Abstract
Fowl glioma is characterized morphologically by multiple nodular astrocytic growth with disseminated non-suppurative encephalitis. The disease is caused by fowl glioma-inducing virus (FGV) and its variants, belonging to subgroup A of avian leukosis virus (ALV-A). Fifty-seven FGV variants have so far been isolated from Japanese fowls and these variants have a variable degree of glioma inducibility. However, how these ALVs induce glioma with different degrees and frequencies has not been fully elucidated. In this study, we investigated the relationship between intracerebral viral replication and astrocytic growth in the early infectious phase. Replication abilities of two ALV strains, Sp-53 (a FGV variant) and ALV-based replication-competent vector RCAS(A) without glioma inducibility, were compared in the brains of C/O specific pathogen free chickens at 35 days of age. Sp-53 replicated faster than RCAS(A), and the histological score and the level of interleukin (IL)-1β in brains increased depending on the level of intracerebral viral RNA. Up-regulation of IL-1β was also demonstrated in primary cultured astrocytes. These results suggest that the astrocytic growth in this phase is enhanced through the autocrine/paracrine production of IL-1β in the FGV-infected astrocytes.
Introduction
Fowl glioma is characterized histopathologically by multiple nodular astrocytic growths with disseminated non-suppurative encephalitis (Summers et al., Citation1994; Swayne et al., Citation2008). This disease has been reported as various disease entities in the past (Belmonte, Citation1935; Jungherr & Wolf, Citation1939; Jackson, Citation1954; Biering-Sørensen, Citation1956; Wight & Duff, Citation1964; Reece, Citation2008). We have reported that it is caused by fowl glioma-inducing virus (FGV) and its variants, belonging to subgroup A of avian leukosis virus (ALV-A) (Ochiai et al., Citation1999; Iwata et al., Citation2002; Tomioka et al., Citation2003). The main brain lesions can be divided histologically into three types: non-suppurative inflammation, gliosis, and glioma (Iwata et al., Citation2002). Perivascular cuffing, microgliosis and subependymal gliosis are scattered throughout the brain in the initial infectious phase. Subsequently, astrocytes proliferate in response to these inflammatory reactions and some of the astrocytes seem to develop discrete neoplastic nodules (fowl glioma). FGV has a unique sequence in the 3′-untranslated region domain, which is not detected in standard strains of ALVs (Tomioka et al., Citation2004). Fifty-seven FGV variants have been isolated from swab or brain tissue in culture and these variants were confirmed to have an FGV-specific region in their genome using FGV-specific nested polymerase chain reaction (PCR) (Hatai et al., Citation2005; Ochi et al., Citation2012). Phylogenic analysis of the envSU region of these variants indicated that they evolved in three clusters. In addition, FGV variants with 85 to 95% identity of envSU with FGV still have the ability to induce brain lesions, but the frequency and severity of astrocytic proliferation differ among them (Nakamura et al., Citation2011). However, the possibility that nodular lesions of astrocytes are directly related to the rapid and abundant replication of FGV and the variants in the brain could not be ruled out previously (Nakamura et al., Citation2011).
Although other retroviruses, including human immunodeficiency virus type 1 (HIV-1), human T-lymphotropic leukaemia virus and simian immunodeficiency virus, occasionally cause non-suppurative encephalitis, only limited strains of ALV-A can induce marked astrocytic growth. Cytokine expression has important roles in various diseases, including infectious disease, neoplasms and autoimmune diseases. Among various cytokines, the proinflammatory cytokine interleukin (IL)-1β plays key roles in infectious diseases and tumours. Up-regulation of IL-1β has been reported in brains infected with HIV-1 (Merrill & Chen, Citation1991), highly pathogenic avian influenza virus (Wang et al., Citation2008) and Marek's disease virus (Parvizi et al., Citation2010). IL-1β is also up-regulated in primary cultured astrocytes from HIV-1-infected patients, which suggests that this cytokine is an important factor for the neuropathogenesis of HIV-1 (Coyle-Rink et al., Citation2002). On the other hand, the proliferation and survival of astrocytes could be promoted by IL-1β in the microenvironment of human glioblastoma (Sen, Citation2011; Sharma et al., Citation2011; Yeung et al., Citation2013). We investigated the viral load and the amount of cytokines in the early infectious phase of a FGV variant, to clarify the association between them. Here, we describe that autocrine/paracrine production of IL-1β from astrocytes could mediate the glial proliferation in this infectious phase.
