ABSTRACT
Ornithobacterium rhinotracheale is a widely distributed rod-shaped Gram-negative bacterium that infects several avian species including chickens and turkeys. It is associated with respiratory signs, growth retardation, mortality, and reduced egg production, thus causing severe economic losses to the poultry industries. In this study, 37 field isolates of O. rhinotracheale, collected from various locations in Hungary between 1997 and 2015, were identified and characterized by the analysis of partial 16S rRNA gene sequences, enterobacterial repetitive intergenic consensus (ERIC)-PCR, and random amplified polymorphic DNA (RAPD) PCR assays with the OPG11, OPH19, and M13 primers. Most of the field isolates were serotype A, one was serotype B, and four were serotype D. One isolate could not be typed with antisera against serotypes A–E. In a phylogenetic analysis of the 16S rRNA sequences, the isolates formed two clusters. Thirteen distinct patterns were identified with ERIC-PCR, and the RAPD assay with the M13 primer assigned the isolates to 10 different patterns. The other two RAPD assays were unsuitable for distinguishing and grouping the isolates. Neither ERIC type nor RAPD pattern correlated with the place or year of isolation. However, the strains isolated from chickens were more heterogeneous on ERIC-PCR than the isolates recovered from turkeys. In this study, ERIC-PCR was the most discriminatory method for investigating the genetic diversity of O. rhinotracheale isolates.
Introduction
Respiratory diseases are one of the most important health-care problems in the global poultry industry. Bacteria are often involved in the aetiology of these diseases, either alone or in combination with other microorganisms (Chin et al., Citation2013). Ornithobacterium rhinotracheale is a widely distributed rod-shaped, Gram-negative bacterium. It is considered a possible causative agent of the respiratory disease complex of birds, which is associated with respiratory signs, growth retardation, mortality, and reduced egg production, thus causing severe economic losses to the poultry industries. The severity of the clinical signs and the mortality rates vary and are influenced by several environmental factors, including high levels of ammonia, inadequate ventilation, extremes of temperature and humidity, high stocking densities, and concurrent infections with other respiratory pathogens, such as Escherichia coli, avian metapneumovirus, infectious bronchitis virus, Newcastle disease virus, Mycoplasma synoviae, Bordetella avium, or Chlamydophila psittaci (Chin et al., Citation2013). O. rhinotracheale infection has been reported in a wide range of avian host species, including the chicken, turkey, duck, goose, pigeon, guinea fowl, quail, ostrich, gull, partridge, pheasant, and rook (Hafez, Citation2002). It was first described by Charlton et al. (Citation1993) and further characterized by Vandamme et al. (Citation1994). Retrospective studies have shown that it was first isolated from five-week-old turkeys in Germany in 1981 (Hinz & Hafez, Citation1997). The first outbreak in Hungary in 1994 was described by Tanyi et al. (Citation1995) and the strains isolated were subsequently characterized by Varga et al. (Citation2001).
The relatively rapid worldwide spread of the bacterium has prompted a number of epidemiological studies, which entailed the detection of the intraspecies variability among O. rhinotracheale isolates. Eighteen different serotypes have been identified to date (A–R) using agar gel precipitation (AGP) or enzyme-linked immunosorbent assays (ELISAs) (Hafez & Sting, Citation1999). Approximately 97% of isolates belong to four main serotypes: A, B, D, and E (Hafez, Citation2002). However, the limited availability of antisera and the frequent cross-reactions between serotypes (van Empel et al., Citation1997) make ELISA and AGP somewhat inefficient. The limitations of typing based on phenotypic characteristics have encouraged the development of genotyping techniques in this field.
The analysis of 16S rRNA gene sequences is routinely used to differentiate organisms at the genus level across all major phyla of bacteria. The sequence variability in this region often allows comparison to be made at the species and subspecies levels (Clarridge, Citation2004). Previous studies have showed high levels of similarity (98–100%) in this gene among O. rhinotracheale isolates (Amonsin et al., Citation1997; Tsai & Huang, Citation2006).
Random amplified polymorphic DNA (RAPD) assays and repetitive extragenic palindromic PCR (rep-PCR), including enterobacterial repetitive intergenic consensus (ERIC) sequences, have been successfully used for genotyping several bacterial species (Olive & Bean, Citation1999), including O. rhinotracheale (Amonsin et al., Citation1997; Koga & Zavaleta, Citation2005; Waldow, Citation2009).
