Abstract
We constructed a live, attenuated Salmonella Gallinarum (SG) that secretes heat-labile enterotoxin B subunit protein (LTB), and evaluated this strain as a new vaccine candidate by assessing its safety, immunogenicity and protective efficacy against fowl typhoid. An asd+ p15A ori low-copy plasmid containing eltB encoding LTB was transformed into a ΔlonΔcpxRΔasd SG (JOL967) to construct the candidate, JOL1355. In Experiments 1 and 2, birds were orally immunized with JOL1355 at 4 weeks of age, while control birds were inoculated with sterile phosphate-buffered saline. In Experiment 2, the birds of both groups were orally challenged with a virulent SG at 8 weeks of age. In Experiment 1, examination for safety revealed that the immunized group did not show any bacterial counts of the vaccine candidate in the liver and spleen. Birds immunized with the vaccine candidate showed a significant increase in systemic IgG and mucosal secretory IgA levels in Experiment 2. In addition, the lymphocyte proliferation response and the numbers of CD3+CD4+ and CD3+CD8+ T cells were also significantly elevated in the immunized group, which indicated that the candidate also induced cellular immune responses. In the protection assay, efficient protection with only 16% mortality in the immunized group was observed against challenge compared with 76% mortality in the control group. These results indicate that the live, attenuated SG secreting LTB can be a safe vaccine candidate. In addition, it can induce humoral and cellular immune responses and can efficiently reduce mortality of birds exposed to fowl typhoid.
Introduction
Fowl typhoid (FT) is an important systemic disease of poultry. FT is caused by the Gram-negative bacterium Salmonella enterica serovar Gallinarum (SG) (Shivaprasad, Citation2000). Globally, FT is characterized by highly acute mortality (60 to 70%) and severe inflammation including hepatitis, splenitis, typhlitis, and omphalitis, which results in significant economic losses to the poultry industry (Zhang-Barber et al., Citation1998; Wigley et al., Citation2005; Basnet et al., Citation2008). Extensive vaccination programmes, surveillance and biosecurity practices have contributed greatly to reducing the mortality caused by FT (Runnells et al., Citation1927; Berchieri et al., Citation1995; Barrow & Freitas-Neto, Citation2011). Among the potential vaccines, SG9R is an undefined mutant rough strain, which has been recommended to control FT disease (Lee et al., Citation2005). However, SG9R induced lesions of the liver and spleen due to residual virulence (Silva et al., Citation1981). In addition, SG9R did not protect efficiently against SG infection (Bouzoubaa et al., Citation1989).
Humoral and cellular immunity are required to prevent pathogenic Salmonella infections (Jones & Falkow, Citation1996). Strong secretory IgA (sIgA) immune response in the gut mucosa is essential to protect against infection with Salmonella (Roesler et al., Citation2006; Brumme et al., Citation2007). Cell-mediated immune mechanisms are essential to activate macrophages for clearance of intracellular Salmonella infection because salmonellas survive and replicate within macrophages (Mastroeni et al., Citation1993; Beal et al., Citation2006).
It is difficult to induce immune responses with orally inoculated antigens due to weak uptake of antigens from mucosal surfaces (Ogra et al., Citation2001). However, heat-labile enterotoxins of Escherichia coli have been extensively studied to stimulate immune responses (Hajishengallis et al., Citation2005). The E. coli heat-labile enterotoxin B subunit (LTB) binds to ganglioside receptors on the surface of eukaryotic cells, mainly GM1 (Lim et al., Citation2009). The stable cross-linking of GM1 at cell surfaces has been shown to increase the uptake of co-administered proteins (Freytag & Clements, Citation2005), with the resultant enhancement of mucosal and cellular immunity (Fingerut et al., Citation2006). Furthermore, oral vaccines offer an inexpensive, easily accessible and needle-free delivery mode of immunization, thus making them preferable for mass immunization during pandemics (Holmgren et al., Citation2003). In a previous study, a live SG candidate secreting LTB, JOL1229, was used as an adjuvant for a live SG vaccine candidate, JOL916 (Jeon et al., Citation2012). However, a significant drawback in this preparation was that the two different live SG strains had to be properly diluted to prepare the proper vaccine mixture before being inoculated into birds, a process that was inconvenient, uneconomical and laborious. In addition, safety is also a concern in the case of the combined JOL916 and JOL1229 vaccine candidate, which showed bacterial recovery in the liver and spleen after immunization (Jeon et al., Citation2012).
In this study, a live Salmonella Gallinarum secreting LTB, JOL1355, was constructed by transforming a low-copy-number plasmid (p15A ori) with eltB encoding LTB into a Salmonella Gallinarum delivery strain (▵lon▵cpxR▵asd) (). The efficacy of Salmonella Gallinarum secreting LTB as a vaccine candidate was evaluated for efficient protection against FT in chickens.
Table 1. Bacterial strains and plasmids used in this study.
Materials and Methods
Chickens
One-day-old female Lohmann-Brown chickens were used in this study. Water and food were provided ad libitum. All bird experiments were conducted under ethics approval (CBU 2011–0017) from the Chonbuk National University Animal Ethics Committee in accordance with the guidelines of the Korean Council on Animal Care.