Materials and Methods
Virus and cell culture
Sp-53 is one of the strains that were isolated from a Japanese chicken affected with fowl glioma; this strain induced the most severe brain lesions in an experimental infection study (Nakamura et al., Citation2011). A replication-competent ALV-based vector, RCAS(A), was kindly donated by Dr Stephen H. Hughes (National Cancer Institute, Bethesda, MD, USA). DF-1 cells were obtained from the American Type Culture Collection (Manassas, VA, USA). Cell culture and preparation of the viral suspension were performed using methods described previously (Iwata et al., Citation2002). The propagation of RCAS(A) was initiated by transfection of 1 µg plasmid DNA into DF-1 cells using FuGENE HD transfection reagent (Promega, Madison, WI, USA), according to the manufacturer's instructions. For inoculation, 100-fold concentrated culture supernatant was prepared according to the method described by Bowles et al. (Citation1996).
Primary chicken astrocytic culture was prepared by a method described previously (Peters et al., Citation2005). DF-1 cells and primary chicken astrocytes were infected with Sp-53 and RCAS(A) by adding viral suspension containing 5 × 104 tissue culture infectious units (TCIU) of each virus. After infection, cell supernatants were collected every 24 h and cultured cells were collected at intervals of 3 days until 9 days post infection (d.p.i.).
Birds and experimental infection
Fertile eggs from specific pathogen free chickens of the WL-M/O (C/O) strain were used in the bird infection experiment. Fertile eggs of the commercial specific pathogen free White Leghorn strain WL-M/O were purchased from the Nippon Institute for Biological Science (Tokyo, Japan). This strain lacks both chicken helper factor and group-specific antigen and is susceptible to ALV subgroups A to E (International Registry of Poultry Genetic Stocks, Bulletin 476, March 1988, Ralph G. Somes, Jr, University of Connecticut, Storrs, CT, USA). The presence of endogenous viral genes other than chicken helper factor and group-specific antigen is not known. Chicken embryos were inoculated via the yolk sac on the sixth day of incubation with 5 × 102, 5 × 104 or 5 × 106 TCIU Sp-53. As a control group, embryos were inoculated with 5 × 102, 5 × 104 or 5 × 106 TCIU RCAS(A). These chickens were euthanized at 35 days of age. The hatched chickens were reared humanely according to the guidelines set by the Animal Care and Use Committee of our institute. No chickens received any vaccinations or medication.
Histopathology and immunohistochemistry
For histological examination, the chickens were euthanized humanely according to a procedure approved by Hokkaido University. The cerebrum, cerebellum and other organs, including the liver, spleen, kidney and lung, were fixed in 20% neutral-buffered formalin, routinely processed and embedded in paraffin wax. Sections (4 µm) were cut and stained with haematoxylin and eosin. The scores of histopathologic changes were defined as follows: Grade 0, no lesions; Grade 1, only perivascular cuffing; Grade 2, perivascular cuffing with microglial infiltration; Grade 3, astrocytic growth consisting of fewer than 10 astrocytes in addition to Grade 2 lesion; Grade 4, astrocytic growth consisting of more than 10 astrocytes in addition to Grade 2 lesion; and Grade 5, multiple astrocytic growth with Grade 2 lesion. Immunostaining using the labelled streptavidin–biotin method (Nichirei Corp, Tokyo, Japan) was performed for glial fibrillary acidic protein and IL-1β, using primary antibodies of rabbit-anti-cow glial fibrillary acidic protein (DAKO Co., Copenhagen, Denmark) at 1:2500 dilution and rabbit-anti-IL-1β (Biorbyt, Cambridge, UK) at 1:200 dilution.
