ABSTRACT
Salmonella biofilm formation is important to environmental stress resistance and virulence. However, the roles of the csgA and bcsA genes, which affect curli protein and cellulose production, respectively, in Salmonella enterica serovar Pullorum, are unknown. Here we constructed deletions in the csgA and bcsA genes in S. enterica serovar Pullorum strain S6702 and evaluated several aspects of biofilm formation and virulence. ΔcsgA showed decreased production of curli fimbriae, while ΔbcsA had reduced cellulose production. Both mutants had a reduced ability to form biofilms. ΔcsgA was reduced in adhesion and invasion to HeLa cells and exhibited decreased intracellular proliferation in HD11 macrophages. ΔbcsA exhibited increased proliferation in HD11 cells and replicated better in chicken spleens, as compared to the wild-type strain. ΔcsgA virulence was attenuated in assays involving oral challenge of one-day-old chickens.
Introduction
Salmonella Pullorum negatively impacts the chicken industry by causing systemic disease in young chickens. Infections can cause reduced weight gain, reduced egg laying and defects in reproductive tract functions (Barrow & Neto, Citation2011). Salmonella Pullorum may persist for several months in the spleen, leading to reproductive tract infections and vertical transmission of the infection to eggs or progeny (Wigley et al., Citation2001). Salmonella can form biofilms, which can enhance bacterial resistance to adverse conditions (Mangalappalli-Illathu & Korber, Citation2006). Biofilms consist of aggregates of microorganisms embedded in a self-produced extracellular matrix of polymeric substances. Salmonella can form biofilms on a variety of surfaces and cellulose and curli fibres are major components of their biofilm matrix (Solomon et al., Citation2005). The regulation of biofilm formation in Salmonella has been extensively studied. It is known that CsgD activates the production of curli fimbriae by transcriptional activation of the csgBAC operon that encodes the structural genes of curli fimbriae (Römling et al., Citation1998) while AdrA activates cellulose production by activating the basABZC-bcsEFG operons (Zogaj et al., Citation2001; Solano et al., Citation2002). Here we characterized the roles of the csgA and bcsA genes in Salmonella Pullorum biofilm formation and virulence.
Materials and methods
Strains, plasmids and growth conditions
The bacterial strains and plasmids used in this study are described in Table S1 and the primers used are listed in Table S2. Strains were routinely cultured in Luria–Bertani (LB; Difco, Sparks, MD, USA) broth and LB agar medium (containing 1.5% (w/v) agar) with antibiotic supplementation as needed. Tryptic soy broth (TSB; Difco) diluted 1:10 (1/10 TSB) in distilled water was used for biofilm assays. The csgA and bcsA genes of Salmonella Pullorum strain S6702 (Lu et al., Citation2011) were deleted by using standard lambda red-mediated mutagenesis procedures (Datsenko & Wanner, Citation2000). pKD3 was used for mutagenesis and pCP20 was used to resolve the cat gene that interrupted the csgA and bcsA genes. pCP20 was cured from the final deletion strains by growing the mutants at 43°C. All mutants were verified by PCR amplification using the primers csgA-F/R and bcsA-F/R (Table S2). PCR products were confirmed using DNA sequencing (Huada Gene Sequencing Company, Shanghai, China). Complementation strains were constructed by expressing each gene from the pEASY plasmid using the TA cloning vector One Step Cloning Kit (Promega Biotech Co., Ltd., Beijing, China). Growth assays were performed in LB broth at 37°C with shaking at 220 rpm. Samples from each bacterial culture were spectrophotometrically monitored hourly for 8 h (Lu et al., Citation2012).
Biofilm assays
Biofilm formation was measured in 1/10 TSB, as previously described (Kim et al., Citation2007; Crawford et al., Citation2008). Overnight broth cultures of each strain were diluted 1:100 in 1/10 TSB and 100 µl of each bacterial suspension was added to a 96-well U-bottomed polystyrene microtitre plate (Corning, New York, NY, USA). Wells containing only medium served as negative controls. Plates were incubated at 28°C for 24 h without shaking. Non-adherent bacteria were removed and the wells were gently washed three times with 200 µl of distilled water. One hundred microlitre of 0.4% crystal violet (CV, v/v) was then added for 20 min. Wells were washed three times with distilled water. The remaining CV bound to the adherent cells was solubilized with 100 µl 33% acetic acid with anhydrous ethanol for 30 min and then the optical density (OD550) nm was measured (Bio-Tek, Winooski, VT, USA). Assays were performed three times, using duplicate wells in each independent assay.
To assay biofilm formation on glass surfaces, 2 ml of overnight cultures diluted 1:100 in 1/10 TSB was added to borosilicate glass test tubes and incubated at 28°C for 48 h. Tubes were washed three times gently with distilled water. Two millilitre of 0.4% CV was added for 20 min.
To assay for curli production, 10 µl of overnight broth cultures was added to Congo red plates and incubated at 28°C for 4 days, when colony morphology was assessed (Anriany et al., Citation2006). Cellulose production was monitored by supplementing LB agar plates with 200 µg/ml calcofluor (Anriany et al., Citation2006).
Field emission scanning electron microscope
Biofilm morphology was also determined by using scanning electron microscopy, as previously described (Anriany et al., Citation2006; Lu et al., Citation2012). Polystyrene coverslips (5 × 5 mm) were inoculated as described above, fixed in 3% glutaraldehyde in 0.1 M phosphate-buffered saline (PBS) (pH 7.0) for 2 h at 4°C, washed three times with PBS, and dehydrated with increasing concentrations of ethanol and isoamyl acetate. Samples were dried using critical point-drying for 5 h, coated with gold palladium alloy, and observed with an FESEM S4800 (Hitachi, Tokyo, Japan).