Bacterial strains, plasmids and genetic manipulation
The chromosomal asd mutation of the SG delivery strain JOL967 (▵lon▵cpxR▵asd) was complemented in a balanced, lethal host-vector system by a low-copy Asd+ vector (p15A ori) containing the asd gene (). The asd gene sequence of Salmonella Typhimurium (accession number AF015781) was amplified by polymerase chain reaction (PCR) using asd primers (). The amplified DNA fragment of the asd gene was used to construct an Asd+ vector (p15A ori). This recombinant plasmid was further enhanced by adding a DNA fragment specifying the signal sequence of β-lactamase, which is efficiently transported into the periplasmic space in Salmonella. The β-lactamase gene from the pBR322 DNA template was amplified by PCR. This PCR product was then cloned into the Asd+ vector, resulting in the plasmid, pJHL80 (). Transcription promoted by Ptrc can be stopped by the 5ST1T2 transcriptional terminator that is located after the multicloning sites. The eltB gene encoding LTB was amplified from genomic DNA of the E. coli strain, JOL500, by PCR using the eltB primers (). The PCR product of eltB gene was introduced into pJHL80 to generate pJHL80-LTB. Subsequently, the plasmid pJHL80-LTB was introduced into JOL967 by electroporation. This final strain was designated JOL1355, and was used as the live LTB vaccine candidate of SG ().
Table 2. Primers used in this study.
Western blot assay
To observe LTB protein secretion from JOL1355, western blot assay was performed. The samples were prepared by culturing the vaccine JOL1355 (pJHL80-LTB) and negative control JOL967-pJHL80 strains in Luria–Bertani broth (Becton, Dickinson and Company, Sparks, MD, USA) at 37°C (). After centrifugation at 3400 × g for 20 min, the supernatants of both cultures were passed individually through a 0.22-µm pore-size filter and precipitated overnight with 20% trichloroacetic acid (v/v) to isolate the secreted form of LTB. JOL1355 and JOL967-pJHL80 protein samples were boiled at 96°C for 5 min, and were separated by 15% sodium dodecyl sulphate-polyacrylamide gel electrophoresis. Separated proteins were transferred electrophoretically onto polyvinylidene fluoride membranes (Millipore, Billerica, MA, USA) for the immunoblotting procedure as reported previously (Hur & Lee, Citation2011).
Preparation of LTB and virulent challenge strains
The JOL1355 and a virulent wild-type strain, JOL422, were grown in Luria–Bertani broth (Becton, Dickinson and Company) at 37°C to an optical density at 600 nm of 0.6. After centrifugation (16,100 × g for 5 min) of harvested cells, the pellets were re-suspended in sterile phosphate-buffered saline (PBS) to adjust to appropriate concentrations for inoculations.
Bacterial inoculation in chickens
In Experiment 1, chickens (n = 40) were equally distributed into two groups. The first group of chickens was orally inoculated with 100 µl sterile PBS as a control (n = 20). The remaining chickens were orally immunized with 100 µl total bacterial suspension containing 1 × 108 colony-forming units (CFU) of the live attenuated SG secreting LTB (JOL1355) at 4 weeks of age (n = 20).
In Experiment 2, chickens (n = 50) were divided into two groups. The first group of chickens was inoculated with 100 µl sterile PBS as a control (n = 25). The remaining chickens were orally immunized at 4 weeks with 100 µl total bacterial suspension containing 1 × 108 CFU of vaccine candidate (JOL1355) (n = 25). The wild-type SG isolate (JOL422) was used as the virulent challenge strain. All chickens were orally challenged with 100 µl bacterial suspension containing 1 × 106 CFU of the strain at the fourth week post immunization. Food and water were withdrawn prior to bacterial inoculation and were re-supplied 2 h after immunization.
Examination of the safety of the LTB-secreting strain JOL1355
During 4 weeks post immunization, birds were monitored daily for anorexia, depression and diarrhoea, which may be caused by administration of live vaccine strain JOL1355 in Experiment 1 (Matsuda et al., Citation2011). In addition, the presence of the LTB strain (JOL1355) in the liver and spleen from five birds of each group was examined weekly for 4 weeks post immunization. The presence of LTB strain was confirmed by PCR using OMPC primers for the Salmonella genus, SG primers for Salmonella Gallinarum, lon and cpxR primers for the vaccine strain, and LTB primers for the LTB adjuvant strain (Alvarez et al., Citation2004; Kang et al., Citation2011; Jeon et al., Citation2012). The gross lesions and bacterial recovery from the liver and spleen samples were examined as described previously (Matsuda et al., Citation2011).
Systemic (IgG) and mucosal (sIgA) immune responses by enzyme-linked immunosorbent assay
To evaluate anti-Salmonella Gallinarum antibodies, blood samples and intestinal lavage samples were collected from five birds of each group after immunization in Experiment 2. For determination of systemic IgG concentrations, plasma samples were separated by centrifugation of peripheral blood. The lavage technique was used to collect intestinal lavage samples for determination of sIgA concentrations (Porter & Holt, Citation1992). The intestine lavage samples were stored at 4°C until use. Indirect enzyme-linked immunosorbent assay (ELISA) was performed with the outer membrane protein fraction (OMP) extracted from a wild-type SG, JOL394. ELISA was performed using chicken IgG and IgA ELISA Quantitation Kits (Bethyl Laboratories, Texas, USA) for determination of IgG and IgA concentrations as reported previously (Matsuda et al., Citation2010, Citation2011).
