Abstract
Congenital cerebellar anomalies have been rarely reported in birds. We examined cerebellums with disorganized folia from seven specific-pathogen-free White Leghorn chickens (Gallus gallus domesticus). Islands of heterotopic cortex were distributed from the deeper cortices to the medulla in the cerebellum. The characteristic lesions were composed of randomly admixed components of the cerebellar cortex, including Purkinje cells, a molecular layer and granular cells. Immunofluorescent analysis revealed Purkinje cells with haphazardly extended dendrites and a lack of Bergmann's glial fibres in the foci. Chicken parvovirus, Aino virus and avian retrovirus were not detected in the affected birds by polymerase chain reaction. This is the first report of cerebellar dysplasia in chickens possibly caused by a genetic abnormality.
Introduction
Congenital cerebellar anomalies are common in domestic animals and are classified into hypoplasia, abiotrophy and dysplasia (Maxie & Youssef, Citation2007). Cerebellar hypoplasia secondary to an in utero or perinatal viral infection is most commonly seen in domestic animals, including cats, cattle and pigs. Cerebellar abiotrophy refers to the premature or accelerated degeneration of formed elements, presumably caused by some intrinsic metabolic defect. Cerebellar dysplasia is a rare entity in domestic animals, with the exception of mouse cerebellar mutants studied in developmental biology (Rice & Curran, Citation1999). The cerebellar disorder has been described as a part of Dandy–Walker syndrome, Arnold–Chiari malformation, copper deficiency in sheep and some metabolic storage disorders, although minor dysplastic lesions of no consequence are common in young animals (Maxie & Youssef, Citation2007). In contrast to mammals, congenital cerebellar abnormalities have been rarely reported in birds. Cerebellar hypoplasia due to viruses, including a chicken parvovirus, avian leukosis viruses (ALVs) and an Aino virus, has been described previously (Kitano et al., Citation1997; Toyoda et al., Citation2006; Marusak et al., Citation2010). Cerebellar hypoplasia, suspected of being a genetic disorder, has also been described in two free-living American kestrels (Armién et al., Citation2013). Here, we describe the morphological characteristics of cerebellar dysplasia in specific-pathogen-free (SPF) White Leghorn chickens.
Materials and Methods
Birds
The seven chickens examined in this study were part of the control group of an avian leukosis virus experiment. Fertilized eggs from a SPF White Leghorn strain were purchased from a commercial vendor. All eggs were incubated at 37.5°C and inoculated via the yolk sac on the sixth day of incubation with 0.1 ml pathogen-free culture medium. The culture medium was Dulbecco's modified Eagle's medium (Nissui Pharmaceutical Co., Tokyo, Japan) added with 3500 mg/l d(+)-glucose (Wako Pure Chemical Industries Ltd, Osaka, Japan) and 4 mM l-glutamine (Life Technologies Corp., Carlsbad, California, USA). The hatched chicks were reared in isolators in the animal facilities of our school, and were euthanized at 0 and 35 days of age. The chickens did not receive any vaccinations or medication. All experiments were performed humanely according to the guidelines set by the Hokkaido University Veterinary Medicine Animal Care and Use Committee.
Histopathology and immunohistochemistry
The brain and other organs, including the liver, spleen, kidneys, heart, lungs, bone marrow, gastrointestinal tract, bursa of Fabricius, thymus, spinal cord, peripheral nerves and skeletal muscles, were fixed in neutral-buffered 20% formalin, embedded in paraffin wax, sectioned at 4 µm thickness, and examined by light microscopy after staining with haematoxylin and eosin. To visualize the Purkinje cell axons, Bodian stain was used.
For the immunohistochemical examination, selected sections were deparaffinized. The sections for vimentin evaluation were pretreated with trypsin (0.1% in 0.1 M Tris buffer) for 30 min at 37°C, and other sections for glial fibrillary acid protein and proliferating cell nuclear antigen were pre-treated with microwaves. The sections were treated with 0.3% hydrogen peroxide to inhibit endogenous peroxidase. Indirect immunoperoxidase staining was performed using a commercial streptavidin–biotin kit (Nichirei Corp., Tokyo, Japan). After the blocking of non-specific reactions, each section was incubated with specific primary antibodies: against vimentin (1:100, clone Vim3B4; Dako, Copenhagen, Denmark), glial fibrillary acid protein (1:2500; Dako) or proliferating cell nuclear antigen (no dilution; Dako).