In this study, we examined the intraspecies genetic diversity of O. rhinotracheale isolates collected in Hungary using a partial 16S rRNA gene analysis, ERIC-PCR, and RAPD assays. The possible relationships between the serotypes and genotypes were also assessed.
Materials and methods
Bacterial isolates
Thirty-seven field isolates of O. rhinotracheale were included in the study. The isolates were collected from either backyard farms or commercial flocks, or were supplied by the Veterinary Diagnostic Directorate (Budapest, Hungary). Serotype-specific O. rhinotracheale strains B3263/91, GGD 1261, ORV K91-201, ORV 94108 nr. 2, and O-95029 nr. 12229 (serotypes A–E, respectively) were purchased from the BCCM/LMG Bacteria Collection of Ghent University, and were included in the analysis. The isolates were cultured on Columbia agar enriched with 5% sheep blood, at 37°C for 48 h under 5% carbon dioxide. The isolates were identified with conventional biochemical tests, including the production of indole, urease, nitrate reductase, and the fermentation of arabinose, dulcitol, glucose, lactose, maltose, sucrose, and sorbitol. Pure cultures were stored at −70°C in skimmed milk until further analysis. The sources and geographic origins of the O. rhinotracheale isolates characterized in this study are presented in .
Table 1. Ornithobacterium rhinotracheale isolates used in this study.
Serotyping
Antisera against representative strains of serotypes A–E were raised in four-week-old specific-pathogen-free chickens, with the method described by Hafez and Sting (Citation1999). The serotype-specific strains and the field isolates were grown on Columbia agar enriched with 5% sheep blood, at 37°C for 48 h under microaerophilic conditions. The bacteria were harvested with 1.5 ml of phosphate-buffered saline (PBS), and the turbidity of the suspension was adjusted to three McFarland standards. Heat-stable antigens were prepared according to Heddleston et al. (Citation1972) and were used as the antigens in AGP. The AGP test was performed as described by Hafez and Sting (Citation1999).
DNA extraction
The DNA templates for PCR were prepared with the simple boiling method, as follows. Individual colonies were suspended in 50 μl of PCR-grade water (VWR, Radnor, PA, USA), heated at 100°C for 20 min, and then centrifuged at 12,000 × g for 5 min. The supernatant was used as the PCR template.
PCR
The isolates were identified with species-specific PCR (van Empel & Hafez, Citation1999). The ERIC-PCR mixture contained 1 × DreamTaq Buffer (including 20 mM MgCl2) (Thermo Fisher Scientific Inc., Asheville, NC, USA), 0.2 mM of each deoxynucleoside triphosphate (Thermo Fisher Scientific Inc.), 0.6 μM of each primer (ERIC1R 5′-ATGTAAGCTCCTGGGGATTCAC-3′ and ERIC2 5′-AAGTAAGTGACTGGGGTGAGCG-3′ (Sigma-Aldrich, St Louis, MO, USA), 2 U of DreamTaq DNA Polymerase (Thermo Fisher Scientific Inc.), 500 ng of template DNA, and PCR-grade water (VWR) to a final volume of 25 μl. The PCR conditions were: initial denaturation at 94°C for 4 min, followed by 30 cycles of denaturation at 94°C for 1 min, annealing at 52°C for 1.5 min, and extension at 72°C for 1.5 min, followed by a final extension at 72°C for 7 min. The partial 16S rRNA sequences were amplified with PCR using primers 27f (5’-AGAGTTTGATCMTGGCTCAG-3’) and 1492r (5’-TACGGYTACCTTGTTACGACTT-3’); the expected size of amplicon was 1465 bp (Lane, Citation1991). The PCR mixture was the same as for the ERIC-PCR. The amplification cycles were: initial denaturation at 94°C for 3 min, followed by 30 cycles of denaturation at 94°C for 30 s, annealing at 55°C for 45 s, and extension at 72°C for 1.5 min, followed by a final extension at 72°C for 7 min.