In addition, plasma IgG and intestinal sIgA antibodies against LTB antigen were also evaluated. Briefly, Microlon® ELISA plate wells (Greiner, Bio-One GmbH, Frickenhausen, Germany.) were coated with LTB antigen at a concentration of 3.4 µg/ml. The LTB antigen was prepared from the E. coli wild-type strain JOL500 as described previously (Hur & Lee, Citation2011). After the 1:100 dilution of plasma and 1:4 dilution of intestinal wash samples, these samples were added to the LTB-coated plates and incubated for 1 h, followed by reaction with goat anti-chicken IgG and IgA horseradish peroxidase conjugates for 1 h. The bound horseradish peroxidase activity was determined using o-phenylenediamine dihydrochloride (Sigma-Aldrich, St. Louis, MO, USA.). The optical density at 492 nm was determined with an ELISA reader after the reaction was stopped with 3 M H2SO4. The mean concentration of antibody titres was measured by plotting the standard curve for each IgG and IgA antibody as reported previously (Hur & Lee, Citation2011).
Peripheral lymphocyte proliferation assay
In Experiment 2, the peripheral lymphocytes were separated from five randomly selected chickens per group using the gentle swirl technique at the fourth week post immunization to examine cell-mediated immunity. After trypan blue dye exclusion testing, 100 µl viable mononuclear cell suspensions at 1 × 105 CFU/ml in RPMI-1640 medium were incubated in triplicate in 96-well tissue culture plates with 50 µl medium alone or medium containing 4 µg/ml soluble antigen at 40°C, in a humidified 5% carbon dioxide atmosphere for 72 h. Soluble antigen was prepared from the SG wild-type strain JOL394 as described previously with a little modification (Rana & Kulshreshtha, Citation2006). The proliferation of stimulated lymphocytes was measured using adenosine triphosphate bioluminescence as a marker of cell viability with the ViaLight® Plus Kit (Lonza, Rockland, ME, USA). The emitted light intensity was measured using a luminometer (TriStarLB941; Berthold Technologies GmbH & Company, Bad Wildbad, Germany). The blastogenic response against specific antigen was expressed as the mean stimulation index as reported previously (Rana & Kulshreshtha, Citation2006).
Flow cytometry for CD3+CD4+ and CD3+CD8+ T cells
To examine the effective induction of T cells upon immunization, blood samples were collected from three birds of each group at the seventh day after immunization in Experiment 2. These blood samples were mixed with 3% hetastarch (Sigma Immuno Chemicals, St Louis, MO, USA) at a ratio of 1:2 and centrifuged at 65 × g for 10 min to allow erythrocytes to sediment. The supernatant cells (leukocytes) were adjusted to a concentration of 1 × 106 cells/ml in PBS. Then 100 µl of 1 × 106 isolated leukocytes were incubated with the fluorescein isothiocyanate-labelled anti-CD3, biotin-labelled anti-CD4 and phycoerythrin-labelled anti-CD8a (Southern Biotech, Birmingham, AL, USA) monoclonal antibodies in the dark at 4°C for 30 min. After washing with PBS, the cells were re-suspended in 100 µl PBS and again incubated with allophycocyanin-labelled streptavidin (Southern Biotech) in the dark at 4°C for 30 min. After washing, all samples were analysed using a FACS Calibur (BD Bioscience, Heidelberg, Germany).
Challenge experiment
The wild-type SG isolate, JOL422, was used as the virulent challenge strain, and was orally inoculated into each bird with 100 µl bacterial suspension containing 1 × 106 CFU at the fourth week post immunization in Experiment 2. All chickens were examined for general conditions and mortality for 14 days after the virulent challenge strain inoculation.
Statistical analysis
The analyses of all data were performed by the SPSS 16.0 program (SPSS Inc., Chicago, IL, USA.). Results are expressed as mean ± standard deviation values. The Mann–Whitney U test was used to show the significant differences in the immune responses between the immunized and control birds. In addition, mortality of birds was analysed by chi-square test between the immunized and control groups after virulent SG challenge.
Results
LTB secretion from JOL1355
The 327-base-pair DNA fragment of eltB gene from the LT+ E. coli JOL500 was amplified using the eltB-specific primers () and cloned into low-copy plasmid pJHL80. Furthermore, pJHL80 carrying eltB (pJHL80-LTB) was introduced into a ΔlonΔcpxRΔasd Salmonella Gallinarum strain (JOL967) and designated as the LTB vaccine candidate JOL1355. To examine the secretion of LTB protein from JOL1355, immunoblot analysis was performed. An SG harbouring pJHL80 plasmid only, JOL967, was used as the negative control. After analysis by immunoblot assay, an 11.6-kDa band (the monomer size of the LTB protein) was evident in the precipitated culture supernatant (, lane 2). However, no band was observed in the negative control sample (, lane 1).
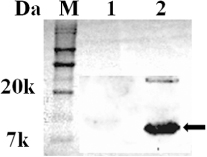
Examination of the safety of the LTB-secreting strain JOL1355
The live LTB vaccine candidate JOL1355 did not cause any adverse effects on the general condition of the birds in Experiment 1. In addition, the gross lesions were not observed, while the LTB vaccine strain was not isolated from the liver and spleen of the immunized and control birds at the first, second, third and fourth week post-immunization.