Immunofluorescence and confocal microscopy
Sections were cut at 10 µm, deparaffinized and washed twice with phosphate-buffered saline (pH 7.4) containing 0.1% Triton X-100 (PBST) and blocked with 2% bovine serum albumin in PBST for 30 min. A rabbit polyclonal anti-calbindin D28 antibody (1:400; Spring Bioscience, Pleasanton, CA, USA) and a mouse monoclonal anti-vimentin antibody (1:100, clone 3B4; Dako) were used and incubated at 4°C overnight in a humidified chamber. Following three washes in PBST, bound antibodies were detected with Alexa488-conjugated donkey anti-rabbit and Alexa555-conjugated donkey anti-mouse immunoglobulin G (Molecular Probes, Life Technologies, Carlsbad, CA, USA). After three additional washes in PBST, coverslips were mounted with ProLong Gold with bisBenzimide H 33258 (Sigma-Aldrich, St Louis, MO, USA) and examined with a Zeiss LSM-700 confocal microscope (Carl Zeiss Microscopy GmbH, Jena, Germany).
Polymerase chain reaction
Polymerase chain reaction (PCR) was conducted on DNA extracted from frozen brain tissue for ALV, Marek's disease virus serotype 1 and chicken parvovirus as described previously (Chang et al., Citation2002; Hatai et al., Citation2005; Zsak et al., Citation2008; Tarasiuk et al., Citation2012). The PCR products were electrophoresed through 1.5% agarose gels.
Results
All seven SPF chickens showed no clinical signs. At necropsy, the cerebellar folia showed an irregular disorganized pattern in four and three chickens at 0 and 35 days of age, respectively ( and ). Both males and females were affected. The size of the affected cerebellums appeared normal. Common histopathologic findings in these cerebellums were solitary or multiple cerebellar cortical tissue islands from the deeper cortices to the medulla of the vermis (). These ectopic cortical tissues consisted of tangled islands of molecular and granular cell layers with haphazardly distributed Purkinje cells (), while the overlying cortical layers were normal. Small round to spindle-shaped cells, with round to oval nuclei and scant cytoplasm, resembling granular cells, also aggregated around small blood vessels (). Mitotic figures were occasionally observed in these granular cells. The thickness of the molecular and granular cell layers overlaying these ectopic tissues were slightly reduced with the external granular cell layers being one to two cells thick.
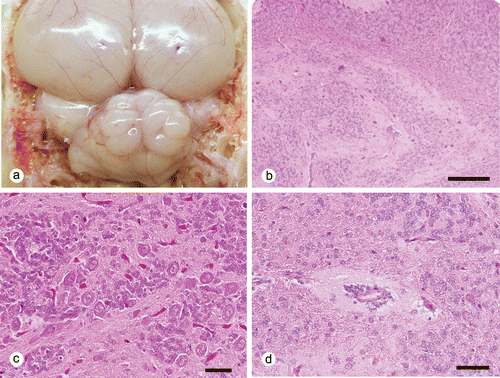
Table 1. Distribution and degree of cerebellar dysplasia in SPF chickens.
In sections stained by Bodian's method, heterotopic Purkinje cells intermingled with numerous disorganized axonal processes. By using immunofluorescent analysis for calbindin and vimentin, Purkinje cells in both the heterotopic islands and the normal cortex were strongly positive for the calbindin D28 protein. The heterotopic Purkinje cells randomly extended dendritic processes into the adjacent tissue and formed a molecular layer-like structure. A few disordered vimentin-positive Bergmann's fibres were present within the ectopic tissues, whereas the fibres radially extended towards the pial surface from the Golgi epithelial cells (Bergmann's glia) in the normal cortex (). The granular cells that aggregated around vessels were positive for proliferating cell nuclear antigen. All cells within the ectopic tissue were negative for glial fibrillary acid protein.