RAPD assays with M13, OPG11, and OPH19 primers
The RAPD reaction mixtures contained 1 × DreamTaq Buffer (including 20 mM MgCl2) (Thermo Fisher Scientific Inc.), 0.4 mM of each deoxynucleoside triphosphate, 2 μM of either Universal M13 primer (5′-GAGGGTGGCGGTTCT-3′), primer OPG11 (5′-TGCCCGTCGT-3′), or primer OPH19 (5′-CTGACCAGCC-3′) (Leroy-Sétrin et al., Citation1998), 2 U DreamTaq DNA Polymerase (Thermo Fisher Scientific Inc.), 500 ng of template DNA, and PCR-grade water (VWR) to a final volume of 25 μl. The amplification cycles for the RAPD assay with the M13 primer were: initial denaturation at 94°C for 4 min, 35 cycles of denaturation at 94°C for 1 min, an initial annealing temperature of 40°C (increasing by 0.2°C per cycle) for 1 min, and extension at 72°C for 1.5 min, followed by a final extension at 72°C for 7 min. The amplification cycles for the assays with the OPG11 and OPH19 primers were as described by Leroy-Sétrin et al. (Citation1998).
All amplification reactions were performed in a Biometra T1 Thermocycler (Biometra GmbH, Göttingen, Germany). Aliquots (8 µl) of the amplified DNA products were resolved by electrophoresis in 0.5% TAE buffer on 1% (w/v) TopVision Agarose (Thermo Fisher Scientific Inc.) stained with GelRed™ Nucleic Acid Gel Stain (Biotium Inc., Hayward, CA, USA), and photographed under UV transillumination with the Kodak Gel Logic 212 Imaging System (Rochester, NY, USA).
Partial 16S rRNA gene sequence analysis
The partial 16S rDNA nucleotide sequences were determined from the amplicons in both directions by a commercial sequencing facility (Macrogen, Amsterdam, the Netherlands). The sequence data were analysed with the BioEdit Sequence Alignment Editor software (v. 7.1.3.0; Hall, Citation1999). The nucleotide sequence identities were calculated with the pairwise distance algorithm in the MEGA6 software (Tamura et al., Citation2013). The evolutionary history was inferred with the neighbour-joining method, and the evolutionary distances were computed with the Jukes–Cantor method.
Analysis of ERIC-PCR and RAPD assay results
The presence (1) or absence (0) of different sized bands after each amplification reaction was used to generate a binary matrix. The Dice coefficient was used to estimate the genetic similarity of the O. rhinotracheale isolates, and a dendrogram was created with the unweighted pair group method with the arithmetic average method. The robustness of the dendrogram topology was assessed with 100 bootstrap replicates in PyElph version 1.4. (Pavel & Vasile, Citation2012).
Accession numbers
The GenBank accession numbers of the sequences reported in this paper are KX998665–KX998706.
Results
Identification
All the suspected O. rhinotracheale isolates gave negative results in the indole and nitrate reduction test, and were positive in the urease test. None of the isolates fermented arabinose, dulcitol, or sorbitol. The fermentation of glucose (27 positive/15 negative), lactose (23/19), maltose (24/18), and sucrose (4/38) was variable (). The identity of the isolates was confirmed with species-specific PCR.
Table 2. Results of the biochemical tests of Ornithobacterium rhinotracheale isolates.
Serotyping
Serotyping revealed that 31 of the 37 field isolates (83.8%) belonged to serotype A, one isolate (OR082) (2.7%) to serotype B, and four isolates (10.8%: OR007, OR009, OR047, and OR063) to serotype D. One isolate (2.7%) could not be typed with antisera raised against serotypes A–E. Thirty-three field isolates showed monospecific reactions. Two isolates (OR010 and OR060) were serotype A but cross-reacted with serotype B antiserum, whereas two isolates (OR007 and OR063) that were serotype D cross-reacted with serotype C antiserum. The cross-reactions appeared as weaker lines than the main reactions after 24 h, but became stronger after 48–72 h.
Partial 16S rRNA gene sequence analysis
16S rRNA sequence analysis grouped our isolates into two clusters (). The majority of the field isolates (34 isolates; 91.9%) showed 100% similarity to sequences from GenBank and the type strains of serotypes A, B, and E ().
