Abstract
Streptococcus zooepidemicus has recently been shown to be a severe pathogen in layer chickens, where it is able to cause serious lesions in the vascular system. To evaluate the haemostatic response, 10 layer chickens were inoculated intravenously with S. zooepidemicus. Four hypotheses were tested: that the infection-induced inflammation would increase the plasma fibrinogen (Fbg) concentration, would prolong the prothrombin time (PT) and would prompt hypercoagulability or hypocoagulability as assessed by whole-blood thromboelastography (TEG), and that a possible correlation would exist between one of the TEG values and Fbg/PT. Each parameter was measured at days 1, 3 and 6 post inoculation (p.i.), and compared with the values at day 0 from each individual bird and with values obtained from non-infected control chickens (n = 10). In the infected chickens, the mean (± standard error) of Fbg was higher at day 3 p.i. (9.4 ± 1.4 g/l) and day 6 p.i. (8.0 ± 0.7 g/l) and the PT was prolonged at day 6 p.i. (168.1 ± 21.0 sec) compared with the day 0 standards (2.6 ± 0.2 g/l and 104.6 ± 2.0 sec, respectively) (P < 0.05). The majority of infected chickens demonstrated a hypercoagulable TEG result with increased mean values of the clot formation rate (α-angle) and maximal amplitude (MA) of TEG tracing at day 3 p.i. (83.1 ± 0.7°, 83.8 ± 1.4 mm) and day 6 p.i. (84.0 ± 0.4°, 89.8 ± 1.0 mm) compared with the day 0 values (75.8 ± 2.2° and 66.9 ± 1.4 mm, respectively) (P < 0.05). In control birds, the means of Fbg (1.5 ± 0.1 g/l), PT (79.4 ± 6.4 sec), TEG-α (76.7 ± 1.5°) and TEG-MA (64.0 ± 2.3 mm) were lower at day 6 compared with values observed for the infected chickens (P < 0.05). A negative correlation coefficient (−0.71) was found between the clot formation time (TEG-K) and Fbg at day 1 in the control group (P = 0.02). In conclusion, infection with S. zooepidemicus following intravenous injection in layer chickens induced haemostatic alterations including hyperfibrinogenaemia, prolonged PT, and hypercoagulability as measured by increased TEG-α and TEG-MA.
Introduction
Recently, Streptococcus equi subsp. zooepidemicus (S. zooepidemicus) has been shown to be a pathogen in adult layer chickens capable of causing severe lesions, and the clinical signs associated with its infection included respiratory disturbances, fever and diarrhoea lasting for several weeks leading to mortality between 18 and 80% (Bisgaard et al., Citation2012; Roy et al., Citation2013a). In these studies, septicaemia, congested vessels, spleen necrosis, valvular endocarditis, liver necrosis, oophoritis and airsacculitis were recorded in the infected chickens (Bisgaard et al., Citation2012; Roy et al., Citation2013a).
In chickens, the inflammatory response to infection has been reported to include an acute phase response with production of acute phase proteins—a group of blood proteins, for instance ovo-transferrin and transferrin (Xie et al., Citation2002; Cray et al., Citation2009; Roy et al., Citation2013b). These acute phase proteins are part of the innate immune response involved in restoring homeostatic balance by restricting growth of microorganisms and inflammation through an antibody-independent response (Murata et al., Citation2004). The coagulation factor fibrinogen is also considered an acute phase protein and can be increased upon activation by infection, inflammation or other mechanisms such as trauma (Donahue & Otto Citation2005; Weisel, Citation2005; Wagg et al., Citation2009; Davalos & Akassoglou, Citation2012). The synthesis of fibrinogen is expected to have a role in “walling off” infectious foci (Beutler, Citation2004). Chicken fibrinogen (Fbg) is thus considered a biomarker of persistent infections in routine haematological and biochemical screening tests (Hawkey & Hart, Citation1988).
Haemostatic alterations, as part of the innate immune response, have been described in response to acute inflammation during severe infection or trauma disturbing the delicate balance of pro-coagulant, fibrinolytic and anticoagulant factors, which can lead to development of disease complications such as disseminated intravascular coagulation (DIC) and microvascular thrombosis, described in mammalian species (Esmon, Citation2004; Levi & van der Poll, Citation2010; O'Brien, Citation2012). Although the basic mechanism of haemostasis and clot formation is considered similar in all vertebrates, including birds (Davidson et al., Citation2003; Gentry, Citation2004), few specific studies have been focused on avian haemostasis. The vascular changes observed in the studies cited above suggest that haemostatic alterations may be part of the pathophysiologic mechanisms in S. zooepidemicus infection.