Systemic and mucosal immune responses in chickens induced by immunization
Systemic IgG and mucosal sIgA antibody titres against SG specific antigen and LTB were evaluated each week after immunization in the immunized and control groups in Experiment 2. The plasma IgG and intestinal sIgA levels of the immunized group remained significantly elevated compared with those of the control group at the second, third and fourth week post immunization (; P ≤ 0.05). The plasma IgG level of the immunized group was significantly increased by approximately 2.2 times compared with that of the control group at the third week post immunization. In addition, the intestinal sIgA level of the immunized group was also significantly augmented by approximately 2.3 times compared with that of the control group at the third week post immunization (; P ≤ 0.05).
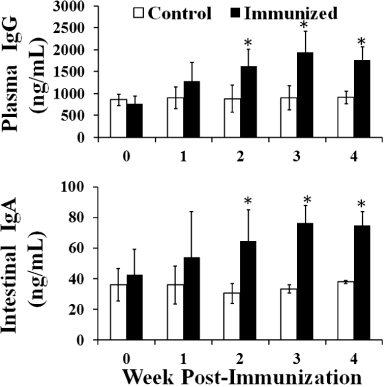
Anti-LTB plasma IgG and intestinal sIgA levels of the immunized group were significantly higher than the control group. The plasma IgG levels were significantly higher in the immunized group at the second, third and fourth week post immunization, while the intestinal sIgA levels were significantly elevated in the immunized group at the first, second, third and fourth week post immunization (; P ≤ 0.05). The plasma IgG level of the immunized group was significantly augmented by approximately 3.1 times compared with that of the control group at the third week post immunization, while the intestinal sIgA level of the immunized group was significantly increased by approximately 2.0 times compared with that of the control group at the second and third week post immunization (; P ≤ 0.05).
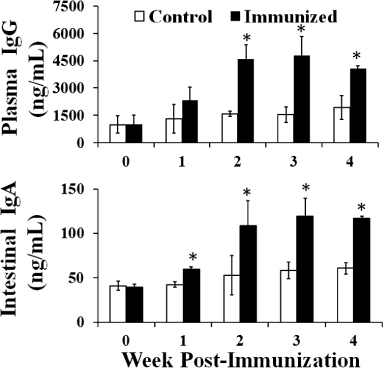
Cellular immune responses in chickens induced by immunization
In Experiment 2, the patterns of cellular immune responses in the immunized and control groups were investigated by the peripheral lymphocyte cell proliferation assay using the soluble antigen of SG. The lymphocytic proliferative responses are presented in . After immunization, the mean stimulation index value of the immunized group was 2.3 times higher than the control group at the fourth week post immunization (; P ≤ 0.05).
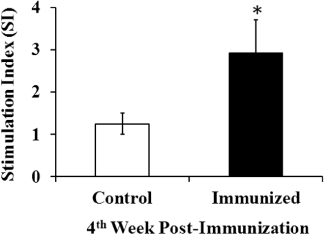
To quantify changes in T-cell composition of the control and immunized groups, the occurrence of CD3+CD4+ and CD3+CD8+ T cells in peripheral blood was also analysed by flow cytometry. At the seventh day post immunization, the CD3+CD4+ T-cell population in the immunized group was significantly elevated to 31.8 ± 9.1 compared with 15.5 ± 6.1 for the control group (; P ≤ 0.05). In addition, the CD3+CD8+ T-cell population of the immunized group was significantly increased to 14.0 ± 1.5 compared with 7.7 ± 3.8 for the control group (; P ≤ 0.05).
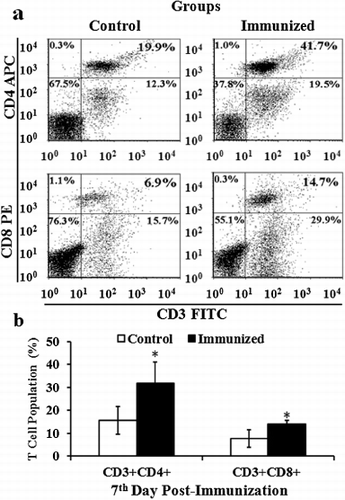
Protective efficacy against virulent strain challenge
To evaluate whether the LTB strain had protective efficacy, the general condition and mortality rates were assessed in the control and immunized groups 14 days after challenge in Experiment 2. In the immunized group, a small number of birds showed slight and temporary depression followed by recovery, while in the unimmunized control group, all birds showed signs of depression after virulent SG challenge. As demonstrated in , the immunized group showed a significantly higher survival rate than that of the control group. During 14 days post challenge, 21 of 25 birds survived in the immunized group, while only six of 25 survived in the control group. This result indicates that the immunized group showed a 16% mortality rate compared with the 76% mortality in the control group (P ≤ 0.05).