RNA extraction and cDNA synthesis
Total RNA was extracted from brains, cultured primary chicken astrocytes and DF-1 cells using TRizol reagent (Invitrogen Life Technologies, Carlsbad, CA, USA). RNA in each sample was quantified using an ND-1000 spectrophotometer (Nanodrop Technologies, Inc., Wilmington, DE, USA). Then 1000 ng RNA from each sample was used to produce cDNA. Reverse transcription (RT) was performed as described previously (Hatai et al., Citation2008). As an internal control for RNA extraction and cDNA synthesis, PCR amplification was performed for all cDNA samples using primers specific to chicken β-actin. Primer sequences were as follows: chicken β-actin forward, 5′-TAT CCG TAA GGA TCT GTA TG-3′; and chicken β-actin reverse, 5′-ATC TCG TCT TGT TTT ATG CG-3′. The PCR products were analysed by 2% agarose gel electrophoresis.
Quantitative real-time RT-PCR
The quantitative real-time RT-PCR was performed using the primers presented in and the StepOne Real Time PCR system (Applied Biosystems, Foster City, CA, USA). Amplified mixtures were 20 µl reaction volumes containing 1 µl cDNA, 10 µl Fast SYBR Green Master Mix (2×), 0.4 µl each primer (10 µM) and 8.2 µl water. PCR conditions were the same for each targeted gene and were as follows: 20 sec at 95°C followed by 40 cycles of 3 sec at 95°C and 30 sec at 60°C.
Table 1. Sequence of the oligonucleotide primers used in quantitative RT-PCR.
Enzyme-linked immunosorbent assay
ALV common antigen (p27) in the supernatant of the cell culture was measured using enzyme-linked immunosorbent assay (Avian Leukosis Virus Antigen Test Kit; IDEXX Laboratories, Inc., Westbrook, ME, USA), following the manufacturer's instructions.
Calculations and statistics
Expression of each target gene was determined by the –ΔΔCt method using β-actin as an endogenous reference gene to normalize the level of target gene expression. Logarithmic transformation was performed on each value before analysis by Student's t test, analysis of variance and the Kruskal–Wallis test.
Results
Glioma-inducibility in chickens inoculated with different viral loads
Chickens inoculated with three different viral titres of Sp-53 were analysed histopathologically and the mRNA expression of cytokines in brains was evaluated. Multiple astrocytic growth (,) was observed in three of five (60%) chickens inoculated with 5 × 106 TCIU and two of three (67%) chickens inoculated with 5 × 104 TCIU. In contrast, chickens with 5 × 102 TCIU had no astrocytic growth. Histological scores were significantly higher in the 5 × 104 and 5 × 106 TCIU-inoculated groups than in the 5 × 102 TCIU-inoculated group (). Intracerebral viral RNA levels were also greater in 5 × 106 TCIU-inoculated chickens than in 5 × 102 TCIU-inoculated birds. The mRNA levels of IL-1β in the brains were much higher in the 5 × 106 TCIU-inoculated group than in the 5 × 102 TCIU-inoculated group, which depended on the viral mRNA levels in the brains. The mRNA levels of transforming growth factor (TGF)-β increased in the 5 × 104 TCIU-inoculated group compared with those in the 5 × 102 TCIU-inoculated group, although there was no significant difference between the 5 × 102 and 5 × 106 TCIU-inoculated groups.
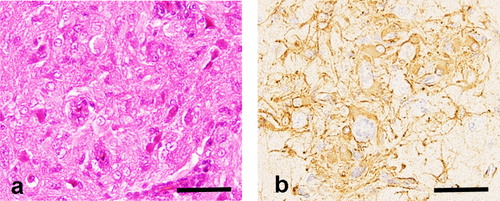
Table 2. Histological score, viral RNA and cytokine expression (relative units) in brain of chickens inoculated with different titres of Sp-53.