Coagulation factors play a crucial role in maintaining normal haemostasis, and deficiencies of some of the important coagulation factors—for example, factor VII, factor X, factor V, factor II (prothrombin) and fibrinogen—involved in the enzymatic reaction of coagulation can be evaluated by the prothrombin time (PT) (Hoffman & Monroe, Citation2001; Lubas et al., Citation2010). In chickens, factors VII, X and V have been shown to be key factors in the tissue factor pathway of coagulation (Thomson et al., Citation2002). To reveal defects in the tissue factor pathway of coagulation or deficiency in the coagulation factors, the PT as a coagulation assay is commonly applied in birds (Morrisey et al., Citation2003). The prolonged PT was recorded in chickens infected with Erysipelothrix rhusiopathiae (Shibatani et al., Citation1997) and with infectious bursal disease virus (Lima et al., Citation2005), and in birds having aflatoxicosis (Fernandez et al., Citation1995).
Imbalances in haemostasis may lead to hypercoagulability or hypocoagulability (Donahue & Otto, Citation2005). Recently, whole blood thromboelastography (TEG) has been reported to enable measurement of hypercoagulability, hypocoagulability and also fibrinolysis (rate of reduction of amplitude of clot [LY]) in a wide range of animals. Whole blood TEG evaluates the overall haemostatic response attributable to both cellular and humoral components compared with the traditional plasma-based assays, which only evaluate initiation of plasma-based coagulation (Donahue & Otto, Citation2005; Wiinberg et al., Citation2008; Wagg et al., Citation2009; Wiinberg & Kristensen, Citation2010). TEG can reveal hypercoagulable or hypocoagulable states by tracing the reaction time (R), clot formation time (K), clot formation rate (α-angle) and clot strength or maximal amplitude of the clot (MA), respectively (Franz & Coetzee, Citation1981; Donahue & Otto Citation2005). To date, evaluation of haemostasis in birds by TEG has not been reported.
In the present study, we aimed at investigating whether haemostatic alterations are present during experimental S. zooepidemicus infection in layer chickens as well as at characterizing the response by evaluating changes in Fbg, PT and TEG during the course of the first week of the infection, assuming that haemostatic alterations are part of the pathophysiology in S. zooepidemicus infection in layer chickens.
Materials and Methods
Isolates
The normal variant of S. zooepidemicus (strain F122 Hj5) initially isolated from a natural outbreak in chickens from an organic layer farm in Denmark by Bisgaard et al. (Citation2012), was used in this study. The pathogenic potential of the strain has been reproduced experimentally in chickens by Roy et al. (Citation2013a).
Groups of chickens
The experimental study was conducted in 20-week-old brown layer chickens, weighing on average 800 g, obtained from a commercial flock with no history of disease. The chickens had been vaccinated against coccidiosis, infectious bronchitis, Newcastle disease, infectious bursal disease and avian encephalomyelitis, according to the general recommendations in Denmark. Two groups, referred to as S. zooepidemicus-infected and control, comprising 10 chickens in each were investigated. The control group was included to evaluate the effect of repeated venipuncture on coagulation. Each group was housed separately, and provided with ad libitum standard feed and water.
Experimental infections and sample collections
After 1 week of acclimatization, blood was collected from each bird and placed into two separate vacuum tubes (BD Vacutainer™; BD Diagnostic-Preanalytical Systems, Franklin Lakes, NJ, USA) containing 3.2% tri-sodium citrate, using a 23-G butterfly needle and without withdrawing the needle from the vein between tube changes. Subsequently, chickens belonging to the S. zooepidemicus-infected group were injected intravenously with 0.5 ml bacterial suspension (109 colony-forming units/ml in brain heart infusion broth) of the S. zooepidemicus isolate. Each bird in the control group received 0.5 ml sterile brain heart infusion broth. During the 6-day experiment, 1 ml blood was collected each day from each chicken of both groups for bacteriology. In addition, 1.8 ml blood (citrate:blood = 1:9) from each bird was collected at the same time of day 0 pre inoculation (day 0), and days 1, 3 and 6 post inoculation (p.i.) for haemostasis analysis. Various sites (brachial, jugular, basilic or ulnar vein) were used for venipuncture to avoid excessive trauma to the same site.
At day 6 p.i., all birds were euthanized after blood collection. A post-mortem examination was conducted, and swabs for bacteriological investigation were collected from the liver and spleen. All experimental procedures and animal management protocols were approved by the Danish Animal Ethics Committee (approval number: 2008/561-1481).