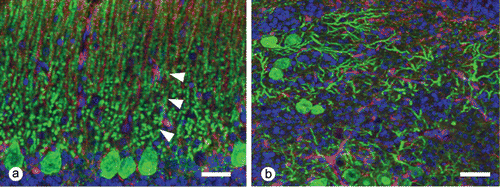
The cells in the affected brains were negative for ALVs, Marek's disease virus and chicken parvoviruses by PCR.
Discussion
All examined birds developed single to multiple foci of heterotopic cerebellar cortex from the deeper cortex to the medulla, mainly in the vermis. These heterotopic tissues consisted of disordered molecular, Purkinje cell and granular cell layer components.
The classification of cerebellar abnormalities appears to be confusing in veterinary medicine (Maxie & Youssef, Citation2007). In veterinary fields, cerebellar abnormalities are divided into two broad categories, namely hypoplasia and abiotrophy. In addition to these two categories, cerebellar dysplasia has been reported to be a minor entity and common in young animals rather than in adults. It is most commonly detected in the flocculonodular lobules in mammals. The foci are characterized histopathologically by tangled islands of germinal, molecular and granular cell layers together with haphazardly distributed Purkinje cells. Such dysplasia has been reported as part of the abnormalities of Dandy–Walker syndrome, Arnold–Chiari malformation, copper deficiency of sheep and some metabolic storage disorders. On the other hand, more detailed classifications for cerebellar dysplasia are proposed in human medicine, including cerebellar heterotopia as one type of isolated hemispheric dysplasia (Patel & Barkovich, Citation2002; Ellison et al., Citation2004). According to the classifications, cerebellar heterotopias fall into one of the dysplastic categories and are morphologically characterized by heterotopic grey matter within the cerebellar white matter. The foci are incidentally found in the hemispheres of infants, varying from a few cells to large islands of grey matter in which there are clusters of large cells surrounded by neuropil or islands of heterotopic cortex. On the basis of the classification of human medicine, the lesions of the current cases were consistent with cerebellar heterotopia because the cerebellar cortical tissue, with disordered layers, was distributed in the cerebellar medulla, resulting in disarrangement of the folia. In contrast to human cerebellar heterotopia, the ectopic islands in the current cases were mainly located in the vermis. This is considered to reflect the anatomical difference between the mammal and chicken cerebellum as the lateral lobes of the cerebellum in avian species are rudimentary and small in size. Although the thickness of the molecular and granular cell layers overlaying the ectopic tissues were slightly reduced, these foci seemed to be too small to cause cerebellar signs in the affected chickens.
The two most common causes of congenital cerebellar abnormalities in domestic animals are: hypoplasia and atrophy secondary to an in utero or perinatal viral infection; and a primary developmental defect malformation. The former occurs most commonly in cats and cattle (Maxie & Youssef, Citation2007), but has on rare occasions been reported in birds infected with avian parvovirus (Marusak et al., Citation2010), one strain of avian leukosis virus (Toyoda et al., Citation2006) and Aino virus (an arthropod-borne virus of Bunyaviruses) (Kitano et al., Citation1997). These abnormalities are characterized by a smaller cerebellum and histopathologically thinned granular cell layer and disorganized Purkinje cells with selective necrosis of the granular cells and gliosis. In the present cases, cerebellums had neither necrosis of the nervous tissues nor gliosis and all seven chickens were negative for chicken parvoviruses, ALVs and Aino viruses by PCR. These results suggest that the cerebellar dysplasia in this study is likely to have a genetic base. Unfortunately, follow-up studies were impossible as the commercial supplier of the SPF eggs changed the breeding lines.
There is only one report on cerebellar hypoplasia as a primary developmental defect malformation in avian species (Armién et al., Citation2013). In this report, the authors describe Purkinje cell heterotopia with cerebellar hypoplasia in two free-living American kestrels and that the anomaly seems to be genetically induced by molecular disruption of Purkinje cell migration, placement and maturation.