Figure 1. Phylogenetic tree constructed from the partial 16S rRNA nucleotide sequences of Ornithobacterium rhinotracheale isolates (nucleotides 64–1398 based on the nucleotide positions of LMG 9086 T, accession no. U87101). Because 34 of the 37 field isolates showed 100% similarity in this region, only 25 field isolates are included here. *Reference sequences from GenBank.
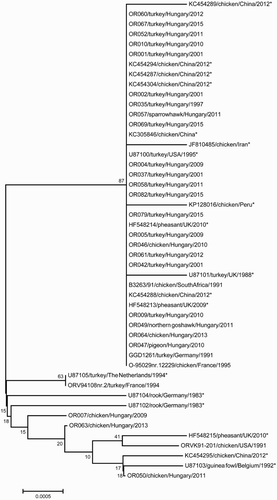
ERIC-PCR
Thirteen distinct ERIC patterns were identified among the isolates studied (). The most common pattern (type 1) consisted of 20 isolates (47.6%). Sixteen of the ERIC type 1 field isolates were recovered from turkeys, two from birds of prey, and one from a chicken. Serotype type strain B (GGD 1261), originating in a turkey, also grouped in type 1. Apart from this strain and OR082 (serotype B), all ERIC type 1 isolates belonged to serotype A. ERIC type 2 and type 3 (eight and four isolates, respectively) contained only isolates from turkeys. All ERIC type 2 and 3 isolates were serotype A, except for OR009 (ERIC type 2, serotype D). The remaining 10 isolates, including four of the five serotype-specific strains (A, C, D, and E), had unique ERIC patterns. Seven of these isolates were isolated from chickens, two from turkeys, and one from a pigeon.
RAPD assays with M13, OPG11, and OPH19 primers
The RAPD assay with the M13 primer assigned the O. rhinotracheale isolates to 10 distinct fingerprint patterns (). Thirty-one field isolates and the type strain for serotype A belonged to type 1. Type 2 consisted of two isolates, whereas eight isolates, including four of the five serotype-specific strains (B–E), showed unique RAPD patterns.
Figure 3. Dendrogram grouping the O. rhinotracheale isolates based on RAPD assay with the M13 primer using the unweighted pair group method with the arithmetic average method.
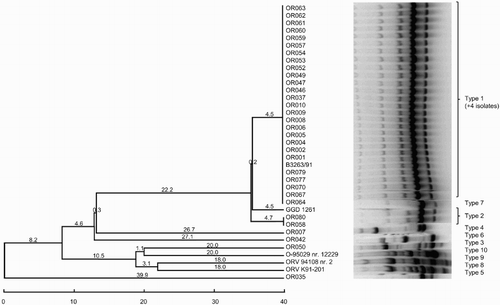
The OPG11 assay separated the field isolates into two main types (). Two field isolates and the type strains of serotypes A, B, and C had unique patterns, whereas the type strains for serotype D and E shared the same fingerprint. The RAPD assay with the OPH19 primer also assigned the isolates to two main types (). OR007 and OR050 formed type 4 and the type strains for serotypes D and E formed type 6, a pattern that differed in only one line from type 2. OR063 and the type of serotype strain C had unique fingerprints.
Discussion
Serotyping is the traditional method of distinguishing O. rhinotracheale isolates (van Empel & Hafez, Citation1999). Consistent with data in the literature (van Empel et al., Citation1997; Numee et al., Citation2012), serotype A was the most prevalent serotype among the strains isolated in Hungary in 1997–2015. Most of the isolates from turkeys and those from birds of prey belonged to this group. The five field isolates from chickens showed somewhat higher variability: two of them belonged to serotype A, two were serotype D, and one was not typeable with A–E antisera. However, the value of serotyping is dubious, especially with the recognition of vaccines that are cross-protective against several serotypes (Schuijffel et al., Citation2005).
A 16S rRNA sequence analysis revealed that our O. rhinotracheale isolates were closely related to strains recorded in GenBank. Other studies (Amonsin et al., Citation1997; Tsai & Huang, Citation2006) had similar results, and it is speculated that the O. rhinotracheale strains from all over the world are closely related. Taiwanese isolates from pigeons and chickens were grouped into two distinct clusters based on the analysis of a shorter fragment of 16S rRNA (Tsai & Huang, Citation2006). In this study, the 16S rRNA sequence analysis did not differentiate the isolates from different host species.