Glioma inducibility of Sp-53
Sp-53 induced multiple astrocytic growth in three of five (60%) inoculated chickens. However, RCAS(A) induced only minimal gliosis, which consisted of fewer than 10 astrocytes in two of five (40%) inoculated birds and showed no gliomatous growth. According to the scoring system described in Materials and Methods, the Sp-53-inoculated group revealed a significantly higher histological score than the RCAS(A)-inoculated group (). Real-time PCR analysis showed that viral RNA levels were significantly higher in the brains of Sp-53-inoculated chickens than in RCAS(A)-inoculated birds. The mRNA expression of IL-4 was up-regulated in the brains of RCAS(A)-inoculated chickens compared with that in Sp-53-inoculated birds, whereas the mRNA levels of TGF-β were significantly up-regulated and the RNA levels of IL-1β were considerably higher in the brains of Sp-53-inoculated chickens than in those of RCAS(A)-inoculated birds.
Table 3. Histological score, viral RNA and cytokine expression (relative units) in brain of chickens infected with Sp-53 and RCAS(A).
Viral replication in DF-1 cells and primary chicken astrocytes
Replication kinetics of Sp-53 and RCAS(A) were estimated in DF-1 fibroblasts and primary cultured astrocytes. ALV common antigen p27 and viral RNA of both viruses were detected from 2 d.p.i. in DF-1 cells (,), and Sp-53 replicated more rapidly than RCAS(A), with a peak at 4 d.p.i. in viral protein in cultured supernatant. Similarly, Sp-53 replicated in astrocytes showed more rapid replication than RCAS(A), with a peak at 7 d.p.i. in viral protein in supernatant (,). Compared with DF-1, p27 of RCAS(A) was rarely detected in the supernatant of infected astrocytes. Antigen p27 and viral RNA were not detected in both control cells. Additionally, the mRNA levels of IL-1β were higher in Sp-53-infected astrocytes (mean mRNA level = 3.20 ± 0.49, calculated by the –ΔΔCt method using β-actin as an internal control, n = 2) than in RCAS(A)-infected cells (0.52 ± 0.12, n = 2) at 9 d.p.i.
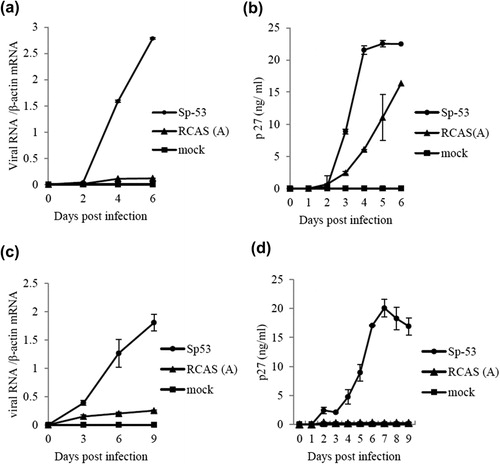
Discussion
Fowl glioma is defined as multiple nodular growths of astrocytes with disseminated non-suppurative encephalitis. In this study, we investigated the relationship between intracerebral viral replication and astrocytic growth in the early infectious phase. Among the C/O specific pathogen free chickens inoculated in ovo with three different titres of Sp-53 (a FGV variant), chickens with higher titres revealed multiple proliferation of astrocytes and the levels of IL-1β in those brains increased in close correlation with the viral load and intracerebral viral replication. Additionally, Sp-53 replicated more quickly and at higher titres in DF-1 cells and primary cultured astrocytes, and enhanced the excessive release of IL-1β from the infected astrocytes.
The level of viral antigen is not always correlated with neurological disease progression associated with retroviruses, including HIV-1, human T-lymphotropic leukaemia virus-1 and simian immunodeficiency virus. In macaques with simian immunodeficiency virus, a high viral load in cerebrospinal fluid and brain tissue could be predictive of the development of central nervous system lesions associated with these viral infections (Zink et al., Citation1999: Dang et al., Citation2012). On the other hand, some affected individuals showed no correlation between central nervous system lesions and cerebrospinal fluid viral load (Matsuda et al., Citation2013). In the current study, the positive correlation between intracerebral viral load and degree of astrocytic growth was recognized in the early infectious phase, indicating that the inflammatory response to viral infection is dominant in this phase.