Development of the cerebellum is a complex event involving a number of cell types and this event is regulated by gradual gene expression (McGeady et al., Citation2006). Therefore, numerous gene abnormalities have been reported to contribute to the pathogenesis of cerebellar dysplasia in laboratory animals (Wang & Zoghbi, Citation2001). In the development process of the cerebellum in chick embryos, Purkinje cells and Bergmann's glia originate in the rhombic lip and migrate to form an irregular layer from the third to the sixth day of incubation (Nickel et al., Citation1973; Akar & Sur, Citation2010). At this stage, Purkinje cells move from the rhombic lip to their future position along radial fibres with the guidance of Reelin secreted from external granular cells. The external granular cell layer is formed between the sixth day and 15th day of incubation. The granular cells of the external granular cell layer migrate through the molecular layer to form the internal granular cell layer from the 18th day of incubation, peak at 2 days of age and are finally complete around 34 days of age. Bergmann's fibres act as guides for the migration of granular cells during this process. Genetic defects, including mutation within math1 and reln, have been reported to induce Purkinje cell heterotopy and disorganization of the cerebellar cortex architecture in mice (Heckroth et al., Citation1989; Jensen et al., Citation2002). Over 95% of Purkinje cells are ectopic in the reeler mutant mouse because of complete loss of the Reelin signal, whereas one population of Purkinje cells was located in an aberrant position in math1 null-mutant mouse due to the lack of an external granular cell layer. As well as Purkinje cell heterotopy, anomalies in the folia and fissures have been suggested to be associated with defects of genetic controls in the foliation process (Cerri et al., Citation2010). Bergmann's glial fibres direct migration of the granular cells at the base of the fissure, and the Purkinje cells regulate the number of folia by secreting sonic hedgehog, which influences granular cell proliferation in the external granular cell layer (Corrales et al., Citation2006; Sudarov & Joyner, Citation2007). It is suggested that the genetic defects change the relationship among glial fibre morphology, granular cell migration and Purkinje cell differentiation and impede the mechanical forces of foliation, resulting in alteration of cerebellar architecture (Cerri et al., Citation2010). The condition in our cases was probably due to defects of radial glia fibres and related migration factors. This is the first report on cerebellar dysplasia in White Leghorn chickens (Gallus gallus domesticus).
Acknowledgements
This research was supported by the Ministry of Education, Culture, Sports, Science and Technology of Japan under a Grant-in-Aid for Scientific Research (number B22380162) and a Grant-in-Aid for Challenging Exploratory Research (number 23658249); and a Research Fellowship of the Japan Society for the Promotion of Science for Young Scientists (grant number 2172).
References
- Akar, S. & Sur, E. (2010). The development of chicken cerebellar cortex and the determination of AgNOR activity of the Purkinje cell nuclei. Belgian Journal of Zoology, 140, 216–224.
- Armién, A.G., McRuer, D.L., Ruder, M.G. & Wünschmann, A. (2013). Purkinje cell heterotopy with cerebellar hypoplasia in two free-living American kestrels (Falco sparverius). Veterinary Pathology, 50, 182–187. 10.1177/0300985812442690
- Cerri, S., Piccolini, V.M. & Bernocchi, G. (2010). Postnatal development of the central nervous system: anomalies in the formation of cerebellum fissures. Anatomical Record, 293, 492–501. 10.1002/ar.21082
- Chang, K.S., Lee, S.I., Ohashi, K., Ibrahim, A. & Onuma, M. (2002). The detection of the meq gene in chicken infected with Marek's disease virus serotype 1. The Journal of Veterinary Medical Science, 64, 413–417. 10.1292/jvms.64.413
- Corrales, J.D., Blaess, S., Mahoney, E.M. & Joyner, A.L. (2006). The level of sonic hedgehog signaling regulates the complexity of cerebellar foliation. Development, 133, 1811–1821. 10.1242/dev.02351
- Ellison, D., Love, S., Chimelli, L., Harding, B.N., Lowe, J. & Vinters, H.V. (2004). Malformations. In D. Ellison & S. Love (Eds.). Neuropathology 2nd edn (pp. 95–99). Edinburgh: Mosby.