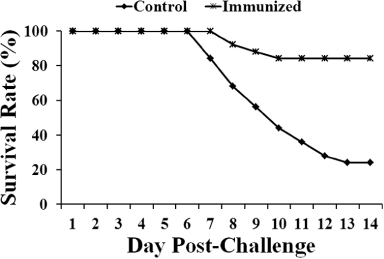
Discussion
SG is an intracellular pathogen that invades the gastrointestinal mucosa and establishes systemic infection by residing in macrophages (Barrow et al., Citation1994;). Unlimited proliferation of fully virulent strains of SG in the host leads to bacteraemia, acute illness and death (Christensen et al., Citation1996). The efficient systemic immune responses including both cell-mediated and humoral immune responses are considered to be essential for protection against Salmonella (Wigley et al., Citation2002). Mucosal immunity is also important in protecting mucosal surfaces against invasion and colonization by microbes (Holmgren & Czerkinsky, Citation2005). Orally administered vaccines can enhance mucosal and systemic immunity (Freytag & Clements, Citation2005). However, most orally administered antigens are poor immunogens due to immunological tolerance or unresponsiveness as a result of induced tolerance (Yuki & Kiyono, Citation2003).
LTB is capable of modulating immune responses (Fischer et al., Citation2010). In addition, LTB acts as an efficient inducer without danger of toxicity after administration (Fingerut et al., Citation2005). In our previous studies, an LTB-secreting live SG strain, JOL1229, was used as an adjuvant for the SG vaccine candidate, JOL916, by mixture of both strains (Jeon et al., Citation2012). However, the high-copy-number plasmid pBR ori was used for the construction of JOL1229 (Kang et al., Citation2002, Jeon et al., Citation2012). In addition, it was essential to mix JOL916 and JOL1229 at an appropriate dilution before inoculation into birds (Jeon et al., Citation2012). Thus, the use of this LTB adjuvant strain strategy is limited due to the inconvenience of optimizing the concentrations of vaccine candidate and LTB adjuvant strains. In addition, the SG vaccine–adjuvant mixture approach was not shown to be completely safe because bacteria from the vaccine were recovered in the liver and spleen after immunization in birds. To overcome this problem, SG harbouring the eltB gene expressing the LTB protein carried by the recombinant p15A ori plasmid was constructed to obtain a vaccine candidate that did not require mixture of any additional strain. The low-copy-number plasmid p15A ori maintains 10 to 12 copy numbers per cell (Sambrook et al., Citation1989). This SG vaccine candidate can secrete LTB protein, which is highly immunogenic and/or more readily interacts with antigen-presenting cells (Kang et al., Citation2002). In addition, a recombinant p15A ori plasmid was also designated as pJHL80, which possesses the asd gene and β-lactamase signal sequence (bla SS). The fusion of a protein to the bla SS promotes the secretion of the fusion protein into the periplasm of Gram-negative bacteria, including Salmonella (Summers & Knowles, Citation1989; Pawelek et al., Citation1997; Kang et al., Citation2002). The ΔlonΔcpxRΔasd SG strain carrying the eltB gene on the plasmid pJHL80-LTB with the bla SS (JOL1355) can therefore efficiently express and secrete LTB protein ().
SG invades the macrophages of the liver and spleen (Barrow et al., Citation1994). The high numbers of live vaccine strains in the internal organs may cause significant lesions, functional organ impairment and appearance of clinical signs (Christensen et al., Citation1996). However, gross lesions and bacterial recovery were not observed in the JOL1355 immunized birds. Subsequently, no clinical signs such as anorexia, depression and diarrhoea were observed in the birds immunized with the vaccine candidate. These results suggest that the live LTB vaccine candidate JOL1355 can be safe in the birds after immunization.
Oral immunization can be an effective means of inducing mucosal, systemic and cell-mediated immune responses (Freytag & Clements, Citation2005). The success of immunization against intracellular pathogens such as Salmonella Gallinarum is largely attributed to the enhancement of systemic and mucosal immune responses (Titball, Citation2008). In this study, systemic IgG and mucosal sIgA immune responses were significantly enhanced against SG-OMP-specific and LTB-specific antigens by immunization with the vaccine candidate. Systemic antibodies are important to target Salmonella, which escape from infected cells to establish new infection foci at distant tissue sites (Dougan et al., Citation2011). In addition, secretory antibodies, such as sIgA, may block the colonization of pathogens in the mucosal epithelium. They can also inhibit the attachment of microbial toxins on epithelial cells (Pavot et al., Citation2012).
Furthermore, cell-mediated immune responses are important in the late stages of a primary Salmonella infection (Barrow, Citation2007; Hur & Lee, Citation2010). Immunization with live vaccines is considered to confer better protection against intracellular pathogens due to their ability to enhance not only humoral immune responses, but also cell-mediated immune responses (Lindberg & Robertsson, Citation1983; Chong et al., Citation1996; Mastroeni et al., Citation2001). In this study, the lymphocyte proliferation assay using adenosine triphosphate bioluminescence as a marker of cell viability showed significant response at the fourth week post immunization in the vaccinated group, suggesting that this vaccine candidate could induce cellular immune responses. In addition, significantly increased CD3+CD4+ and CD3+CD8+ T-cell populations were observed by the flow cytometric analysis in the immunized group as compared with the control group. In the previous study, oral administration of LTB stimulated CD4+ regulatory T cells (Turcanu et al., Citation2002). To kill intracellular pathogens, macrophage and immune responsiveness can be activated by CD4+ T cells, which produce a range of cytokines (Seder & Hill, Citation2000). In addition, CD8+ cytotoxic T cells can kill Salmonella-infected cells (Bleackley, Citation2008). The humoral and cellular immune responses induced in the immunized group are indicative of efficiently developed acquired immunity in the immunized chickens.
