Abstract
Gallibacterium anatis is a major cause of reproductive tract infections in chickens. Here, we aimed to evaluate the efficacy of the recombinant protein GtxA-N at protecting hens, by addressing three objectives; (i) evaluating the antibody response following immunization (ii) scoring and comparing lesions, following challenge with G. anatis, in immunized and non-immunized hens and (iii) investigating if the anti-GtxA-N antibody titre in individual hens correlated with the observed lesions. Two consecutive experiments were performed in hens. In the first experiment hens were immunized with GtxA-N on day 0 and day 14, infected with G. anatis on day 28 and euthanized on day 56. The GtxA-N antibody response was assessed in pooled serum samples throughout the experiment, using an indirect enzyme-linked immunosorbent assay (ELISA). In the second experiment the GtxA-N antibody titres were assessed in individual hens before and after immunization. Subsequently, the hens were inoculated with G. anatis and finally all hens where euthanized and submitted for post mortem examination 48 h after inoculation. Immunization elicited strong antibody responses that lasted at least 8 weeks (P < .0001). The individual antibody titres observed in response to immunization varied considerably among hens (range: 174,100–281,500). Lesion scores following G. anatis infection were significantly lower in immunized hens compared to non-immunized hens (P = .004). Within the immunized group, no correlation was found between the individual antibody titres and the lesion scores. This study clearly demonstrated GtxA-N as a vaccine antigen able of inducing protective immunity against G. anatis.
Introduction
Gallibacterium anatis is a Gram-negative bacterium belonging to the Pasteurellaceae family (Christensen et al., Citation2003). It constitutes a part of the normal microflora of the upper respiratory tract and lower genital tract in both layer and broiler chickens (Bisgaard, Citation1977; Mushin et al., Citation1980; Bojesen et al., Citation2003a). G. anatis infections cause decreased egg production and increased mortality, leading to decreased animal welfare and an economic burden in poultry production (Bisgaard, Citation1977; Mushin et al., Citation1980; Shaw et al., Citation1990; Neubauer et al., Citation2009). Notable phenotypic and genotypic diversity has been observed among G. anatis strains (Bojesen et al., Citation2003b; Bisgaard et al., Citation2009; Johnson et al., Citation2013). This diversity poses a challenge for the development of a universal vaccine that protects against a wide selection of strains. The so-called pan-genomic reverse vaccinology approach has shown promise at identifying antigens that are highly conserved amongst isolates of a bacterial species and, thus, is a method to account for antigenic diversity when developing vaccines (Serruto et al., Citation2009). The pan-genomic reverse vaccinology approach was recently applied on 10 highly diverse G. anatis strains aiming at identifying potential immunogens with a high protective potential against G. anatis. Among all the recombinant proteins tested, the N-terminal part of the Gallibacterium toxin A (GtxA) showed the highest antibody titres in sera from chickens infected with G. anatis (Bager et al., Citation2014). GtxA is a well conserved RTX (repeats in toxin)-toxin produced by G. anatis. RTX-toxins are a group of secreted toxins found in Gram-negative bacteria (Frey & Kuhnert, Citation2002). GtxA has leukotoxic and haemolytic activity, which makes it an important virulence factor for G. anatis, and the full toxin activity is dependent on the N-terminal (Kristensen et al., Citation2010, Citation2011).
The efficacy of a vaccine relates to its ability to induce a broadly protective and long-lasting immune response, including the production of antigen-specific antibodies (Sallusto et al., Citation2010). Previous studies have shown that a number of factors can influence the immune response to vaccination, including size of the individual being vaccinated, age, gender, species, breed and genetics (Dick & Avakian, Citation1991; Bertelsen et al., Citation2007; Kennedy et al., Citation2007; Kaiser, Citation2010; Chathuranga et al., Citation2013). Thus, the antibody response following vaccination can vary greatly among individuals (Dick & Avakian, Citation1991; Kennedy et al., Citation2007; Vratskikh et al., Citation2013).
In the present investigation we wanted to evaluate the immunogenic and protective efficacy of immunization with the recombinant protein GtxA-N in hens. In the first experiment we monitored the GtxA-N specific antibody response to immunization and subsequent challenge with G. anatis, over a period of 8 weeks. In the second experiment we evaluated the protective ability of GtxA-N, by investigating whether immunization of hens using recombinant GtxA-N offered protection against macroscopic lesions induced by an experimental G. anatis challenge, when compared to a non-immunized control group. In the second experiment we also determined the magnitude of variation in the individual antibody titres in the immunized hens, and we investigated if a correlation could be established between the individual antibody titres and the corresponding lesion scores.