No relationship between the ERIC pattern and the year of isolation, or the serotype of the isolate could be established. However, the ERIC pattern and the host of origin of the isolate showed some correlation: type 2, type 3, and the majority of type 1 were made up of isolates from turkeys, whereas the isolates from chickens were highly variable. The eight isolates from chickens represented eight different types. Amonsin et al. (Citation1997) used ERIC-PCR to examine 54 O. rhinotracheale strains isolated from poultry in various countries between 1983 and 1995. They assigned the isolates to seven distinct fingerprint patterns, which did not correspond to their geographic origins. Strains isolated from chickens and turkeys were not distinguishable with this method, although the ERIC fingerprints of isolates recovered from wild birds differed from those from poultry. In another study, Koga and Zavaleta (Citation2005) analysed 25 O. rhinotracheale isolates from chickens with ERIC-PCR and all the isolates generated the same pattern.
No correspondence between the M13 RAPD patterns and either the isolation date, host, geographic origin, or serotype was established. Waldow (Citation2009) found a correlation between the serotype and RAPD pattern using the Universal M13 primer, but our results could not confirm this finding. Both serotype B and D isolates occurred among the RAPD M13 type 1 isolates, whereas four serotype A isolates (OR035, OR042, OR058, and OR080) had patterns other than type 1. Leroy-Sétrin et al. (Citation1998) tested eight different RAPD primers. OPG11 and OPG19 had the best discriminatory power, and when the RAPD assay was combined with ribotyping, they grouped their isolates into three clusters. However, no correlation with the origin of the isolates was found. Ozbey et al. (Citation2005) reported high genetic diversity among O. rhinotracheale isolates from chickens in Turkey with an RAPD assay using the OPG11 primer. However, they used only six field isolates, which represented six different profiles, so the number of isolates was too low to draw such far-reaching conclusions. Contrary to these findings, the OPG11 and OPH19 assays had less discriminatory power than either ERIC-PCR or the RAPD assay with the M13 primer in our study.
The fingerprints generated with ERIC-PCR and the RAPD assays did not strictly coincide: some isolates belonging to the same ERIC type showed different RAPD patterns and vice versa. However, in general, most isolates that belonged to the most common ERIC type also had common RAPD types. Isolates that had a unique ERIC pattern also belonged to rarer RAPD types in more than one of the RAPD assays (OR007, OR050, OR063, and the type strains for serotypes C, D, and E). In contrast, OR047 and OR061 had unique ERIC patterns but belonged to type 1 in all the RAPD assays. Although the isolates recovered from chickens showed more heterogeneity on ERIC-PCR and RAPD with primers OPG11 and OPH19 than the isolates from turkeys, this was not the case in the RAPD analysis with the M13 primer: four of the eight isolates from chickens belonged to RAPD type 1 and four isolates had unique patterns.
Although the results of the 16S rRNA analysis did not fully correspond to those of any of the RAPD assays, ERIC-PCR, or serotyping, the isolates that differed from the rest of the field isolates in their 16S rDNA sequence (OR007, OR050, and OR063) belonged to the rarer ERIC types or had unique patterns in more than one of the RAPD and ERIC assays, and were also rarer serotypes. This observation indicates that any of these three methods are capable of recognizing distinct types.
The isolates from chickens were more diverse than the isolates from turkeys according to all the methods used in this study. This heterogeneity might be explained by the fact that these isolates, like the one from the pigeon, were recovered from backyard farms, whereas the majority of the isolates from turkeys were obtained from densely populated and closed commercial flocks. It can be hypothesized that only a few types are circulating in relatively recently established large turkey flocks, whereas the chickens traditionally kept in small and separated backyard farms have had the opportunity to develop individual types. Interestingly, the two isolates recovered from birds of prey showed characteristics similar to those recovered from turkey farms. A case of O. rhinotracheale infection has been described in young falcons where the source of infection was probably the infected cockerels used as feed (Hafez & Lierz, Citation2010). Whether the two wild birds analysed in this study had any contact with commercial poultry flocks is unknown.