In addition, all chickens of the control group showed detectable signs of depression in their general condition after virulent challenge with a wild-type SG strain. However, the vaccinated chickens showed no or very few clinical signs of depression. In the protection assay, the mortality rate in the immunized group was significantly lower than that of the control group. Survival rates in the immunized group were considerably higher than those of the control group chickens from the seventh day post challenge to the 14th day post challenge (). Immunization with JOL1355 offered efficient protection with only 16% mortality following wild-type challenge, while 76% mortality was observed in the control group (P ≤ 0.05), indicating that JOL1355 is a potential live LTB vaccine candidate.
In conclusion, the present data demonstrated that oral administration with only the LTB vaccine candidate, JOL1355, can be safe; and can efficiently induce acquired immunity including mucosal sIgA, systemic IgG, and cellular immune responses, such as lymphocyte proliferative response and CD3+CD4+ and CD3+CD8+ T cells. To protect against experimental infection of FT, protective immune responses can be induced by the LTB vaccine candidate of SG, which can be used alone without addition of any other strains. Furthermore, immunization with the vaccine candidate, JOL1355, may be a more convenient strategy than the previous one that relied on finding the optimal mixture of vaccine–adjuvant.
Acknowledgements
This work was supported by a National Research Foundation of Korea grant funded by the Korea Government (MISP; No. 2013R1A4A1069486).
References
- Alvarez, J., Sota, M., Vivanco, A.B., Perales, I., Cisterna, R., Rementeria, A. & Garaizar, J. (2004). Development of a multiplex PCR technique for detection and epidemiological typing of Salmonella in human clinical samples. Journal of Clinical Microbiology, 42, 1734–1738. 10.1128/JCM.42.4.1734-1738.2004
- Barrow, P.A. (2007). Salmonella infections: immune and non-immune protection with vaccines. Avian Pathology, 36, 1–13. 10.1080/03079450601113167
- Barrow, P.A. & Freitas-Neto, O.C. (2011). Pullorum disease and fowl typhoid – new thoughts on old diseases: a review. Avian Pathology, 40, 1–13. 10.1080/03079457.2010.542575
- Barrow, P.A., Huggins, M.B. & Lovell, M.A. (1994). Host specificity of Salmonella infection in chickens and mice is expressed in vivo primarily at the level of the reticulo-endothelial system. Infection and Immunity, 62, 4602–4610.
- Basnet, H.B., Kwon, H.J., Cho, S.H., Kim, S.J., Yoo, H.S., Park, Y.H., Yoon, S.I., Shin, N.S. & Youn, H.J. (2008). Reproduction of fowl typhoid by respiratory challenge with Salmonella Gallinarum. Avian Diseases, 52, 156–159. 10.1637/7974-032607-Reg
- Beal, R.K., Powers, C., Davison, T.F., Barrow, P.A. & Smith, A.L. (2006). Clearance of enteric Salmonella enterica serovar Typhimurium in chickens is independent of B-cell function. Infection and Immunity, 74, 1442–1444. 10.1128/IAI.74.2.1442-1444.2006
- Berchieri, Jr. A., Iba, A.M. & Barrow, P.A. (1995). Examination by ELISA of sera obtained from chicken breeder and layer flocks showing evidence of fowl typhoid or pullorum disease. Avian Pathology, 24, 411–420. 10.1080/03079459508419081
- Bleackley, R.C. (2008). Cytotoxic T lymphocytes. In W.E. Paul (Ed.). Fundamental Immunology 6th edn (pp. 1079–1095). Philadelphia, PA: Lippincott Williams and Wikins.
- Bolivar, F., Rodriguez, R.L., Greene, P.J., Betlach, M.C., Heyneker, H.L., Boyer H.W., Crosa, J.H. & Falkow, S. (1977). Construction and characterization of new cloning vehicles. II. A multipurpose cloning system. Gene, 2, 95–113. 10.1016/0378-1119(77)90000-2
- Bouzoubaa, K., Nagaraja, K.V., Kabbaj, F.Z., Newman, J.A. & Pomeroy, B.S. (1989). Feasibility of using proteins from Salmonella Gallinarum vs. 9R live vaccine for the prevention of fowl typhoid in chickens. Avian Diseases, 33, 385–391. 10.2307/1591094
- Brumme, S., Arnold, T., Sigmarsson, H., Lehmann, J., Scholz, H.C., Hardt, W.D., Hensel, A., Truyen, U. & Roesler, U. (2007). Impact of Salmonella Typhimurium DT104 virulence factors invC and sseD on the onset, clinical course, colonization patterns and immune response of porcine salmonellosis. Veterinary Microbiology, 124, 274–285. 10.1016/j.vetmic.2007.04.032
- Chong, C., Bost, K.L. & Clements, J.D. (1996). Differential production of interleukin-12 mRNA by murine macrophages in response to viable or killed Salmonella spp. Infection and Immunity, 64, 1154–1160.