Materials and Methods
Experimental animals and housing facilities
Apparently healthy Lohmann Brown layer hens were purchased at a commercial free-range farm with no history of clinical disease. All birds were adult and in lay. Due to practical reasons the birds in the first experiment were 36 weeks old and the birds in the second experiment were 22 weeks old. After arrival the hens were allowed one week to acclimatize. During the trial all hens were housed in free-range pens with access to ad libitum commercial feed for egg-laying hens, water and nesting material. All hens were swabbed in the cloaca. The swabs were cultivated on brain heart infusion agar with 5% citrated bovine blood in sealed plastic bags at 37°C overnight to test for the presence of G. anatis prior to initiation of the experiment. G. anatis isolates were identified based on colony morphology as described by Christensen et al. (Citation2003). The trials were approved by the Danish National Animal Ethics Committee (Approval no. 2012-15-2934-00339 and 2012-15-2934-00923).
Immunization of hens with recombinant GtxA-N
In the first experiment immunization was performed on 14 hens using the recombinant protein GtxA-N. The recombinant protein had previously been purified as described by Bager et al. (Citation2014). The recombinant GtxA-N was diluted in a 1:1 solution of protein buffer (50 mM NaP, 150 mM NaCl, 0.5 mM TCEP, 10% glycerol; pH 7.5) and Freund's incomplete adjuvant (Sigma-Aldrich). The immunization dose was 50 µg of protein per hen for the first immunization (Day 0), and 100 µg of protein per hen for the second immunization (Day 14). The hens were immunized by a subcutaneous injection in the neck. The control group of 13 hens received a 1:1 solution of protein buffer and Freund's incomplete adjuvant only. To monitor the antibody titre throughout the experiment blood samples were collected from the brachial vein of each hen using a sterile needle and syringe. Sample were collected on Day 0, 14, 28 (before the experimental infection), 42 (14 days post infection (p.i.)) and 56 (28 days p.i.).
In the second experiment 19 hens were immunized on Day 0 and Day 14 by a subcutaneous injection as described in the first experiment. The immunization dose was 100 µg of protein per hen. A non-immunized control group of 14 hens was included, two of which were used as uninfected controls. Blood samples were collected from each hen on Day 0 (before the immunization) and on Day 35 (before the experimental infection).
Experimental infection of hens
G. anatis biovar haemolytica strain 12656-12 was used for the experimental infections as previously described by Bojesen et al. (Citation2004). In the first experiment the immunized group and the control group were infected intraperitoneally (i.p.) with 108 CFU of G. anatis on Day 28 (day 14 following the second immunization). Subsequently, the hens were kept for a period of 4 weeks, during which serum samples were collected twice (Day 42 and 56). In the second experiment individual lesion scores were obtained following infection with G. anatis to evaluate the protective effect of immunization. The immunized group and the control group were infected with an i.p. injection of 108 CFU of G. anatis. Of the 14 non-immunized hens in the control group, two were used as uninfected controls. The hens were euthanized 48 h p.i. in the acute phase of the infection and macroscopic lesions in the peritoneum, ovaries and salpinx were recorded and scored as previously described by Bager et al. (Citation2014). SE Pors and AM Bojesen did the scoring of the lesions independently. After the separate scoring a final score was then adjusted when different scoring occurred. Scores were given according to severity, with a minimum score of 0 and a maximum score of 2–5 depending on the parameter (). A total lesion score for each hen was calculated as a sum of the scores in the peritoneum, ovaries and salpinx. The total lesion scores, in the immunized and the non-immunized group, were compared using the Mann–Whitney test, and a P-value < .05 was considered significant. To identify a possible correlation, within the immunized group, between the individual antibody titres after immunization and the corresponding total lesion scores, a Spearman rank correlation coefficient was calculated. Furthermore, all hens were swabbed from the peritoneum, ovaries and salpinx using a sterile cotton swab. The swabs were plated on brain heart infusion agar supplemented with 5% bovine blood and cultivated overnight at 37°C in sealed plastic bags. Gallibacterium isolates were identified based on colony morphology as described by Bojesen et al. (Citation2003a).
