (R2 = 0.71, P = 0.002) but there was a non-significant negative relationship at 7 d.p.c. (R2 = 0.26, P = 0.13) (, second row). On the other hand it was not significantly associated with Rispens load in feather cells of chickens at 7, 14 and 21 d.p.v. with a trend towards a negative association ().
Table 5. Pairwise correlation of MD incidence (MD%) at d56 with viral load in various tissues, immune organ weight and MD antibody titre of different challenge days.
There were no significant associations between pathogenic MDV loads in isolator exhaust dust at 7, 14 and 21 d.p.c. with MD incidence up to 56 d.p.c. (). There was also no significant association between Rispens load in dust and MD incidence by 56 d.p.v. with a trend towards negative associations at 7 and 14 d.p.v. and a positive association at 21 d.p.v. In this analysis there were also no significant associations between MD incidence to 56 d.p.c. and splenic and bursal weights at 14 and 56 d.p.c., or MD antibody titre at 21 and 56 d.p.c. ().
Discussion
This study has demonstrated that VCI have a significant effect on the protective efficacy of the Rispens/CVI988 vaccine, with full protection observed only when vaccination occurred 10 days before challenge, and no significant protection occurring if challenge precedes vaccination by 5 or 10 days. Pathogenic MDV viral load in PBL and feather tips at 14 and 21 d.p.c., but not 7 d.p.c., was a good predictor of subsequent MD incidence, whereas the Rispens CVI988 viral load in PBL and feather tips was not.
The first hypothesis was supported because vaccination with Rispens, 5 or 10 days after challenge with vvMDV provided no significant protection against MD. The second hypothesis was also supported, as there was significant protection against MD when challenge occurred concurrently with vaccination or at days 5 or 10 following it. The respective PI values were 60.4, 84.8 and 100% with a highly significant positive linear association between PI and VCI on an individual isolator basis (). These data suggest that maximal protection is obtained by day 10 following vaccination. This is supported by the findings of Baigent et al. (Citation2007) of uniformly high PI (> 90%) in anti-MDV maternal antibody-negative chickens vaccinated with Rispens CVI988 at 1 day of age and challenged with MDV at 14, 21 and 28 d.p.v. A positive association between VCI and PI has also been reported in other studies. Islam et al. (Citation2007) reported that vaccination of maternal anti-MDV antibody-positive broilers with a range of HVT doses induced higher protection against MDV challenge at VCI of 5 days (mean PI of 79%) than VCI of 2 days (mean PI of 15%). Similarly, Islam et al. (Citation2008) reported PI of HVT vaccination against MDV challenge in maternal antibody-positive broilers of 48, 69 and 77% for VCI of 2, 4 and 7 days respectively. In a second experiment they reported PI of 66, 33, 53, 76 and 76% for VCI of 0, 2, 4, 7 and 10 days respectively, concluding that for HVT no improvement in protection is obtained beyond VCI of 7 days. Interestingly the latter experiment also showed significant protection when the vaccine and challenge virus were co-administered, an observation also seen in the present experiment. In all studies using maternal antibody-positive chickens, those with longer VCI are challenged later, and thus have lower levels of maternal antibody directed against MDV at challenge time than those with shorter or negative VCI. It is established that the presence of maternal antibody directed against MDV slows the pathogenesis of MD (Chubb & Churchill, Citation1969), presumably by slowing the MDV replication rate. It is therefore possible that part of the observed effect of VCI is mediated by a reduced maternal antibody (passive immunity) inhibition of challenge virus, in addition to the enhanced active immunity expected when the immune response has time to develop. The significantly higher mortality rate with MD in chickens with VCI of −5 days relative to those with VCI of −10 days was unexpected. Chicks in both cases were challenged with MDV at day 0. Possibly vaccination at day 5 rather than day 10 of age resulted in greater maternal antibody inhibition of the homologous vaccine virus. Alternatively, vaccination during the early cytolytic phase of pathogenesis at 5 d.p.c. facilitates MD pathogenesis relative to vaccination at 10 d.p.c. during the latent phase of pathogenesis. One should note that many factors in addition to VCI affect the efficiency of vaccinal protection against MD. These include chicken genotype, maternal antibody status, vaccine virus batch and dose and the level and route of challenge, which may interact to alter the optimum VCI. The 10-day optimum VCI observed in this experiment is therefore likely to be indicative, rather than applicable to all circumstances.