- Christensen, J.P., Barrow, P.A., Olsen, J.E., Poulsen, J.S. & Bisgaard, M. (1996). Correlation between viable counts of Salmonella Gallinarum in spleen and liver and the development of anaemia in chickens as seen in experimental fowl typhoid. Avian Pathology, 25, 769–783. 10.1080/03079459608419180
- Dougan, G., John, V., Palmer, S. & Mastroeni, P. (2011). Immunity to salmonellosis. Immunological Reviews, 240, 196–210. 10.1111/j.1600-065X.2010.00999.x
- Fingerut, E., Gutter, B., Meir, R., Eliahoo, D. & Pitcovski, J. (2005). Vaccine and adjuvant activity of recombinant subunit B of E. coli enterotoxin produced in yeast. Vaccine, 23, 4685–4696. 10.1016/j.vaccine.2005.03.050
- Fischer, G., Conceicao, F.R., Leite, F.P.L., Moraes, C.M., Ferreira, L.N., Vilela, C.O., Caetano, C.F., Vargas, G.D., Hubner, S.O., Vidor, T. & Roehe, P.M. (2010). Recombinant Escherichia coli heat-labile enterotoxin B subunit humoral adjuvant effect depends on dose and administration route. World Journal of Microbiology and Biotechnology, 26, 489–495. 10.1007/s11274-009-0195-z
- Fingerut, E., Gutter, B., Goldway, M., Eliahoo, D. & Pitcovski, J. (2006). B subunit of E. coli enterotoxin as adjuvant and carrier in oral and skin vaccination. Veterinary Immunology and Immunopathology, 112, 253–263. 10.1016/j.vetimm.2006.03.005
- Freytag, L.C. & Clements, J.D. (2005). Mucosal adjuvants. Vaccine, 23, 1804–1813. 10.1016/j.vaccine.2004.11.010
- Hajishengallis, G., Arce, S., Gockel, C.M., Connell, T.D. & Russell, M.W. (2005). Immunomodulation with enterotoxins for the generation of secretory immunity or tolerance: application for oral infections. Journal of Dental Research, 84, 1104–1116. 10.1177/154405910508401205
- Holmgren, J. & Czerkinsky, C. (2005). Mucosal immunity and vaccines. Nature Medicine, 11, S45–S53. 10.1038/nm1213
- Holmgren, J., Czerkinsky, C., Eriksson, K. & Mharandi, A. (2003). Mucosal immunisation and adjuvants: a brief overview of recent advances and challenges. Vaccine, 21, S89–S95. 10.1016/S0264-410X(03)00206-8
- Hur, J. & Lee, J.H. (2010). Immunization of pregnant sows with a novel virulence gene deleted live Salmonella vaccine and protection of their suckling piglets against salmonellosis. Veterinary Microbiology, 143, 270–276. 10.1016/j.vetmic.2009.11.034
- Hur, J. & Lee, J.H. (2011). Enhancement of immune responses by an attenuated Salmonella enterica serovar Typhimurium strain secreting an Escherichia coli heat-labile enterotoxin B subunit protein as an adjuvant for a live Salmonella vaccine candidate. Clinical Vaccine Immunology, 18, 203–209. 10.1128/CVI.00407-10
- Jeon, B.W., Jawale, C.V., Kim, S.H. & Lee, J.H. (2012). Attenuated Salmonella Gallinarum secreting an Escherichia coli heat-labile enterotoxin B subunit protein as an adjuvant for oral vaccination against fowl typhoid. Veterinary Immunology and Immunopathology, 150, 149–160. 10.1016/j.vetimm.2012.09.006
- Jones, B.D. & Falkow, S. (1996). Salmonellosis: host immune responses and bacterial virulence determinants. Annual Review of Immunology, 14, 533–561. 10.1146/annurev.immunol.14.1.533
- Kang, H.Y., Srinivasan, J. & Curtiss, R. (2002). Immune responses to recombinant pneumococcal PspA antigen delivered by live attenuated Salmonella enterica serovar Typhimurium vaccine. Infection and Immunity, 70, 1739–1749. 10.1128/IAI.70.4.1739-1749.2002
- Kang, M.S., Kwon, Y.K., Jung, B.Y., Kim, A., Lee, K.M., An, B.K., Song, E.A., Kwon, J.H. & Chung, G.S. (2011). Differential identification of Salmonella enterica subsp. enterica serovar Gallinarum biovars Gallinarum and Pullorum based on polymorphic regions of glgC and speC genes. Veterinary Microbiology, 147,181–185. 10.1016/j.vetmic.2010.05.039
- Lee, Y.J., Mo, I.P. & Kang, M.S. (2005). Safety and efficacy of Salmonella Gallinarum 9R vaccine in young laying chickens. Avian Pathology, 34, 362–366. 10.1080/03079450500180895
- Lim, J., Kim, J., Chung, H., Kim, T., Kim, J., Lee, K., Park, S., Yang, M. & Kim, D. (2009). Expression of functional pentameric heat-labile enterotoxin B subunit of Escherichia coli in Saccharomyces cerevisiae. Journal of Microbiology and Biotechnology, 19, 502–510. 10.4014/jmb.0803.207
- Lindberg, A.A. & Robertsson, J.A. (1983). Salmonella Typhimurium infection in calves: cell-mediated and humoral immune reactions before and after challenge with live virulent bacteria in calves given live or inactivated vaccines. Infection and Immunity, 41, 751–757.
- Mastroeni, P., Chabalgoity, J.A., Dunstan, S.J., Maskell, D.J. & Dougan, G. (2001). Salmonella: immune responses and vaccines. The Veterinary Journal, 161, 132–164. 10.1053/tvjl.2000.0502
- Mastroeni, P., Villarreal-Ramos, B. & Hormaeche, C.E. (1993). Adoptive transfer of immunity to oral challenge with virulent Salmonella in innately susceptible BALB/c mice requires both immune serum and T cells. Infection and Immunity, 61, 3981–3984.