Table 1. Scoring system for evaluating macroscopic lesions in peritoneum, ovaries and salpinx in hens following experimental infection with G. anatis. Parameters scored and explanation of each score within all parameters.
Enzyme-linked immunosorbent assay (ELISA)
To evaluate the antibody response in the serum samples, all serum samples were tested against the recombinant protein GtxA-N using an indirect ELISAs as described by Bager et al. (Citation2014). The only minor adjustment to the previous protocol was that serum dilution rows were started at 1:300 or 1:900. In the first experiment the antibody response was assessed in pooled serum samples in both groups. In the second experiment the antibody response was assessed in individual serum samples in the immunized group, and in pooled serum samples in the control group. The antibody titres were calculated based on the optical density and using linear regression as described by Bager et al. (Citation2014) using “Myassays”, an analysis software solution available at URL http://www.myassays.com/antibody-titers.assay. All serum samples were analysed in triplicate and a mean antibody titre and a standard deviation (SD) was calculated based on these triplicates. The antibody titres were compared between two sampling points (dates) using the Mann–Whitney test and between several sampling points using the Kruskal–Wallis test. The difference between the antibody titres was considered significant at a P-value <.05.
Results
Response to immunization and i.p. infection
To test for colonization with G. anatis prior to initiation of the experiments, all hens were swabbed from the cloaca. As expected, the hens were positive for G. anatis in the cloacal mucosa. To evaluate the immune response to recombinant protein GtxA-N in the first experiment, antibody titres were calculated using pooled serum samples obtained chronologically following immunization with GtxA-N and i.p. infection with G. anatis (). The first immunization with GtxA-N resulted in a rise in the antibody titre at Day 14. Another rise was observed after the second immunization, resulting in a peak antibody titre at Day 28. Although the antibody titre declined on Days 42 and 56, the titre remained well above (35 fold) the level observed before immunization at Day 0 (P < .0001). The antibody titres observed in the non-immunized control group are also illustrated in . The antibody titre against GtxA-N remained stable and low until day 42 (14 days after the i.p. infection), where a rise in the antibody titre was observed (P = .04), indicating that GtxA-N was expressed in vivo during infection. In relation to the challenge the birds became depressed and reluctant to move. Within 6 h the birds in the immunization group no longer appeared clinically affected, while the birds in the non-immunized group remained reluctant to move. Within 48 h none of the birds appeared clinically affected.
Figure 1. Mean antibody titres (±SD) against GtxA-N assessed by indirect ELISA in pooled serum samples from 14 immunized hens and 13 non-immunized hens. The hens were immunized with recombinant protein GtxA-N and Freund's incomplete adjuvant on Day 0 and 14. The non-immunized hens received a GtxA-N-free buffer and Freund's incomplete adjuvant. An i.p. infection with 108 CFU G. anatis was performed on Day 28.
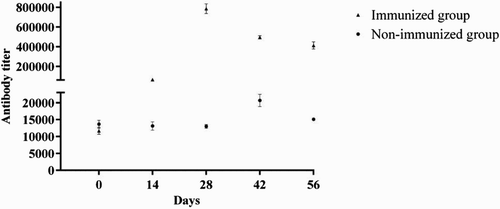
Necropsy findings
To evaluate the protective efficacy of immunization with GtxA-N in the second experiment, total lesion scores were obtained 48 hours after i.p. infection with G. anatis in hens immunized with GtxA-N and in non-immunized hens. Two hens from the non-immunized control group were used as uninfected controls and no lesions were observed in these hens. Lesion scores from the hens are summarized in . Briefly, 48 h p.i. lesions found in the infected groups were local to diffuse peritonitis with varying amounts of purulent material. Vascular engorgement of the ovary, salpinx and peritoneum was also found. In some cases purulent oophoritis and regression of the follicles were observed. In the immunized group the mean lesion score for each organ was 5.16 for peritoneum, 1.74 for the ovaries and 1.37 for salpinx, while the mean lesion score for each organ in the non-immunized group was 8.33 for peritoneum, 3.58 for the ovaries and 3.67 for salpinx, giving the immunized group the lowest mean lesion score in all organs. The i.p. infection resulted in a clear difference in total lesion scores when comparing the immunized and non-immunized hens (P = .0004), with the immunized hens having the least lesions (the mean total lesion scores were 8.21 and 15.58 in the immunized and non-immunized group, respectively). Gallibacterium isolates were cultivated from the organs of 13 out of 19 hens in the immunization group and from all infected hens in the non-immunized group. No other bacterial species were isolated.