The PI provided by the Rispens vaccine in the experiment is numerically greater than that provided by HVT or HVT/MDV-2 against the same challenge virus in the same chicken strain some years ago. Renz (Citation2008) reported PI of 27.2 and 63.1% for HVT and HVT/SB1 vaccines administered to ISA brown chicks at hatch, followed by challenge with 500 PFU of 02LAR at day 5 (VCI = 5 days). This compares with a value of 85% for VCI 5 days in the present experiment. This supports field experience that the Rispens CVI988 vaccine is one of the most effective vaccines against MDV currently available.
With regard to the third hypothesis, pathogenic MDV viral copy number in PBL and feather, but not dust, measured at 14 and 21 d.p.c. provided very good prediction of subsequent MD incidence. However, the relationship was much weaker at 7 d.p.c. in PBL (R2 = 0.23) and at 7 d.p.c. in feather cells (R2 = 0.26). On the other hand, Rispens load in PBL at 7, 14 and 21 d.p.v. and in feather cells at 7, 14 and 21 d.p.v. showed mostly weak non-significant negative associations with subsequent MD incidence (PBL, R2 = 0.06 to 0.56; feather cells, R2 = 0.06 to 0.11). Other studies have shown that the load of MDV in lymphocytes or splenocytes in the first few weeks after challenge are good predictors of subsequent MD status (Islam et al., Citation2006, Citation2007, Citation2008; Gimeno et al., Citation2008; Walkden-Brown et al., Citation2013) but that the load of HVT is not (Islam et al., Citation2006; Gimeno et al., Citation2008). Our results are consistent with these earlier findings in chickens vaccinated HVT and or MDV-2 vaccines. In the case of isolator exhaust dust no significant associations were found between either pathogenic MDV-1 or Rispens virus on subsequent MD incidence. This contrasts with the findings of Walkden-Brown et al. (Citation2013), who showed that MDV loads in dust at 14 and 21 d.p.c. were powerful early predictors of subsequent MD status in commercial broiler chickens.
There were also no significant relationships found between relative immune organ weights or MD IgY titre at 21 and 56 d.p.c. and MD incidence to 56 d.p.c. (). However, Renz (Citation2008) showed significant association between subsequent MD status at 56 d.p.c. and relative bursal weight at 14 and 56 d.p.c. but no significant association with splenic weight. Previous studies showed that in commercial broiler chickens the association between relative immune organ weights and subsequent MD status is very strong (Renz et al., Citation2012).
From the above discussion it can be concluded that the viral load of pathogenic MDV in PBL and in feather tips at 14 and 21 d.p.c. is the best predictor of subsequent MD incidence. This finding supports that of Islam et al (Citation2006) that quantitation of MDV in PBL of broiler chickens (Renz et al., Citation2012) during the early stages of infection has predictive value for subsequent MD incidence. Other studies have shown that MDV load in spleen is an excellent predictor of future MD in specific pathogen free, commercial broiler and layer chickens (Islam et al., Citation2007; Renz, Citation2008; Renz et al., Citation2012; Walkden-Brown et al., Citation2013), suggesting that MDV load in circulating PBL or splenocytes may be equally good early predictors of subsequent MD incidence.
Anti-MDV antibody titres in chickens injected with the Rispens vaccine only on days 0, 5 and 10 were significantly higher than those of chickens challenged with pathogenic MDV only on the same days (). This may be due to the higher dose of Rispens vaccine used compared with 02LAR (3200 PFU vs. 400 PFU), or possibly the Rispens virus is more immunogenic.
We also found that the 56 d.p.i. antibody titres were significantly higher than the 21 d.p.i. titres in all treatments (), indicating an active response to infection, and the probable initial inhibitory effect of maternal antibody directed against MDV. There was also a broadly positive relationship between antibody titre and VCI () and thus with PI. Interestingly, administration of pathogenic MDV in addition to the Rispens vaccine virus generally reduced antibody titres, except with VCI of 10 days (). This suggests that pathogenic MDV interferes with the immune response to Rispens vaccination. Given the well-documented immunosuppressive effects of pathogenic MDV infection due to lytic infection of B and T lymphocytes this is not surprising, and may also explain the lower antibody titres induced by pathogenic MDV alone, compared with vaccinal Rispens virus alone.