The levels of IL-1β were correlated with intracerebral viral RNA levels of Sp-53 in the experimental infection, and cultured astrocytes infected with Sp-53 excessively released this proinflammatory cytokine following successful viral replication. IL-1β affects cell proliferation and the survival of neoplastic astrocytes via NF-κB, p38/MAPK and JNK pathways (Sen, Citation2011; Yeung et al., Citation2013). Activated astrocytes themselves produce IL-1β and enhance its effects in an autocrine and paracrine manner (Yamanaka et al., Citation1994). In addition, IL-1β induces reactive astrocytic proliferation as a response to virus itself and/or viral cytopathic effect in some viral infections, including highly pathogenic avian influenza virus and HIV-1 (Wang et al., Citation2008; Xing et al., Citation2009). However, there are limited manifestations on ALV-A infection, although TGF-β2 expression has been reported to be up-regulated in the serum of infected chickens (Barbour et al., Citation1999). Sp-53 had higher replication ability in cultured astrocytes and induced excessive release of IL-1β from these cells, suggesting that IL-1β was produced by the astrocyte response to viral infection. Furthermore, ALV-A has the simplest genome structure without any accessory genes and shows no cytopathic effects in the infected cells. ALVs showing high replication ability in chicken astrocytes could therefore induce the glial cells to release IL-1β, which affects astrocytic growth through an autocrine/paracrine signal pathway.
TGF-β is a multipotent cytokine that can regulate cell growth, differentiation, apoptosis and development (ten Dijke & Hill, Citation2004; Rahimi & Leof, Citation2007). TGF-β promotes the proliferation of fibroblasts, whereas it inhibits the proliferation of blood cells and epithelial cells (Jakowlew, Citation2006; Hill et al., Citation2009). Although the effects of TGF-β on astrocytic growth are still controversial, TGF-β could promote or inhibit the proliferation of astrocytes in several neoplastic diseases (Massagué, Citation2008; Tiwari et al., Citation2011). TGF-β can also induce self-renewal and oncogenic capability of one glioma cell line, LN-229, in cooperation with IL-1β, although the detailed molecular mechanism of the phenomenon is not clear. The intracerebral up-regulation of TGF-β in chickens inoculated with 5 × 104 TCIU Sp-53 thus implies that TGF-β as well as IL-1β may contribute to severe astrocytic proliferation in this group.
The long terminal repeat domain of ALVs, which is formed by integration of viral cDNA in the S phase of mitosis, performs a crucial role to induce avian lymphoid leukosis. When the provirus is eventually inserted upstream of cellular oncogenes, the long terminal repeat domain activates transcription of these genes, resulting in tumour formation (promoter insertion). Transformation of host cells usually takes more than 5 months in lymphoid leukosis. Although there is currently no reliable method to distinguish between reactive and neoplastic astrocytes in fowl glioma, the oncogenesis theory cannot explain the pathogenesis of fowl glioma because the gliomatous lesions are detected 35 to 70 days after in ovo infection (Nakamura et al., Citation2011).
RCAS(A) virus generally replicates at high titres in DF-1 cells (Hughes, Citation2004), but a very low level of viral RNA was detected in infected DF-1 cells in this experiment. We recognized that there were a few insertions and mutations of the RCAS(A) vector we used in this experiment compared with the original RCAS(A) vector. These mutations and insertions may affect replication efficiency of this strain. However, we confirmed that the RCAS(A) we used at this time could not induce glioma in chickens at 70 and 140 days of age (Nakamura et al., Citation2011).
In conclusion, our results demonstrate that astrocytic growth at least in the early phase of fowl glioma-inducing ALV infection could be promoted by autocrine/paracrine IL-1β production by astrocytes.
Acknowledgement
The authors would like to thank Dr Stephen H. Hughes of the National Cancer Institute, USA, for donating avian retrovirus vector RCAS(A).