Figure 2. Individual macroscopic lesion scores obtained 48 h after i.p. infection with G. anatis in hens. The following parameters were scored; in the peritoneum (i) inflammation (ii) amount of exudate and (iii) transparency of peritoneum, in the ovaries (i) increased vascularization (ii) purulent exudate and (iii) regression of follicles, and in the salpinx (i) increased vascularization and (ii) purulent exudate. Scores were given according to severity, with a minimum score of 0 and a maximum score of 2–5 depending on the parameter. The total lesion score is the sum of all scores given to a hen.
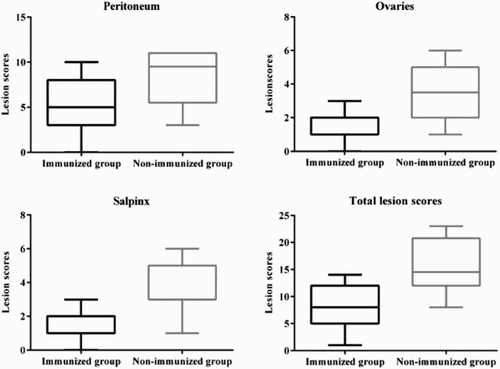
Individual antibody titres and corresponding lesion scores
To search for a correlation between immune response and level of protection in the second experiment, individual antibody titres for the group of hens immunized with GtxA-N and corresponding total lesion scores following i.p. infection with G. anatis were obtained (). A significant rise in the antibody titres in response to immunization was observed (P < .0001). In the non-immunized control group, the antibody titres in the pooled serum samples remained low throughout the experiment (Day 0: 7491; Day 35: 6038). The individual antibody titres following immunization with GtxA-N were varying among the hens (range: 174,100–281,500,000, median: 575,967). The corresponding total lesion scores following i.p. infection with G. anatis ranged from 1 to 14. No correlation could be found between the individual antibody titres following immunization and the corresponding total lesion scores (the Spearman correlation coefficient: −0.34, P-value: .08).
Table 2. Antibody titres and corresponding total lesion scores in hens immunized with recombinant protein GtxA-N.
Discussion
The present study demonstrated that the recombinant protein Gtx-A is highly immunogenic in chickens and able to induce protective immunity against lesions caused by G. anatis. Infections with G. anatis can cause macroscopic lesions in several tissues and organs, including the peritoneum, reproductive tract and respiratory tract (Hacking & Pettit, Citation1974; Shaw et al., Citation1990; Bojesen et al., Citation2004; Neubauer et al., Citation2009). This was also the case in the present study, where i.p. infection with G. anatis resulted in macroscopic lesions in the peritoneum, ovaries and salpinx in the infected hens. Immunization with the recombinant protein GtxA-N, however, resulted in fewer and less severe lesions following i.p. infection with G. anatis, when compared to infection in the non-immunized control group. The ability of recombinant GtxA-N to provide protective immunity against lesions has not previously been described. The use of lesion scores for evaluating protection is of great relevance for G. anatis, as clinical symptom following infection can be subtle and, of nature, hard to quantify (Bojesen et al., Citation2004). Several factors clearly indicate that the lesions observed in the current study were in fact caused by G. anatis. First, lesions were found in target tissues for G. anatis and the lesions were those typical of an acute purulent infection which has previously been observed following experimental infection with G. anatis (Bojesen et al., Citation2004). Secondly, G. anatis was isolated from the affected organs and no other bacterial species were isolated. Thirdly, no lesions were observed in the uninfected controls. Finally, the significant protection against lesion in the GtxA-N immunization group clearly indicates that the lesions were in fact caused by the harmful effects of the GtxA toxin secreted from thereby further demonstrating the importance of GtxA as a virulence factor. Immunization with other RTX-toxins has previously been investigated and proven effective. The RTX-toxins ApxI and ApxII from Actinobacillus pleuropneumonia offered protection against lesions in swine (Haga et al., Citation1997). Thus, the finding that GtxA-N offers protection against macroscopic lesions further supports the use of RTX-toxins in vaccines.