Measuring the level of specific antibody is generally an effective way to determine vaccination success. However, the antibody response to pathogenic and vaccinal MDV cannot be differentiated by standard immunological tests. Moreover, in maternal antibody-positive commercial chickens, interpretation of early titres is confounded by passive maternal antibody and active responses to vaccination are very low or non-detectable at 21 d.p.v. as we observed in the present experiment (). On the other hand, differential qPCR can detect the viral load of different viruses from day 7 and provides a good prediction of future MD status at days 14 and 21. Thus tests for vaccinal success based on qPCR quantification of vaccinal or pathogenic MDV offer significant advantages. Unfortunately, in the present experiment no significant association between pathogenic or vaccinal MDV in isolator exhaust dust and future MD status was observed. The reasons for this are not clear given the good associations reported previously (Walkden-Brown et al., Citation2013). It is of concern that in the present experiment many dust samples from isolators known to have MDV active in them were negative for MDV. This is suggestive of failure of MDV detection (false negatives) in dust by the methods used in this experiment.
The survival rate of unvaccinated but challenged chickens to 56 d.p.c. was comparatively high, being 72.5, 77.5 and 62.5% for chickens challenged with pathogenic virus at day 0, day 5 and day 10 respectively. It is also interesting to note that there was none of the early paralysis and mortality observed between 9 and 15 d.p.c. with the same challenge virus in specific pathogen free chickens (Renz et al., Citation2012). The higher survival rate and absence of early paralysis and mortality in the present experiment is most probably due to the protective effect of maternal antibody directed against MDV, which is well known to inhibit or delay the pathogenesis of MD (Chubb & Churchill, Citation1969). Although not directly measured, such maternal antibody would have been present in chickens in the current experiment as they came from parents vaccinated against MD. Similarly high survival rates and an absence of early mortality have been reported in unvaccinated commercial broiler chickens challenged with 02LAR (Walkden-Brown et al., Citation2013). These chickens would have been maternal antibody-positive because they were hatched from parents vaccinated against MD.
In summary, we can conclude that: the Rispens CVI988 vaccine provides increasing levels of protection as VCI increased from 0 to 10 days with 100% protection observed at VCI of 10 days; the Rispens CVI988 vaccine provides no significant protection if chickens are vaccinated 5 or 10 days after challenge with vvMDV; and qPCR quantitation of pathogenic MDV-1 in PBL or feathers at 14 to 21 d.p.c. is highly predictive of MD incidence at 56 d.p.c., whereas quantitation of the Rispens vaccine virus provides little predictive value.
Acknowledgement
This work was supported by funding from the University of New England and Australian Egg Corporation Limited, for which the authors are grateful. The technical assistance of Susan Burgess, Michel Raue, Sara Bowers, Gary Taylor and Grahame Chaffey is also gratefully acknowledged.
References
- Afonso, C.L., Tulman, E.R., Lu, Z., Zsak, L., Rock, D.L. & Kutish, G.F. (2001). The genome of turkey herpesvirus. Journal of Virology, 75, 971–978. 10.1128/JVI.75.2.971-978.2001
- Baaten, B.J.G., Butter, C. & Davison, T. (2004). Study of host-pathogen interactions to identify sustainable vaccine strategies to Marek's disease. Veterinary Immunology and Immunopathology, 100, 165–177. 10.1016/j.vetimm.2004.04.009
- Baigent, S.J., Smith, L.P., Currie, R.J.W & Nair, V.K. (2007). Correlation of Marek's disease herpesvirus vaccine virus genome load in feather tips with protection, using an experimental challenge model. Avian Pathology, 36, 467–474. 10.1080/03079450701639319
- Bulow, V.V., Landgraf, H. & Vielitz, E. (1976). Differentiation between antibodies against turkey herpesvirus and Marek's disease virus in field sera. Zentralblatt für Veterinarmedizin Reihe B-Journal of Veterinary Medicine Series B-Infectious Diseases Immunology Food Hygiene Veterinary Public Health, 23, 391–402. 10.1111/j.1439-0450.1976.tb01620.x
- Burmester, B.R., Purchase, H.G. & Okazaki, W. (1972). Long-term experiences with the herpesvirus of turkeys (HVT) as a vaccine against Marek's disease. Progress in Immunobiological Standards, 5, 132–138.