Laboratory analyses of haemostasis
The TEG measurements were performed following the procedures described previously by Wiinberg et al. (Citation2005) using a computerized thromboelastograph (TEG 5000 Haemostasis Analyzer; Haemoscope Corporation, Niles, IL, USA) modified for chicken blood. Undiluted recombinant human tissue factor was used as activator (Innovin; Dade-Behring, Marburg, Germany). The TEG analysis was initiated at a fixed time point of 30 min after blood collection. The blood collection tube was gently inverted five times, after which 400 µl whole citrated blood was added to a cryo-tube containing 25 µl tissue factor at the bottom. From this mixture, 340 µl was added to the pre-warmed cup (37°C) containing 20 µl of 0.2 M CaCl2. Each TEG tracing was run for at least 60 min with one channel for each sample. TEG parameters (R in minutes, K in minutes, α-angle in degrees, MA in mm) and fibrinolysis parameters (LY by 30 min [LY30%] and LY by 60 min [LY60%]) were measured. R was measured as the duration of latency from when the blood was placed in the TEG analyser until initial fibrin formation (an increase in amplitude of 2 mm); K as the period from the initial fibrin generation (i.e. from end of R) until the amplitude of 20 mm was reached; α-angle as the angle between the line in the middle of the TEG tracing and the line tangential to the developing body of the TEG; MA as the greatest amplitude of the TEG tracing; and LY as the rate of amplitude reduction by 30 min (LY30%) or 60 min (LY60%) after MA.
Plasma was extracted from the remaining citrated blood by centrifugation at 1500 × g for 3 min, and stored at −80°C. The stored plasma samples were thawed at 37°C for 3 min before use. PT and Fbg were measured (HemosIL® RecombiPlasTin 2G, ACL TOP 500 CTS system; Instrumentation Laboratory Co., Bedford, MA, USA). The assay was modified to determine PT and Fbg in chicken plasma by extending the test period from 100 to 400 sec and calculating the difference between the starting point and the highest measurement. Blood or plasma samples with inadequate volume or which were partially coagulated were discarded.
Criteria to detect haemostatic alterations
To evaluate haemostatic alterations in the chickens, the day 0 samples were considered normal in both groups. The minimum and maximum values derived from the day 0 healthy samples for each parameter were considered the reference range. The chickens having TEG values within the reference range were defined as normocoagulable. A hypercoagulable TEG tracing expresses a decreased R and K, increased α-angle and MA and also decreased LY, when based on previous studies in humans and dogs (Franz & Coetzee, Citation1981; Donahue & Otto, Citation2005), whereas the opposite change for each parameter is considered a hypocoagulable TEG tracing (Wiinberg et al., Citation2008; Wagg et al., Citation2009). In the present study, the same criteria of TEG-R, TEG-K, TEG-α, TEG-MA and TEG-LY were considered when evaluating whether the birds had normocoagulable, hypercoagulable or hypocoagulable TEG tracing.
Clinical signs, gross lesions, and bacteriology
During the whole trial, all ante-mortem and post-mortem abnormalities were cautiously noted. For bacteriological culture, swabs were smeared on blood agar base (CM 55; Oxoid, Basingstoke, UK), where 5% citrated bovine blood was added to make the blood agar. Then, the samples were incubated at 37°C in sealed plastic bags for 24 h. For blood cultures, 0.9 ml citrate-stabilized blood was added to 9 ml brain heart infusion broth in a test tube, incubated at 37°C for the same period, and subsequently examined for bacterial growth. If positive, isolates were sub-cultured on 5% bovine blood agar.