Funding
This research was supported by the Ministry of Education, Culture, Sports, Science and Technology of Japan under a Grant-in-Aid for Scientific Research [grant number B22380162] and a Grant-in-Aid for Challenging Exploratory Research [grant number 23658249], and a Research Fellowship of the Japan Society for the Promotion of Science for Young Scientists [grant number 2172].
Additional information
Funding
References
- Adams, S.C., Xing, Z., Li, J. & Cardona, C.J. (2009). Immune-related gene expression in response to H11N9 low pathogenic avian influenza virus infection in chicken and Pekin duck peripheral blood mononuclear cells. Molecular Immunology, 46, 1744–1749.10.1016/j.molimm.2009.01.025
- Barbour, E.K., Bouljihad, M., Hamdar, B., Sakr, W., Eid, A. & Safieh-Garabedian, B. (1999). Dynamics of protein 27 of avian leukosis virus and transforming growth factor beta2 in lymphoid leukosis susceptible and resistant broiler chicken breeding stock. Veterinary Research Communications, 23, 191–200.10.1023/A:1006233818946
- Belmonte, V. (1935). Über ein glioma beim haushuhn. Virchows archive für Pathologische Anatomie and Physiologie und für Klinische Madizin [About a glioma in domestic fowls], 294, 329–333.
- Biering-Sørensen, U. (1956). On disseminated, focal gliomatosis (“multiple gliomas”) and cerebral calcification in hens: a study of pathogenesis. Nordisk Veterinaer Medicin, 8, 887–901.
- Bowles, N.E., Eisensmith, R.C., Mohuiddin, R., Pyron, M. & Woo, S.L.C. (1996). A simple and efficient method for concentration and purification of recombinant retrovirus for increased hepatocyte transduction in vivo. Human Gene Therapy, 7, 1735–1742.10.1089/hum.1996.7.14-1735
- Coyle-Rink, J., Sweet, T.M., Abraham, S., Sawaya, B.E. Batuman, O., Khalili, K. & Amini, S. (2002). Interaction between TGFß signaling proteins and C/EBP controls basal and Tat-mediated transcription of HIV-1 LTR in astrocytes. Virology, 299, 240–247.10.1006/viro.2002.1439
- Dang, Q., Whitted, S., Goeken, R.M., Brenchley, J.M., Matsuda, K., Brown, C.R., Lafont, B.A.P., Starost, M.F., Iyengar, R., Plishka, R.J., Buckler-White, A. & Hirsch, M.V. (2012). Development of neurological disease is associated with increased immune activation in simian immunodeficiency virus-infected macaques. Journal of Virology, 86, 13795–13799.10.1128/JVI.02174-12
- Hatai, H., Ochiai, K., Murakami, M., Imanishi, S., Tomioka, Y., Toyoda, T., Ohashi, K. & Umemura, T. (2008). Prevalence of fowl glioma-inducing virus in chickens of zoological gardens in Japan and nucleotide variation in the env gene. Journal of Veterinary Medical Science, 70, 469–474.10.1292/jvms.70.469
- Hatai, H., Ochiai, K., Tomioka, Y., Toyoda, T., Hayashi, K., Anada, M., Kato, M., Toda, A., Ohashi, K., Ono, E., Kimura, T. & Umemura, T. (2005). Nested polymerase chain reaction for detection of the avian leukosis virus causing so-called fowl glioma. Avian Pathology, 34, 473–479.10.1080/03079450500368086
- Hill, J.J., Tremblay, T., Cantin, C., O'Connor-McCourt, M., Kelly, J.F. & Lenferink, A.E.G. (2009). Glycoproteomic analysis of two mouse mammary cell lines during transforming growth factor (TGF)-β induced epithelial to mesenchymal transition. Proteome Science, 7, 2.
- Hughes, H.S. (2004). The RCAS vector system. Folia Biologica, 50, 107–119.