- Calnek, B.W., Schat, K.A., Peckham, M.C. & Fabricant, J. (1983). Field trials with a bivalent vaccine (HVT and SB-1) against Marek's disease. Avian Diseases, 27, 844–849. 10.2307/1590330
- Chubb, R.C. & Churchill, A.E. (1969). Effect of maternal antibody on Marek's disease. The Veterinary Record, 85, 303–305. 10.1136/vr.85.11.303
- Churchill, A.E., Payne, L.N. & Chubb, R.C. (1969). Immunization against Marek's disease using a live attenuated virus. Nature, 221, 744–747. 10.1038/221744a0
- Cole, R.K. (1968). Studies on genetic resistance to Marek's disease. Avian Diseases, 12, 9–28. 10.2307/1588081
- Gimeno, I.M., Cortes, A.L. & Silva, R.F. (2008). Load of challenge Marek's disease virus DNA in blood as a criterion for early diagnosis of Marek's disease tumors. Avian Diseases, 52, 203–208. 10.1637/8089-081407-Reg.1
- Ianconescu, M., Purchase, H.G., Okazaki, W. & Burmester, B.R. (1971). Influence of natural exposure on response to subsequent challenge with virulent Marek's disease. Avian Diseases, 15, 745–752. 10.2307/1588863
- Islam, A. & Walkden-Brown, S.W. (2007). Quantitative profiling of the shedding rate of the three Marek's disease virus (MDV) serotypes reveals that challenge with virulent MDV markedly increases shedding of vaccinal viruses. Journal of General Virology, 88, 2121–2128. 10.1099/vir.0.82969-0
- Islam, A.F.M.F., Walkden-Brown, S.W., Groves, P.J. & Underwood, G.J. (2007). Effects of vaccine dose, virus challenge dose and interval from vaccination to challenge on protection of broiler chickens against Marek's disease virus challenge. Australian Veterinary Journal, 85, 348–355. 10.1111/j.1751-0813.2007.00195.x
- Islam, A.F.M.F., Walkden-Brown, S.W., Groves, P.J. & Underwood, G.J. (2007). Effects of HVT vaccine dose, Marek's disease virus (MDV) challenge dose and vaccination to challenge interval on protection against MDV challenge in broiler chickens. Australian Veterinary Journal, 85, 348–355. 10.1111/j.1751-0813.2007.00195.x
- Islam, A.F.M.F., Walkden-Brown, S.W., Groves, P.J. & Underwood, G.J. (2008). Kinetics of Marek's disease virus (MDV) infection in broiler chickens 1: Effect of varying vaccination to challenge interval on vaccinal protection and load of MDV and herpesvirus of turkey in the spleen and feather dander over time. Avian Pathology, 37, 225–235. 10.1080/03079450701802230
- Islam, A.F.M.F., Walkden-Brown, S.W., Islam, A., Underwood, G.J. & Groves, P.J. (2006). Relationship between Marek's disease virus load in PBL at various stages of infection and clinical Marek's disease in broiler chickens. Avian Pathology, 35, 42–48. 10.1080/03079450500465734
- Jackson, C.A.W. (2000). Lessons from 30 years of Marek's disease control in Australia for 2000 and beyond. Proceedings of Australian Poultry Science Symposium, 12, 186–189.
- Maas, H.J.L., Borm, F. & van Kieft, G. (1982). The prevention of Marek's disease in Holland by vaccination with cell-associated vaccines—results of field trials and routine vaccinations between 1970–1980. World's Poultry Science Journal 38, 163–175. 10.1079/WPS19820012
- Morimura, T., Ohashi, K., Sugimoto, C. & Onuma, M. (1998). Pathogenesis of Marek's disease (MD) and possible mechanisms of immunity induced by MD vaccine. Journal of Veterinary Medical Science, 60, 1–8. 10.1292/jvms.60.1
- Okazaki, W., Purchase, H.G. & Burmester, B.R. (1970). Protection against Marek's disease by vaccination with a herpesvirus of turkeys. Avian Diseases, 14, 413–429. 10.2307/1588488
- Osterrieder, K. & Vautherot, J.-F. (2004). The genome content of Marek's disease-like viruses. In F. Davison & V. Nair (Eds.). Marek's Disease. An Evolving Problem (pp.17–31). Amsterdam: Elsevier.