Test of hypotheses and statistical analysis
Four hypotheses were tested to evaluate the possible haemostatic alterations during S. zooepidemicus infection. To test the hypothesis that the infection-induced inflammation promotes hyperfibrinogenaemia (Hypothesis 1), the Fbg obtained at day 1, 3 and 6 p.i. in the experimental group was compared with the day 0 concentration for each bird and the corresponding concentrations in the control birds. Hyperfibrinogenaemia was represented by Fbg concentrations higher than day 0 (pre inoculation) and control chicken levels. The same procedure was followed for the PT and also for TEG along with the fibrinolysis parameters to test the hypotheses that the infection-induced inflammation prolongs the PT (Hypothesis 2) and that the infection-induced inflammation prompts hypercoagulability or hypocoagulability as assessed by TEG (Hypothesis 3), respectively. For these three hypotheses, descriptive statistics was performed to calculate the mean and standard error. A one-way analysis of variance (ANOVA) was used to compare the differences in the mean values at each sampling point for each parameter, the Tukey's multiple comparison test was used to compare the differences between the mean values at the individual days within group and between groups in a multiple comparisons manner, and the Fisher exact test was used to compare frequencies of chickens showing different values of the parameters or the haemostatic states at the specified days. All tests were performed at a 95% confidence level. Finally, to test the hypothesis that the Fbg concentration and the PT are correlated with the individual values of the TEG parameters during a hypercoagulable or hypocoagulable state (Hypothesis 4), Pearson's correlation coefficient (r) was calculated to evaluate the correlation between one of the TEG values (R, K, α and MA) and Fbg, and between TEG-R and PT, on the basis of the values for each specified day in both groups. GraphPad Prism software (Version 5; GraphPad Software, San Diego, CA, USA) was used for data analyses and graphical presentation.
Results
Clinical signs, gross lesions and bacteriological re-isolation rates
As clinical signs, reluctance to move spontaneously and depression were recorded in three out of the 10 infected chickens. Gross lesions with variable distributions in different organs were observed in all infected chickens. The most commonly encountered lesions were splenomegaly (8/10) and ovarian regression (9/10). Two birds had white necrotic foci in the spleen, and one chicken had purulent oophoritis. Pure growth of S. zooepidemicus was obtained from liver and spleen swabs of 10 and nine infected chickens, respectively. The number of infected chickens' positive for S. zooepidemicus in blood culture was 10 at day 1 and day 2, seven at day 3, nine at day 4 and day 5, and five at day 6 p.i. No bacterial growth was detected from any of the day 0 blood samples, and none of the chickens in the non-infected control group showed any clinical sign or lesion and remained culture-negative during the entire experiment.
Fibrinogen, prothrombin time and thromboelastography
The mean Fbg concentration () was significantly higher in the infected chickens at day 3 and day 6 p.i. compared with means observed at day 0 and day 1 p.i. and in the corresponding control chickens. The mean PT () was significantly prolonged in the infected chickens at day 6 p.i. compared with means observed at day 0 and day 1 p.i and in the corresponding control chickens.
![Figure 1. Fibrinogen concentrations at specified days in the layer chickens. S. zoo: means (± standard error [SE]) of Fbg concentrations were 2.6 ± 0.2 g/l on day 0, 4.2 ± 0.6 g/l on day 1 p.i., 9.4 ± 1.4 g/l on day 3 p.i., and 7.9 ± 0.7 g/l on day 6 p.i., carrying the significant difference in the means by ANOVA (P < 0.01). The reference range was 1.9 to 3.4 g/l, and indicated the increased Fbg in 5/7, 10/10 and 7/7 chickens at day 1, day 3 and day 6 p.i., respectively. Control: means of Fbg concentrations were 2.3 ± 0.3 g/l, 1.7 ± 0.1 g/l, 1.7 ± 0.1 g/l and 1.5 ± 0.1 g/l at the specified days, respectively (P = 0.03). The reference range was 1.4 to 4.8 g/l. The concentrations of Fbg in 8/10, 7/10 and 6/10 chickens were within the reference range at days 1, 3 and 6 p.i., respectively. Day-1, day 1 p.i.; S. zoo, S. zooepidemicus group; mid horizontal line, mean value; error bars, standard error of mean; different shaped black symbols, observations on samples; n, number of samples tested; horizontal lines from day 0 observations, minimum and maximum values from these samples, indicating the reference range in each group; vs, versus.](/cms/asset/e4c6c836-dff4-481c-b65c-c6486de562f6/cavp_a_938608_f0001_b.jpg)
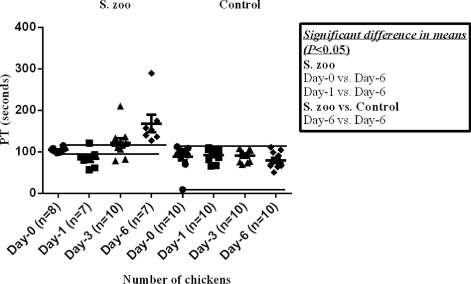
The mean value of TEG-α () was significantly higher in the infected chickens at day 3 and day 6 p.i. compared with the values observed in the group at day 0 and day 1 p.i. Furthermore, the mean value of TEG-α was significantly higher in the infected chickens at day 6 p.i. compared with control chickens at the corresponding day. The mean value of TEG-MA () was significantly higher in the infected chickens at day 3 and day 6 p.i. compared with the values observed in the group at day 0 and day 1 p.i. and compared with the control chickens at the corresponding days.