- Iwata, N., Ochiai, K., Hayashi, K., Ohashi, K. & Umemura, T. (2002). Avian retrovirus infection causes naturally occurring glioma: isolation and transmission of a virus from so-called fowl glioma. Avian Pathology, 31, 193–199.10.1080/03079450120118702
- Jackson, C. (1954). Glioma of the domestic fowls. Onderstepoort Journal of Veterinary Research, 26, 501–597.
- Jakowlew, S.B. (2006). Transforming growth factor-ß in cancer and metastasis. Cancer and Metastasis Reviews, 25, 435–457.10.1007/s10555-006-9006-2
- Jungherr, E. & Wolf, A. (1939). Gliomas in animals. American Journal of Cancer, 37, 493–509.
- Kaiser, P., Rothwell, L., Galyov, E.E., Barrow, P.A., Burnside, J. & Wigley, P. (2000). Differential cytokine expression in avian cells in response to invasion by Salmonella typhimurium, Salmonella enteritidis and Salmonella gallinarum. Microbiology, 146, 3217–3226.
- Massagué, J. (2008). TGFß in Cancer. Cell, 134, 215–230.10.1016/j.cell.2008.07.001
- Matsuda, K., Brown, C.R., Foley, B., Goeken, R., Whitted, S., Dang, Q., Wu, F., Plishka, R., Buckler-White, A. & Hirsch, V.M. (2013). Laser capture microdissection assessment of virus compartmentalization in the central nervous systems of macaques infected with neurovirulent simian immunodeficiency virus. Journal of Virology, 87, 8896–8908.10.1128/JVI.00874-13
- Merrill, J.E. & Chen, I.S. (1991). HIV-1, macrophages, glial cells, and cytokines in AIDS nervous system disease. The FASEB Journal, 5, 2391–2397.
- Nakamura, S., Ochiai, K., Hatai, H., Ochi, A., Sunden, Y. & Umemura, T. (2011). Pathogenicity of avian leukosis viruses related to fowl glioma-inducing virus. Avian Pathology, 40, 499–505.10.1080/03079457.2011.605783
- Ochi, A., Ochiai, K., Kobara, A., Nakamura, S., Hatai, H., Handharyani, E., Tiemann, I., Tanaka 3rd, B.I., Toyoda, T., Abe, A., Seok, S., Sunden, Y., Torralba, C.N., Park, J., Hafez, M.H. & Umemura, T. (2012). Epidemiological study of fowl glioma-inducing virus in chickens in Asia and Germany. Avian Pathology, 41, 299–309.10.1080/03079457.2012.684373
- Ochiai, K., Ohashi, K., Mukai, T., Kimura, T., Umemura, T. & Itakura, C. (1999). Evidence of neoplastic nature and viral aetiology of so-called fowl glioma. The Veterinary Record, 145, 79–81.10.1136/vr.145.3.79
- Parvizi, P., Abdul-Careem, M.F., Haq, K., Thanthrige-Don, N., Schat, K.A. & Sharif, S. (2010). Immune responses against Marek's disease virus. Animal Health Research Reviews, 11, 123–134.10.1017/S1466252310000022
- Peters, J.L., Cassone, V.M. & Zoran, M.J. (2005). Melatonin modulates intercellular communication among cultured chick astrocytes. Brain Research, 1031, 10–19.10.1016/j.brainres.2004.09.064
- Rahimi, R.A. & Leof, E.B. (2007). TGF-ß signaling: a tale of two responses. Journal of Cellular Biochemistry, 102, 593–608.10.1002/jcb.21501
- Reece, R.L. (2008). Other tumors of unknown etiology. In Y.M. Saif, S. Hafner, A.M. Fadly, J.R. Glisson, L.R. McDougald, L.K. Nolan, & D.E. Swayne (Eds.). Diseases of Poultry 12th edn (pp. 593–616). Ames: Iowa State Press.