Several studies (Amonsin et al., Citation1997; Thieme et al., Citation2016) have proposed that the limited heterogeneity found among isolates can be explained by the fact that the O. rhinotracheale isolates recovered over large geographic areas and long time periods are a small group of closely related clones. Although the number of isolates and the geographic areas from which the isolates were obtained were limited, both the ERIC-PCR and RAPD analyses presented here support this theory.
In conclusion, we were able to discriminate O. rhinotracheale isolates with both ERIC-PCR and RAPD assays using different primers, at least to a certain level. Although a multilocus sequence typing method and database have recently been established (Thieme et al., Citation2016), repetitive extragenic palindromic PCR and RAPD assays remain relatively cost-efficient, rapid, sensitive, and specific typing methods, and may therefore be useful in epidemiological studies of O. rhinotracheale outbreaks. In this study, ERIC-PCR proved the most useful technique for detecting intraspecies genetic diversity in O. rhinotracheale isolates.
Acknowledgements
The authors would like to thank Janine Miller, who provided English language editing services on behalf of Edanz Group.
Disclosure statement
No potential conflict of interest was reported by the author(s).
Additional information
Funding
References
- Amonsin, A., Wellehan, J.F., Li, L.L., Vandamme, P., Lindemann, C., Edman, M., Robinson, R. & Kapur, V. (1997). Molecular epidemiology of Ornithobacterium rhinotracheale. Journal of Clinical Microbiology, 35, 2894–2898.
- Charlton, B.R., Channing-Santiago, S.E., Bickford, A.A., Cardona, C.J., Chin, R.P., Cooper, G.L., Droual, R., Jeffrey, J.S., Meteyer, C.U., Shivaprasad, H.L. & Walker, R.L. (1993). Preliminary characterization of a pleomorphic, Gram-negative rod associated with avian respiratory disease. Journal of Veterinary Diagnostic Investigation, 5, 47–51. doi: 10.1177/104063879300500111
- Chin, R.P., van Empel, P.C.M. & Hafez, H.M. (2013). Ornithobacterium rhinotracheale infection. In D.E. Swayne, J.R. Glisson, L.R. McDougald, L.K. Nolan, D.L. Suarez & V.L. Nair (Eds.), Diseases of Poultry (13th ed., pp. 828–834). Ames: Wiley-Blackwell.
- Clarridge, J.E. (2004). Impact of 16S rRNA gene sequence analysis for identification of bacteria on clinical microbiology and infectious diseases. Clinical Microbiology Reviews, 17, 840–862. doi: 10.1128/CMR.17.4.840-862.2004
- Hafez, H.M. (2002). Diagnosis of Ornithobacterium rhinotracheale. International Journal of Poultry Science, 1, 114–118. doi: 10.3923/ijps.2002.114.118
- Hafez, H.M. & Lierz, M. (2010). Ornithobacterium rhinotracheale in nestling falcons. Avian Diseases, 54, 161–163. doi: 10.1637/9008-080309-Case.1
- Hafez, H.M. & Sting, R. (1999). Investigations on different Ornithobacterium rhinotracheale “ORT” isolates. Avian Diseases, 43, 1–7. doi: 10.2307/1592755
- Hall, T.A. (1999). BioEdit: a user-friendly biological sequence alignment editor and analysis program for windows 95/98/NT. Nucleic Acids Symposium Series, 41, 95–98.
- Heddleston, K.L., Gallagher, J.E. & Rebers, P.A. (1972). Gel diffusion precipitin test for serotyping Pasteurella multocida from avian species. Avian Diseases, 16, 925–936. doi: 10.2307/1588773
- Hinz, K.H., Hafez, H.M. (1997). The early history of Ornithobacterium rhinotracheale (ORT). Archiv fur Geflugelkunde, 61, 95–96.
- Koga, Y. & Zavaleta, A.I. (2005). Intraspecies genetic variability of Ornithobacterium rhinotracheale in commercial birds in Peru. Avian Diseases, 49, 108–111. doi: 10.1637/7235-070804R
- Lane, D.J. (1991). 16S/23S rRNA sequencing. In E. Stackebrandt M. Goodfellow (Eds.), Nucleic Acid Techniques in Bacterial Systematics (pp. 115–175). New York: John Wiley.