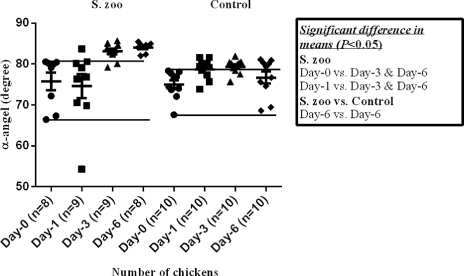
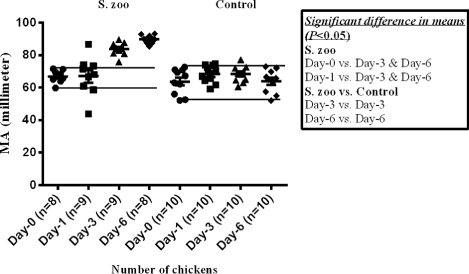
The mean values of TEG-R and TEG-K () in the infected chickens were decreased insignificantly on the specified days (P = 0.86 and P = 0.21, respectively, in ANOVA). Based on the normocoagulable range, a shortened TEG-R value (1 to 2.7 min) representing a hypercoagulable tracing was observed in 2/9, 3/9 and 1/8 chickens at days 1, 3 and 6 p.i., respectively, and a prolonged TEG-R value (7.9 and 9.3 min) representing a hypocoagulable tracing was recorded in 2/9 birds at day 3 p.i. Similarly, an increased TEG-K value (3 min) representing a hypocoagulable tracing was observed in 1/9 chicken at day 1 p.i. In the control group, all observations were within the normocoagulable range in both TEG-R and TEG-K.
Table 1. Values of TEG-R, TEG-K and TEG-LY in the chickens.
In both infected and control groups, the mean values of TEG-LY30% and TEG-LY60% () did not differ significantly over the specified days in ANOVA (P > 0.05). In the infected chickens, all individual values were within the reference ranges for both LY. In the control group, the TEG-LY30% values of 7/10, 7/10 and 4/10 chickens, and the TEG-LY60% values of 8/10, 7/10 and 4/10 chickens were within the reference ranges at days 1, 3 and 6 p.i., respectively, and the remaining birds were recorded with values (TEG-LY30%, 0.1 to 4.9; TEG-LY60%, 0.7 to 6.5) above the upper limit of the reference range. There was a significant difference between the mean values on day 0 and day 6 p.i. for TEG-LY60% in the control chickens (P < 0.05).
Correlation between coagulation parameters
The correlations between the TEG values and the plasma-based coagulation parameters are summarized in . A significant correlation coefficient was observed between the TEG-R value and PT at day 0 (r = 0.66, P = 0.04), day 1 (r = 0.75, P = 0.01) and day 3 (r = 0.69, P = 0.03), and between the TEG-K value and Fbg at day 1 p.i. (r = −0.71, P = 0.02) in the control samples.
Table 2. Correlation coefficient between one of the TEG values and Fbg or PT.
Discussion
The significantly increased means of Fbg at day 3 and day 6 p.i. compared with their day 0 and corresponding control concentrations indicated the presence of hyperfibrinogenaemia in the infected chickens as a result of an acute phase response, which supports Hypothesis 1. Hyperfibrinogenaemia has been demonstrated previously in chickens as a response to bacterial infections by Hawkey & Hart (Citation1988).
The significantly prolonged PT at day 6 p.i. in the infected chickens indicated a possible defect in the tissue factor pathway of coagulation, probably representing a consumptive coagulopathy (Jain, Citation1986; Thomson et al., Citation2002; Lubas et al., Citation2010) in the infected birds, supporting Hypothesis 2. A prolonged PT has previously been demonstrated as a result of DIC in response to E. rhusiopathiae infection in chickens (Shibatani et al., Citation1997).