In the present study, the hens were found to be positive for G. anatis in the cloacal mucosa and antibody titres were observed against GtxA-N prior to immunization, indicating that the hens had already been exposed to the antigen, and correlating with a previous study were pre-immune antibody titres against GtxA-N were also observed (Bager et al., Citation2014). The use of hens that are already colonized with Gallibacterium reflects field conditions where G. anatis is a normal part of the microflora of the lower reproductive tract (Bojesen et al., Citation2003a). Thus, using Gallibacterium free layers for immunization would not resemble field conditions. Evaluating immunization in colonized birds from a commercial farm is of great relevance, as these are the target species in which a future vaccine would be applied. Since the pre-immune antibody titres in the current study were very low compared to the post-immune titres (39-fold lower than the post-immune titre (Day 56) in the first experiment), the colonization with G. anatis was not considered to influence the effect of immunization notably. The extremely high immunogenicity of GtxA-N in the current study correlates well with a previous study where GtxA-N elicited a high antibody response, when tested against sera form hens infected with G. anatis (Bager et al., Citation2014). In the light of this, the N-terminal part of GtxA appears particularly promising as a vaccine candidate.
The antibody titre observed in response to immunization with GtxA-N varied considerably among the 19 hens included in the current study. A variation in the antibody response to immunization among individuals or groups of individuals has been observed in several species, including avian species (Dick & Avakian, Citation1991; Bertelsen et al., Citation2007; Kennedy et al., Citation2007; Chathuranga et al., Citation2013; Vratskikh et al., Citation2013). In chickens, it has been demonstrated that the age of chickens can influence the magnitude of the antibody response to immunization. In 1–11 week old chickens, a positive correlation was found between the age and the antibody response to immunization with a fowl cholera vaccine (Dick & Avakian, Citation1991). However, in the present study the 19 hens were all of the same age (22 weeks). Thus, age cannot explain the observed variation in the antibody response. Both the innate and the adaptive immune response are influenced by genetics, and a difference in susceptibility to pathogens has been observed in different lines of chickens within the same breed (Ferro et al., Citation2004; Kaiser, Citation2010). The genetic background for increased immune resistance in chickens is complex and likely multi-factorial (Kaiser, Citation2010), and it is likely that genetics, to some extent, contributed to the observed variance in the antibody response in the current study. The results from the current study indicate that a variation in the antibody response to immunization in chickens should be expected, even when the birds are of the same age and breed.
The importance of a variation in the antibody response depends on how it affects the level of protection. Antibodies are needed for recognition, opsonization and toxin neutralization in the fight against foreign pathogens (Warrington et al., Citation2011). Thus, it is natural to assume that a higher level of antibodies should lead to a better protection. In the current study, a higher level of antibodies did in fact lead to better protection when comparing the immunized group to the non-immunized group. However, when evaluating antibody titres within the immunized group, the magnitude of the individual antibody response in the immunized hens did not correlate with the level of protection against macroscopic lesions. In contrast, previous studies have shown a clear correlation between the serum concentration of antibodies following vaccination and the level of protection against the toxin producing bacteria Bacillus anthracis, Clostridium tetani and Corynebacterium diphtheriae (Newell et al., Citation1971; Little et al., Citation2004; Plotkin, Citation2010). In the current study, an indirect ELISA was used to assess the antibody response. Both indirect ELISA and toxin neutralization assays have been used when identifying a correlation between the antibody response and the level of protection against infections (Cherry et al., Citation1998; Little et al., Citation2004; Plotkin, Citation2010). While indirect ELISA simply quantifies all antibodies that are able to bind to a specific antigen, the toxin neutralization assay quantifies antibodies that are considered functional by neutralizing a toxin only (Ngundi et al., Citation2010). It is possible that only a fraction of the antibodies produced in response to the GtxA-N immunization were functional in neutralizing the GtxA toxin, and that the remaining non-functional antibodies account for the observed variation in the antibody titres. For future studies, it could be relevant to perform a toxin neutralization assay. For many vaccines, it is possible to identify a specific level of antibodies in serum that is highly correlated with protection, that is, once a certain threshold antibody level is reached a protection against infection is observed (Plotkin, Citation2010). Based on this, it is possible that the lack of correlation between the antibody titres and protection within the immunized group in the current study was due to the fact that the antibody titres of immunized hens were all above the protective threshold. By immunizing groups of hens with lower doses of GtxA-N, it might be possible to identify an antibody titre threshold, where the protection against lesions drops significantly.