- Purchase, H.G. & Okazaki, W. (1971). Effect of vaccination with herpesvirus of turkeys (HVT) on horizontal spread of Marek's disease herpesvirus. Avian Diseases, 15, 391–397. 10.2307/1588710
- Renz, K.G. (2008). In vitro and in vivo characterization of selected Australian isolates of Marek's disease virus. Doctor of Philosophy Thesis, University of New England, Armidale.
- Renz, K.G., Cheetham, B.F. & Walkden-Brown, S.W. (2013). Differentiation between pathogenic serotype 1 isolates of Marek's disease virus and the Rispens CVI988 vaccine in Australia using real-time PCR and high resolution melt curve analysis. Journal of Virological Methods, 187, 144–152. 10.1016/j.jviromet.2012.09.018
- Renz, K.G., Cooke, J., Clarke, N., Cheetham, B.F., Hussain, Z., Islam, A.F.M.F., Tannock, G.A. & Walkden-Brown, S.W. (2012). Pathotyping of Australian isolates of Marek's disease virus and association of pathogenicity with meq gene polymorphism. Avian Pathology, 41, 161–176. 10.1080/03079457.2012.656077
- Rispens, B.H., van Vloten, H., Mastenbroek, N., Maas, H.J., & Schat, K.A. (1972). Control of Marek's disease in the Netherlands. I. Isolation of an avirulent Marek's disease virus (strain CVI 988) and its use in laboratory vaccination trials. Avian Diseases, 16, 108–125. 10.2307/1588905
- Schat, K.A. & Calnek, B.W. (1978). Characterization of an apparently nononcogenic Marek's disease virus. Journal of the National Cancer Institute, 60, 1075–1082.
- Schat, K.A. & Nair, V. (2008). Marek's disease. In Y.M. Saif, A.M. Fadly, J.R. Glisson, L.R. McDougald, L.K. Nolan & D.E. Swayne (Eds.), Diseases of Poultry 12th edn (pp. 452–514). Ames, USA: Blackwell Publising Professional.
- Vielitz, E. & Landgraf, H. (1971). Impfversuche gegen Marek'sche Krankheit unter verwendung von attenuiertem Marek-Herpes-Virus und Puten-herpes-virus. Deutsche Tierartzliche Wochenschrift, 78, 617–623.
- Walkden-Brown, S.W., Islam, A., Islam, A.F.M.F., Burgess, S.C., Groves, P. & Cooke, J. (2013). Pathotyping of Australian isolates of Marek's disease virus in commercial broiler chickens vaccinated with HVT or bivalent (HVT/SB1) vaccine and association with viral load in spleen and feather dander. Australian Veterinary Journal.
- Witter, R.L. (1996). Evolution of virulence of Marek's disease virus: Evidence for a novel pathotype. Current Research on Marek's Disease, 86–91.
- Witter, R.L. (1997). Increased virulence of Marek's disease virus field isolates. Avian Diseases, 41, 149–163. 10.2307/1592455
- Witter, R.L. (1998). The changing landscape of Marek's disease. Avian Pathology, 27, S46–S53. 10.2307/1592455
- Witter, R.L. (1982). Protection by attenuated and polyvalent vaccines against highly virulent strains of Marek's disease virus. Avian Pathology, 11, 49–62. 10.1080/03079458208436081
- Witter, R.L., Lee, L.F. & Fadly, A.M. (1995). Characteristics of CV1988/Rispens and R2/23, 2 prototype vaccine strains of serotype-1 Marek's disease virus. Avian Diseases, 39, 269–284. 10.2307/1591868
- Witter, R.L., Sharma, J.M., Lee, L.F., Opitz, H.M. & Henry, C.W. (1984). Field trials to test the efficacy of polyvalent Marek's disease vaccines in broilers. Avian Diseases, 28, 44–60. 10.2307/1590127
- Zelnik, V., Harlin, O., Fehler, F., Kaspers, B., Gobel, T.W., Nair, V.K. & Osterrieder, N. (2004). An enzyme-linked immunosorbent assay (ELISA) for detection of Marek's disease virus-specific antibodies and its application in an experimental vaccine trial. Journal of Veterinary Medicine Series B-Infectious Diseases and Veterinary Public Health, 51, 61–67. 10.1111/j.1439-0450.2004.00728.x