The increased mean values of TEG-α and TEG-MA at day 3 and day 6 p.i. compared with their day 0 and day 1 p.i. and the corresponding control means indicated the presence of an overall hypercoagulable state in the majority of S. zooepidemicus-infected chickens. The mean values of TEG-R, TEG-K and TEG-LY, which were insignificantly decreased over the specified days compared with their day 0 values, also implied or reflected the presence of a hypercoagulable state in the infected group. A hypocoagulable state was only recorded in few infected chickens with individual values of increased TEG-R and TEG-K, and decreased TEG-α and TEG-MA based on the normocoagulable range. The findings thus demonstrate that S. zooepidemicus infection was associated with haemostatic alterations, resulting in most chickens in overall hypercoagulability and others in hypocoagulability, supporting Hypothesis 3. The increased Fbg and impaired fibrinolysis may explain the finding of hypercoagulability. In addition, this abnormality might also result from activities of associated coagulation factors (for example, factor II, factor VIII and factor XIII) and decreased antithrombin (Franz & Coetzee, Citation1981), which were not investigated in the present study. The hypocoagulation might result from sepsis associated with DIC and thrombocyte deficiency or dysfunction as well as coagulation factor consumption (Donahue & Otto, Citation2005). As DIC in other species, it has been shown to range from hypercoagulability (non-overt DIC) to hypocoagulability (overt-DIC) depending on the severity of the overall inflammatory and haemostatic system response (Taylor et al., Citation2000, Wiinberg et al., Citation2008), and the observed haemostatic alterations in the group of S. zooepidemicus-infected chickens may represent a similar response.
Although significant correlation was found at some points between TEG-R and PT, and between TEG-K and Fbg in the control group, the study did not find any significant correlation between the values at any specified days in the post-infection samples. This might be explained by insignificant differences in the mean values over the specified days in TEG-R and TEG-K, and insignificant differences between the mean values of day 0 and day 1 p.i. in the case of TEG-α, TEG-MA, Fbg and PT. Again, although the shortening of the PT is suggestive of a hypercoagulable state (Green & Thomas, Citation1995), such a PT has not been considered a consistent or reliable predictor of hypercoagulability (Otto et al., Citation2000). Moreover, a significantly prolonged PT was observed in the infected chickens in the present study. In this relation, the significant positive correlation recorded between TEG-R and PT in the control group of present study is also controversial. However, positive correlation between TEG-MA and Fbg, and between TEG-R and PT has recently been demonstrated in dogs by Wagg et al. (Citation2009) and in horses by Epstein et al. (Citation2009), suggesting that further studies should evaluate the possible correlation between Fbg concentration and TEG-α/TEG-MA, and between PT and TEG-R value in hypercoagulability in chickens. Bacteriology revealed that all infected chickens were positive with pure colonies of the S. zooepidemicus, confirming that the bacteraemia and lesions were caused by the experimental infection. Interestingly, however, at day 1 p.i. the TEG tracings only recorded hypercoagulable or hypocoagulable states in one to three birds out of the nine chickens. The reason might be due to individual responses in the birds to the pathogen, or to the nature of the infection which has not yet affected the overall haemostatic balance at day 1 p.i. None of the birds in the control group were culture-positive or had detectable lesions and generally had coagulation parameters within the reference or normocoagulable ranges, suggesting the presence of balanced haemostasis in the non-infected chickens. However, a few chickens in the control group experienced values of TEG-α, TEG-MA, TEG-LY and Fbg outside the reference or baseline values post brain heart infusion broth injection. The present study therefore emphasizes the need for a control group undergoing similar procedures such as injections and multiple blood samplings. Despite the few unexpected results in the control group, we consider the results obtained from the control birds reliable as judged by the non-significant difference in the means compared day by day for most parameters. The present study used the day 0 samples in both infected and control groups as baseline to minimize the effect of the individual bird's physiology on the results.
Overall, in the infected chickens significant haemostatic alterations were documented as part of the S. zooepidemicus infection, characterized by significantly increased Fbg concentrations on day 3 and 6 p.i., prolonged PT on day 6 p.i., and TEG hypercoagulability in the majority of the infected chickens as evidenced by increased TEG-α and TEG-MA values at day 3 and day 6 p.i. compared with their corresponding day 0 healthy and control chickens. The present study supports the assumption that haemostatic alterations are part of the pathophysiologic response in S. zooepidemicus infection in this group of chickens. In addition, the hypercoagulability assessed by increased TEG-α and TEG-MA in the chickens probably represents non-overt DIC.
In conclusion, S. zooepidemicus infection following intravenous injection in layer chickens induces a haemostatic response characterized by increased Fbg, prolonged PT, and TEG hypercoagulability in the birds.