In conclusion, we demonstrated that; (i) that the recombinant protein GtxA-N was highly immunogenic in hens when administered subcutaneously and capable of eliciting an antibody response that lasted at least 8 weeks, (ii) that immunization with GtxA-N induced an antibody response of variable magnitude among individual hens, (iii) that the current immunization protocol did not induce a correlation between the individual antibody response and the severity of macroscopic lesions after challenge, and (iv) that macroscopic lesions scores following i.p. infection with G. anatis were significantly lower in immunized hens compared to non-immunized hens, indicating that immunization with GtxA-N provided protection against lesions caused by G. anatis. Hence, GtxA-N induced protective immunity against lesions caused by G. anatis, independently of the individual antibody titres, suggesting a great potential of the antigen as a vaccine candidate against infections caused by G. anatis.
Acknowledgements
We would like to thank Karin Kold and Annett Voetmann Christoffersen for their great help with the practical part of this project.
Funding
This work was supported by the Faculty of Health and Medical Sciences, University of Copenhagen.
References
- Bager, R.J., Kurdirkiene, E., da Piedade, I., Seemann, T., Nielsen, T.K., Pors, S.E., Mattsson, A.H., Boyce, J.D., Adler, B. & Bojesen, A.M. (2014). In silico prediction of Gallibacterium anatis pan-immunogens. Veterinary Research, 45, 80.
- Bertelsen, M.F., Klausen, J., Holm, E., Grøndahl, C. & Jørgensen, P.H. (2007). Serological response to vaccination against avian influenza in zoo-birds using an inactivated H5N9 vaccine. Vaccine, 25, 4345–4349.
- Bisgaard, M. (1977). Incidence of Pasteurella haemolytica in the respiratory tract of apparently healthy chickens and chickens with infectious bronchitis. Characterisation of 213 strains. Avian Pathology, 6, 285–292.
- Bisgaard, M., Korczak, B.M., Busse, H., Kuhnert, P., Bojesen, A.M. & Christensen, H. (2009). Classification of the taxon 2 and taxon 3 complex of Bisgaard within Gallibacterium and description of Gallibacterium melopsittaci sp. nov., Gallibacterium trehalosifermentans sp. nov. and Gallibacterium salpingitidis sp. nov. International Journal of Systemic and Evolutionary Microbiology, 59, 735–744.
- Bojesen, A.M., Nielsen, O.L., Christensen, J.P. & Bisgaard, M. (2004). In vivo studies of Gallibacterium anatis infection in chickens. Avian Pathology, 33, 145–152.
- Bojesen, A.M., Nielsen, S.S. & Bisgaard, M. (2003a). Prevalence and transmission of haemolytic Gallibacterium species in chicken production systems with different biosecurity levels. Avian Pathology, 32, 503–510.
- Bojesen, A.M., Torpdahl, M., Christensen, H., Olsen, J.E. & Bisgaard, M. (2003b). Genetic diversity of Gallibacterium anatis isolates from different chicken flocks. Journal of Clinical Microbiology, 41, 2737–2740.
- Chathuranga, L.S., Noordeen, F. & Abeykoon, A.M.S.B. (2013). Immune response to hepatitis B vaccine in a group of health care workers in Sri Lanka. International Journal of Infectious Diseases, 17, 1078–1079.
- Cherry, J.D., Gornbein, J., Heininger, U. & Stehr, K. (1998). A search for serologic correlates of immunity to Bordetella pertussis cough illnesses. Vaccine, 16, 1901–1906.
- Christensen, H., Bisgaard, M., Bojesen, A.M., Mutters, R. & Olsen, J.E. (2003). Genetic relationships among avian isolates classified as Pasteurella haemolytica, `Actinobacillus salpingitidis’ or Pasturella anatis with proposal of Gallibacterium anatis gen. nov., comb. nov. and description of additional genomospecies within Gallibacterium gen. nov. International Journal of Systemic Evolutionary Microbiology, 53, 275–287.
- Dick, J.W. & Avakian, A.P. (1991). Response of broiler chickens to fowl cholera vaccination at 1 to 6 weeks of age. Avian Diseases, 35, 761–766.
- Ferro, P.J., Swaggerty, C.L., Kaiser, P., Pevzner, I.Y. & Kogut, M.H. (2004). Heterophils isolated from chickens resistant to extra-intestinal Salmonella enteritidis infection express higher levels of pro-inflammatory cytokine mRNA following infection than heterophils from susceptible chickens. Epidemiology & Infection, 132, 1029–1037.