- Sharma, V., Dixit, D., Koul, N., Mehta, V.S. & Sen, E. (2011). Ras regulates interleukin-1ß-induced HIF-1α transcriptional activity in glioblastoma. Journal of Molecular Medicine, 89, 123–136.10.1007/s00109-010-0683-5
- Shini, S., Shini, A. & Kaiser, P. (2010). Cytokine and chemokine gene expression profiles in heterophils from chickens treated with corticosterone. Stress, 13, 185–194.10.3109/10253890903144639
- Sen, E. (2011). Targeting inflammation-induced transcription factor activation: an open frontier for glioma therapy. Drug Discovery Today, 16, 1044–1051.10.1016/j.drudis.2011.09.003
- Summers, B.A., Cummings, J.F. & de Lahunta, A. (1994). Tumors of the central nervous system. In L. Dunkan (Ed.). Veterinary Neuropathology (pp. 351–401). St Louis: Mosby-Year Book.
- Swayne, D.E., Fletcher, O.J., Abdul-Aziz, T., Shivaprasad, H.L. & Barnes, H.L. (2008). Nervous system. In O.L. Fletcher & T. Abdul-Aziz (Eds.). Avian Histopathology 3rd edn (pp. 260–291). Jacksonville: American Association of Avian Pathologists.
- Ten Dijke, P. & Hill, C.S. (2004). New insights into TGF-beta-Smad signaling. Trends in Biochemical Sciences, 29, 265–273.10.1016/j.tibs.2004.03.008
- Tiwari, R., Bargmann, W. & Bose, H.R., Jr. (2011). Activation of the TGF-ß/Smad signaling pathway in oncogenic transformation by v-Rel. Virology, 413, 60–71.10.1016/j.virol.2011.02.002
- Tomioka, Y., Ochiai, K., Ohashi, K., Kimura, T. & Umemura, T. (2003). In ovo infection with an avian leukosis virus causing fowl glioma: viral distribution and pathogenesis. Avian Pathology, 32, 617–624.10.1080/03079450310001610640
- Tomioka, Y, Ochiai, K., Ohashi, K., Ono, E., Toyoda, T., Kimura, T. & Umemura, T. (2004). Genome sequence analysis of the avian retrovirus causing so-called fowl glioma and the promoter activity of the long terminal repeat. Journal of General Virology, 85, 647–652.10.1099/vir.0.79778-0
- Wang, G., Zhang, J., Li, W., Xin, G., Su, Y., Gao, Y., Zhang, H., Lin, G., Jiao, X. & Li, K. (2008). Apoptosis and proinflammatory cytokine responses of primary mouse microglia and astrocytes induced by human H1N1 and avian H5N1 influenza viruses. Cellular & Molecular Immunology, 5, 113–120.10.1038/cmi.2008.14
- Wight, P.A.L. & Duff, R.H. (1964). The histopathology of epizootic gliosis and astrocytoma of the domestic fowl. Journal of Comparative Pathology, 74, 373–380.10.1016/S0368-1742(64)80043-6
- Xing, H.Q., Hayakawa, H., Izumo, K., Kubota, R., Gelpi, E., Budka, H. & Izumo,S. (2009). In vivo expression of proinflammatory cytokines in HIV encephalitis: an analysis of 11 autopsy cases. Neuropathology, 29, 433–442.10.1111/j.1440-1789.2008.00996.x
- Yamanaka, R., Tanaka, R., Saitoh, T. & Okoshi, S. (1994). Cytokine gene expression on glioma cell lines and specimens. Journal of Neuro-oncology, 21, 243–247.10.1007/BF01063773
- Yeung, Y.T., McDonald, K.L., Grewal, T. & Munoz, L. (2013). Interleukins in glioblastoma pathophysiology: implications for therapy. British Journal of Pharmacology, 168, 591–606.10.1111/bph.12008
- Zink, M.C., Suryanarayana, K., Mankowski, J.L., Shen, A., Piatak Jr., M., Spelman, J.P., Carter, D.L., Adams, R.J., Lifson, J.D. & Clements, J.E. (1999). High viral load in the cerebrospinal fluid and brain correlates with severity of simian immunodeficiency virus encephalitis. Journal of Virology, 73, 10480–10488.