Acknowledgements
The authors would like to thank University of Copenhagen for funding this work. For excellent technical assistance, the authors would like to express their gratitude to Anette U. Martinsen (laboratory technician), Louise Bochsen (laboratory technician) and Clara Buchner Marschner (PhD student) of the Department of Veterinary Clinical and Animal Sciences, and to Pia Mortensen (laboratory technician), Ljudmila Troianova (laboratory technician), Bent Aalbæk (Associate Professor), Ida Thøfner (PhD student) and Ragnhild Jorgensen Bager (PhD student) of the Department of Veterinary Disease Biology, Faculty of Health and Medical Sciences, University of Copenhagen, Denmark.
References
- Beutler, B. (2004). Innate immunity: an overview. Molecular Immunology, 40, 845–859. 10.1016/j.molimm.2003.10.005
- Bisgaard, M., Bojesen, A.M., Petersen, M.R. & Christensen, H. (2012). A major outbreak of Streptococcus equi subsp. zooepidemicus infections in free range chickens is linked to horses. Avian Diseases, 56, 561–566. 10.1637/10123-030712-Reg.1
- Cray, C., Zaias, J. & Altman, N.H. (2009). Acute phase response in animals: a review. Comparative Medicine, 59, 517–526.
- Davalos, D. & Akassoglou, K. (2012). Fibrinogen as a key regulator of inflammation in disease. Seminars in Immunopathology, 34, 43–62. 10.1007/s00281-011-0290-8
- Davidson, C.J., Hirt, R.P., Lal, K., Snell, P., Elgar, G., Tuddenham, E.G.D. & Mcvey, J.H. (2003). Molecular evaluation of the vertebrate blood coagulation network. Thrombosis and Hemostasis, 89, 420–428.
- Donahue, S.M. & Otto, C.M. (2005). Thromboelastography: a tool for measuring hypercoagulability, hypocoagulability and fibrinolysis. Journal of Veterinary Emergency and Critical Care, 15, 9–16. 10.1111/j.1476-4431.2005.04025.x
- Epstein, K.L., Brainard, B.M., Lopes, M.A.F., Barton, M.H. & Moore, J.N. (2009). Thrombelastography in 26 healthy horses with and without activation by recombinant human tissue factor. Journal of Veterinary Emergency and Critical Care, 19, 96–101. 10.1111/j.1476-4431.2008.00381.x
- Esmon, C.T. (2004). Interactions between the innate immune and blood coagulation Systems. TRENDS in Immunology, 25, 536–542. 10.1016/j.it.2004.08.003
- Fernandez, A., Verde, M.T., Gomez, J., Gascon, M. & Ramos, J.J. (1995). Changes in the prothrombin time, haematology and serum proteins during experimental aflatoxicosis in hens and broiler chickens. Research in Veterinary Science, 58, 119–122. 10.1016/0034-5288(95)90063-2
- Franz, R.C. & Coetzee, W.J. (1981). The thromboelastographic diagnosis of hemostatic defects. Surgery Annual, 13, 75–107.
- Gentry, P.A. (2004). Comparative aspects of blood coagulation. The Veterinary Journal, 168, 238–251. 10.1016/j.tvjl.2003.09.013
- Green, R.A. & Thomas, J.S. (1995). Hemostatic disorders: coagulopathies and thrombosis. In S.J. Ettinger & E.C. Feldman (Eds.). Textbook of Veterinary Internal Medicine 4th edn. (pp. 1946–1963). Philadelphia, PA: WB Saunders.
- Hawkey, C. & Hart, M.G. (1988). An analysis of the incidence of hyperfibrinogenaemia in birds with bacterial infections. Avian Pathology, 17, 427–432. 10.1080/03079458808436460
- Hoffman, M. & Monroe, D.M. (2001). Review article: a cell based model of hemostasis. Journal of Thrombosis and Hemostasis, 85, 958–965.
- Jain, N.C. (1986). Schalm's Veterinary Hematology 4th edn. (pp. 563–576). Philadelphia, PA: Lea & Febiger.
- Levi, M. & van der Poll, T. (2010). Inflammation and coagulation. Critical Care Medicine, 38, S26–S34. 10.1097/CCM.0b013e3181c98d21
- Lima, A., Fehervari, T., Paasch, L.H. & Calderón, N.L. (2005). Hematological and histological findings in Leghorn chickens infected with infectious bursal disease virus strain 73668. Acta Veterinaria Hungarica, 53, 501–506. 10.1556/AVet.53.2005.4.10
- Lubas, G., Caldin, M., Wiinberg, B. & Kristensen, A.T. (2010). Laboratory testing of coagulation disorders. In D.J. Weiss & K.J. Wardrop (Eds.). Schalm's Veterinary Hematology 6th edn (pp. 1082–1100). Ames, IA, USA: Wiley-Blackwell.