- Hatai, H., Ochiai, K., Tomioka, Y., Toyoda, T., Hayashi, K., Anada, M., Kato, M., Toda, A., Ohashi, K., Ono, E., Kimura, T. & Umemura, T. (2005). Nested polymerase chain reaction for detection of the avian leucosis virus causing so-called fowl glioma. Avian Pathology, 34, 473–479. 10.1080/03079450500368086
- Heckroth, J.A., Goldowitz, D. & Eisenman, L.M. (1989). Purkinje cell reduction in the reeler mutant mouse: a quantitative immunohistochemical study. Journal of Comparative Neurology, 279, 546–555. 10.1002/cne.902790404
- Jensen, P., Zoghbi, H.Y. & Goldowitz, D. (2002). Dissection of the cellular and molecular events that position cerebellar Purkinje cells: a study of the math1 null-mutant mouse. The Journal of Neuroscience, 22, 8110–8116.
- Kitano, Y., Yasuda, N., Shimizu, T., Ohzono, H. & Iwamoto, T. (1997). Teratogenicity of Aino virus in the chick embryo. Research in Veterinary Science, 62, 195–198. 10.1016/S0034-5288(97)90147-8
- Marusak, R.A., Guy, J.S., Abdul-Aziz, T.A., West, M.A., Fletcher, O.J., Day, J.M., Zsak, L. & Barnes, H.J. (2010). Parvovirus-associated cerebellar hypoplasia and hydrocephalus in day old broiler chickens. Avian Diseases, 54, 156–160. 10.1637/8976-070709-Case.1
- Maxie, M.G. & Youssef, S. (2007). Nervous system. In M.G. Maxie (Ed.). Jubb, Kennedy, and Palmer's Pathology of Domestic Animals 5th edn, vol. 1 (pp. 309–314). Philadelphia, PA: Saunders Elsevier.
- McGeady, T.A., Quinn, P.J. & FitzPatrick, E.S. (2006). Nervous system. In T.A. McGeady, P.J. Quinn & E.S. FitzPatrick (Eds.). Veterinary Embryology 1st edn (pp. 153–182). Oxford: Blackwell Publishing.
- Nickel, R., Schummer, A. & Seiferle, E. (1973). Central nervous system. In R. Nickel, A. Schummer & E. Seiferle (Eds.). Anatomy of the Domestic Birds (pp. 114–130). Berlin, Germany: Verlag Paul Parey.
- Patel, S. & Barkovich, A.J. (2002). Analysis and classification of cerebellar malformations. American Journal of Neuroradiology, 23, 1074–1087.
- Rice, D.S. & Curran, T. (1999). Mutant mice with scrambled brains: understanding the signaling pathways that control cell positioning in the CNS. Genes & Development, 13, 2758–2773. 10.1101/gad.13.21.2758
- Sudarov, A. & Joyner, A.L. (2007). Cerebellum morphogenesis: the foliation pattern is orchestrated by multi-cellular anchoring centers. Neural Development, 2, 26. 10.1186/1749-8104-2-26
- Tarasiuk, K., Wozniakowski, G. & Samorek-Salamonowicz, E. (2012). Occurrence of chicken parvovirus infection in Poland. The Open Virology Journal, 6, 7–11. 10.2174/1874357901206010007
- Toyoda, T., Ochiai, K., Hatai, H., Murakami, M., Ono, E., Kimura, T. & Umemura, T. (2006). Cerebellar hypoplasia associated with an avian leukosis virus inducing fowl glioma. Veterinary Pathology, 43, 294–301. 10.1354/vp.43-3-294
- Wang, V.Y. & Zoghbi, H.Y. (2001). Genetic regulation of cerebellar development. Nature Reviews Neuroscience, 2, 484–491. 10.1038/35081558
- Zsak, L., Strother, K.O. & Kisary, J. (2008). Partial genome sequence analysis of parvoviruses associated with enteric disease in poultry. Avian Pathology, 37, 435–441. 10.1080/03079450802210648