- Frey, J. & Kuhnert, P. (2002). RTX toxins in Pasteurellaceae. International Journal of Medical Microbiology, 292, 149–158.
- Hacking, W.C. & Pettit, J.R. (1974). Pasteurella hemolytica in pullets and laying hens. Avian Diseases, 18, 483–486.
- Haga, Y., Ogino, S., Ohashi, S., Ajito, T., Hashimoto, K. & Sawada, T. (1997). Protective efficacy of an affinity-purified hemolysin vaccine against experimental swine pleuropneumonia. Journal of Veterinary Medical Science, 59, 115–120.
- Johnson, J.T., Danzeisen, J.L., Trampel, D., Nolan, L.K., Seeman, T., Bager, R.J. & Bojesen, A.M. (2013). Genome analysis and phylogenetic relatedness of Gallibacterium anatis strains from poultry. PLoS ONE, 8, e54844.
- Kaiser, P. (2010). Advances in avian immunology – prospects for disease control: a review. Avian Pathology, 39, 309–324.
- Kennedy, L.J., Lunt, M., Barnes, A., McElhinney, L., Fooks, A.R., Baxter, D.N. & Ollier, W.E. (2007). Factors influencing the antibody response of dogs vaccinated against rabies. Vaccine, 25, 8500–8507.
- Kristensen, B.M., Frees, D. & Bojesen, A.M. (2010). GtxA from Gallibacterium anatis, a cytolytic RTX-toxin with a novel domain organization. Veterinary Research, 41, 25.
- Kristensen, B.M., Frees, D. & Bojesen, A.M. (2011). Expression and secretion of the RTX-toxin GtxA among members of the genus Gallibacterium. Veterinary Microbiology, 153, 116–123.
- Little, S.F., Ivins, B.E., Fellows, P.F., Pitt, M.L., Norris, S.L. & Andrews, G.P. (2004). Defining a serological correlate of protection in rabbits for a recombinant anthrax vaccine. Vaccine, 22, 422–430.
- Mushin, R., Weisman, Y. & Singer, N. (1980). Pasteurella haemolytica found in the respiratory tract of fowl. Avian Diseases, 24, 162–168.
- Neubauer, C., De Souza-Pilz, M., Bojesen, A.M., Bisgaard, M. & Hess, M. (2009). Tissue distribution of haemolytic Gallibacterium anatis isolates in laying birds with reproductive disorders. Avian Pathology, 38, 1–7.
- Newell, K.W., Leblanc, D.R., Edsall, G., Levine, L., Christensen, H., Montouri, M.H. & Ramirez, N. (1971). The serological assessment of a tetanus toxoid field trial. Bulletin of the World Health Organization, 45, 773–785.
- Ngundi, M.M., Meade, B.D., Lin, T.L., Tang, W.J. & Burns, D.L. (2010). Comparison of three anthrax toxin neutralization assays. Clinical and Vaccine Immunology, 17, 895–903.
- Plotkin, S.A. (2010). Correlates of protection induced by vaccination. Clinical and Vaccine Immunology, 17, 1055–1065.
- Sallusto, F., Lanzavecchia, A., Araki, K. & Ahmed, R. (2010). From vaccines to memory and back. Immunity, 33, 451–463.
- Serruto, D., Serino, L., Masignani, V. & Mariagrazia, P. (2009). Genome-based approaches to develop vaccines against bacterial pathogens. Vaccine, 27, 3245–3250.
- Shaw, D.P., Cook, D.B., Maheswaran, S.K., Lindeman, C.J. & Halvorson, D.A. (1990). Pasteurella haemolytica as a co-pathogen in pullets and laying hens. Avian Diseases, 34, 1005–1008.
- Vratskikh, O., Stiasny, K., Zlatkovic, J., Tsouchnikas, G., Jarmer, J., Karrer, U., Roggendorf, M., Roggendorf, H., Allwinn, R. & Heinz, F.X. (2013). Dissection of antibody specificities induced by yellow fever vaccination. PLoS Pathogen, 9, e1003458.
- Warrington, R., Watson, W., Kim, H.L. & Antonetti, F.R. (2011). An introduction to immunology and immunopathology. Allergy, Asthma and Clinical Immunology, 7, Suppl 1:S1.