- Morrisey, J.K., Paul-Murphy, J., Fialkowski, J.P., Hart, A. & Darien, B.J. (2003). Estimation of prothrombin times of Hispaniolan Amazon parrots (Amazona ventralis) and Umbrella cockatoos (Cacatua alba). Journal of Avian Medicine and Surgery, 17, 72–77. 10.1647/2001-045
- Murata, H., Shimada, N. & Yoshioka, M. (2004). Current research on acute phase proteins in veterinary diagnosis: an overview. The Veterinary Journal, 168, 28–40. 10.1016/S1090-0233(03)00119-9
- O'Brien, M. (2012). The reciprocal relationship between inflammation and coagulation. Topics in Companion Animal Medicine, 27, 46–52. 10.1053/j.tcam.2012.06.003
- Otto, C.M., Rieser, T.M., Brooks, M.B. & Russell M.W. (2000). Evidence of hypercoagulability in dogs with parvoviral enteritis. Journal of the American Veterinary Medical Association, 217, 1500–1504. 10.2460/javma.2000.217.1500
- Roy, K., Bisgaard, M., Kyvsgaard, N.C., Christensen, J.P., Nielsen, O.L., Biswas, P.K., Pors, S.E. & Bojesen, A.M. (2013a). Pathogenicity of wild-type and small-colony variants of Streptococcus equi subsp. zooepidemicus in layer chickens. Avian Pathology, 42, 316–322. 10.1080/03079457.2013.798396
- Roy, K., Kjelgaard-Hansen, M., Pors, S.E., Christensen, J.P., Biswas, P.K. & Bojesen, A.M. (2013b). Performance of a commercial Chicken-Ovo-transferrin-ELISA on the serum of brown layer chickens infected with Gallibacterium anatis and Streptococcus zooepidemicus. Avian Pathology, 43, 57–61
- Shibatani, M., Suzuki, T., Chujo, M. & Nakamura, K. (1997). Disseminated intravascular coagulation in chickens inoculated with Erysipelothrix rhusiopathiae. Journal of Comparative Pathology, 117, 147–156. 10.1016/S0021-9975(97)80031-X
- Taylor, F.B. Jr., Wada, H. & Kinasewitz, G. (2000). Description of compensated and uncompensated disseminated intravascular coagulation (DIC) responses (non-overt and overt DIC) in baboon models of intravenous and intraperitoneal Escherichia coli sepsis and in the human model of endotoxemia: toward a better definition of DIC. Critical Care Medicine, 28, 12–9. 10.1097/00003246-200009001-00004
- Thomson, A.E., Squires, E.J. & Gentry, P.A. (2002). Assessment of factor V, VII and X activities, the key coagulant proteins of the tissue factor pathway in poultry plasma. British Poultry Science, 43, 313–321. 10.1080/00071660120121553
- Wagg, C.R., Boysen, S.R. & Bedard, C. (2009). Thromboelastography in dogs admitted to an intensive care unit. Veterinary Clinical Pathology, 38, 453–461. 10.1111/j.1939-165X.2009.00161.x
- Weisel, J.W. (2005). Fibrinogen and fibrin. Advances in Protein Chemistry, 70, 247–299.
- Wiinberg, B., Jensen, A.L., Rojkjaer, R., Johansson, P., Kjelgaard-Hansen, M. & Kristensen, A.T. (2005). Validation of human recombinant tissue factor-activated thromboelastography on citrated whole blood from clinically healthy dogs. Veterinary Clinical Pathology, 34, 389–393. 10.1111/j.1939-1676.2008.0058.x
- Wiinberg, B., Jensen, A.L., Johansson, P.I., Rozanski, E., Tranholm, M. & Kristensen, A.T. (2008). Thromboelastographic evaluation of hemostatic function in dogs with disseminated intravascular coagulation. Journal of Veterinary Internal Medicine, 22, 357–365. 10.1111/j.1939-1676.2008.0058.x
- Wiinberg, B. & Kristensen, A.T. (2010). Thromboelastography in veterinary medicine. Seminars in Thrombosis and Hemostasis, 36, 747–756. 10.1055/s-0030-1265291
- Xie, H., Newberry, L., Clark, F.D., Huff, W.E., Huff, G.R., Balog, J.M. & Rath, N.C. (2002). Changes in serum ovotransferrin levels in chickens with experimentally induced inflammation and diseases. Avian Diseases, 46, 122–131. 10.1637/0005-2086(2002)046[0122:CISOLI]2.0.CO;2