- Leroy-Sétrin, S., Flaujac, G., Thénaisy, K. & Chaslus-Dancla, E. (1998). Genetic diversity of Ornithobacterium rhinotracheale strains isolated from poultry in France. Letters in Applied Microbiology, 26, 189–193. doi: 10.1046/j.1472-765X.1998.00315.x
- Numee, S., Hauck, R. & Hafez, H.M. (2012). Detection and typing of Ornithobacterium rhinotracheale from German poultry flocks. Avian Diseases, 56, 654–658. doi: 10.1637/10079-021412-Reg.1
- Olive, D.M. & Bean, P. (1999). Principles and applications of methods for DNA-based typing of microbial organisms. Journal of Clinical Microbiology, 37, 1661–1669.
- Ozbey, G., Ertas, H.B. & Muz, A. (2005). Random amplified polymorphic DNA (RAPD) analysis of Ornithobacterium rhinotracheale strains isolated from chickens in Turkey. Veterinarni medicina, 50, 526–530.
- Pavel, A.B. & Vasile, C.I. (2012). PyElph – a software tool for gel images analysis and phylogenetics. BMC Bioinformatics, 13, 9. doi: 10.1186/1471-2105-13-9
- Schuijffel, D.F., van Empel, P.C.M., Pennings, A.M., van Putten, J.P. & Nuijten, P.J. (2005). Passive immunization of immune-suppressed animals: chicken antibodies protect against Ornithobacterium rhinotracheale infection. Vaccine, 23, 3404–3411. doi: 10.1016/j.vaccine.2005.01.095
- Tamura, K., Stecher, G., Peterson, D., Filipski, A. & Kumar, S. (2013). MEGA6: molecular evolutionary genetics analysis version 6.0. Molecular Biology and Evolution, 30, 2725–2729. doi: 10.1093/molbev/mst197
- Tanyi, J., Bistyák, A., Kaszanyitzky, É., Vetési, F. & Dobos-Kovács, M. (1995). Isolation of Ornithobacterium rhinotracheale from chickens and turkeys with respiratory disease. Preliminary report [in Hungarian, with English abstract]. Magyar Állatorvosok Lapja, 50, 328–330.
- Thieme, S., Mühldorfer, K., Lüschow, D., Hafez, H.M. & Woo, P.C.Y. (2016). Molecular characterization of the recently emerged poultry pathogen Ornithobacterium rhinotracheale by multilocus sequence typing. PLoS ONE, 11, e0148158. doi: 10.1371/journal.pone.0148158
- Tsai, H.J. & Huang, C.W. (2006). Phenotypic and molecular characterization of isolates of Ornithobacterium rhinotracheale from chickens and pigeons in Taiwan. Avian Diseases, 50, 502–507. doi: 10.1637/7527-031906R.1
- Vandamme, P., Segers, P., Vancanneyt, M., Van Hove, K., Mutters, R., Hommez, J., Dewhirst, F., Paster, B., Kersters, K., Falsen, E., Devriese, L.A., Bisgaard, M., Hinz, K.-H. & Mannheim, W. (1994). Ornithobacterium rhinotracheale gen. nov., sp. nov. isolated from the avian respiratory tract. International Journal of Systematic Bacteriology, 44, 24–37. doi: 10.1099/00207713-44-1-24
- van Empel, P.C.M. & Hafez, H.M. (1999). Ornithobacterium rhinotracheale: a review. Avian Pathology, 28, 217–227. doi: 10.1080/03079459994704
- van Empel, P.C.M., van der Bosch, H., Loeffen, P. & Storm, P. (1997). Identification and serotyping of Ornithobacterium rhinotracheale. Journal of Clinical Microbiology, 35, 418–421.
- Varga, J., Fodor, L. & Makrai, L. (2001). Biochemical characteristics, antibiotic susceptibility of some Ornithobacterium rhinotracheale strains and their transmission via eggs. Acta Veterinaria Hungarica, 49, 125–130. doi: 10.1556/004.49.2001.2.1
- Waldow, K. (2009). Untersuchungen zur Embryoletalität, Genotypisierung und Resistenzlage aktueller Ornithobacterium rhinotracheale-Isolate (Ph.D. Thesis). Freie Universität Berlin, Germany.