- Matsuda, K., Chaudhari, A.A., Kim, S.W., Lee, K.M. & Lee, J.H. (2010). Physiology, pathogenicity and immunogenicity of lon and/or cpxR deleted mutants of Salmonella Gallinarum as vaccine candidates for fowl typhoid. Veterinary Research, 41, 59. 10.1051/vetres/2010031
- Matsuda, K., Chaudhari, A.A. & Lee, J.H. (2011). Evaluation of safety and protection efficacy on cpxR and lon deleted mutant of Salmonella Gallinarum as a live vaccine candidate for fowl typhoid. Vaccine, 29, 668–674. 10.1016/j.vaccine.2010.11.039
- Ogra, P.L., Faden, H. & Welliver, R.C. (2001). Vaccination strategies for mucosal immune responses. Clinical Microbiological Reviews, 14, 430–445. 10.1128/CMR.14.2.430-445.2001
- Pavot, V., Rochereau, N., Genin, C., Verrier, B. & Paul, S. (2012). New insights in mucosal vaccine development. Vaccine, 30, 142–154. 10.1016/j.vaccine.2011.11.003
- Pawelek, J.M., Low, K.B. & Bermudes, D. (1997). Tumor-targeted Salmonella as a novel anticancer vector. Cancer Research, 57, 4537–4544.
- Porter, Jr. R.E. & Holt, P.S. (1992). Use of a pilocarpine-based lavage procedure to study secretory immunoglobulin concentration in the alimentary tract of White Leghorn chickens. Avian Diseases, 36, 529–536. 10.2307/1591745
- Rana, N. & Kulshreshtha, R.C. (2006). Cell-mediated and humoral immune responses to a virulent plasmid-cured mutant strain of Salmonella enterica serotype Gallinarum in broiler chickens. Veterinary Microbiology, 115, 156–162. 10.1016/j.vetmic.2006.01.011
- Roesler, U., Heller, P., Waldmann, K.H., Truyen, U. & Hensel, A. (2006). Immunization of sows in an integrated pig-breeding herd using a homologous inactivated Salmonella vaccine decreases the prevalence of Salmonella Typhimurium infection in the offspring. Journal of Veterinary Medicine, 53, 224–228. 10.1111/j.1439-0450.2006.00951.x
- Runnells, R.A., Coon, C.J., Farley, H. & Thorp, F. (1927). An application of the rapid method agglutination test to the diagnosis of bacillary white diarrhoea infection. Journal of the American Veterinary Medical Association, 70, 660–667.
- Sambrook, J., Fritsch, E.F. & Maniatis, T. (1989). Molecular Cloning, a Laboratory Manual 2nd edn. New York: Cold Spring Harbor Laboratory.
- Seder, R.A. & Hill, A.V.S. (2000). Vaccines against intracellular infections requiring cellular immunity. Nature, 406, 793–798. 10.1038/35021239
- Shivaprasad, H.L. (2000). Fowl typhoid and pullorum disease. Revue scientifique et technique (International Office of Epizootics), 19, 405–424.
- Silva, E.N., Snoeyenbos, G.H., Weinack, O.M. & Smyser, C.F. (1981). Studies on the use of 9R strain of Salmonella Gallinarum as a vaccine in chickens. Avian Diseases, 25, 38–52. 10.2307/1589825
- Summers, R.G. & Knowles, J.R. (1989). Illicit secretion of a cytoplasmic protein into the periplasm of Escherichia coli requires a signal peptide plus a portion of the cognate secreted protein. The Journal of Biological Chemistry, 264, 20074–20081.
- Titball, R.W. (2008). Vaccines against intracellular bacterial pathogens. Drug Discovery Today, 13, 596–600. 10.1016/j.drudis.2008.04.010
- Turcanu, V., Hirst, T.R. & Williams, N.A. (2002). Modulation of human monocytes by Escherichia coli heat-labile enterotoxin B-subunit; altered cytokine production and its functional consequences. Immunology, 106, 316–325. 10.1046/j.1365-2567.2002.01429.x
- Wigley, P., Hulme, S., Powers, C., Beal, R., Smith, A. & Barrow, P. (2005). Oral infection with the Salmonella enterica serovar Gallinarum 9R attenuated live vaccine as a model to characterize immunity to fowl typhoid in the chicken. BMC Veterinary Research, 1, 1–6. 10.1186/1746-6148-1-2
- Wigley, P., Hulme, S.D., Bumstead, N. & Barrow, P.A. (2002). In vivo and in vitro studies of genetic resistance to systemic salmonellosis in the chicken encoded by the SAL1 locus. Microbes and Infection, 11, 1111–1120. 10.1016/S1286-4579(02)01635-0
- Yuki, Y. & Kiyono, H. (2003). New generation of mucosal adjuvants for the induction of protective immunity. Reviews in Medical Virology, 13, 293–310. 10.1002/rmv.398
- Zhang-Barber, L., Turner, A.K., Dougan, G. & Barrow, P.A. (1998). Protection of chickens against experimental fowl typhoid using a nuoG mutant of Salmonella serotype Gallinarum. Vaccine, 16, 899–903. 10.1016/S0264-410X(97)00300-9