Protein profiles
Curli protein was detected, as described in a previous study (Anriany et al., Citation2006). One millilitre of bacterial cell suspension (adjusted to an OD600 of 3.0) was centrifuged, and the cell pellets were resuspended in 100 µl sodium dodecyl sulfate (SDS) sample buffer (62.5 mM Tris/HCl (pH 6.8), 10% glycerol, 2% SDS, and 0.1% bromophenol blue) and boiled for 10 min. The cell lysate was centrifuged and the pellet was washed twice with sterile water, dissolved in 100 µl 97% formic acid (Sigma, St. Louis, MO, USA), frozen at 70°C, and then lyophilized. The samples were re-homogenized and suspended in 100 µl SDS sample buffer, sonicated for 5 s, and loaded onto a 15% polyacrylamide gel. Each strain was examined by comparing the overall protein profile on the Coomassie blue-stained gel (Anriany et al., Citation2006).
Lipopolysaccharide profiles
Each strain was inoculated into 50 ml LB and shaken at 37°C for 18 h. Cells were collected using centrifugation at 1792 x g for 10 min and then extracted using phenol–water (Wigley et al., Citation2001). Purified lipopolysaccharide (LPS) samples (20 µl) were resolved using SDS–polyacrylamide gel electrophoresis (SDS-PAGE) with 15% separating gels and visualized by silver staining for carbohydrates (Tsai & Frasch, Citation1982).
Adhesion and invasion assays
Adhesion and invasion assays were carried out according to standard methods (Peng et al., Citation2005). HeLa cells were cultured in Dulbecco modified Eagle medium (HyClone Laboratories Inc., Logan, UT, USA) supplemented with 10% heat-inactivated foetal bovine serum (FBS; Gibco, New York, USA) at 37°C in 5% CO2 humidified incubator for 16 h. For adhesion assays, HeLa cells (1 × 105 cells) were cultured in 24-well cell-bind culture plates (Corning) and incubated for 16 h. Bacterial strains were added at a multiplicity of infection of 100:1 bacteria/cell proportion and incubated at 37°C for 2 h. Non-adherent bacteria were removed by washing with PBS and cells were released from the plates by adding 0.05% trypsin-ethylenediaminetetraacetic acid for 5 min. This cell suspension was serially diluted in 15% glycerol PBS and cultured on MacConkey plates to calculate the number of viable bacteria. For invasion assays, after 2 h incubation with bacteria, 100 µg/ml of gentamicin was added for 90 min. After washing, cells were lysed in 0.1% Triton X-100 PBS for 5 min, serially diluted, and then cultured on MacConkey agar plates. The avian macrophage cell line HD11 was used for macrophage infection assays and the method was the same as that mentioned for the adhesion and invasion assays (Mu et al., Citation2013).
Determination of LD50 in chickens
All bird experiments were approved by the Jiangsu Administrative Committee for Laboratory Animals, and complied with the guidelines of laboratory animal welfare and ethics of the Jiangsu Administrative Committee for Laboratory Animals (Permission number: SYXKSU-2007-0005). One-day-old specific pathogen–free (SPF) chickens were hatched from chicken embryos obtained from Merial Beijing Experimental Animal Technology Co., Ltd. (Beijing, China) and provided with antibiotic-free feed and water. Bacterial concentrations were first adjusted to 109 colony-forming units (CFUs)/ml and then further diluted for oral inoculation of chickens. Chickens were randomly assigned into 10 groups (n = 6/group) and inoculated orally with approximately 106, 107 or 108 CFU of each bacterial strain in 0.1 ml. The control group received 0.1 ml of PBS. Mortality rates were recorded and calculated over a two-week period. Moribund chickens were euthanized humanely according to the guidelines of laboratory animal welfare. The 50% lethal dose (LD50) was calculated using the Reed–Muench method (Reed & Muench, Citation1938).
Distribution and colonization of bacteria in organs
To assess bacterial persistence in the internal organs of chickens, one-day-old SPF chickens (n = 6/group) were euthanized at 12- and 48-h post-challenge, and then liver and spleen samples were collected aseptically. Samples were weighed, suspended in 1 ml of 15% glycerol PBS and homogenized. One hundred microlitre of serial 10-fold dilutions of homogenates was plated onto MacConkey agar and incubated at 37°C for 20 h. Bacteria were enumerated and expressed as log10 CFUs/g tissue (Yin et al., Citation2015).
Statistical analysis
All data are expressed as mean and standard deviations (SD). All statistical analyses were performed using IBM SPSS Statistics 22. P values less than 0.05 were considered significant, using one-way analysis of variance.
Results
Characterization of bcsA and csgA mutants
The csgA and bcsA genes were deleted from Salmonella Pullorum strain S6702 using lambda red mutagenesis ((A)). The growth rates of ΔbcsA and ΔcsgA were similar to that of the wild-type (WT) strain ((B)). Biofilm formation was assayed by performing CV of bacterial strains grown on polystyrene plates. The WT and ΔbcsA strains stained violet, but ΔcsgA was colourless ((A)). Complementation of ΔcsgA restored it to a WT phenotype. Similar conclusions were reached after quantifying the extent of CV staining ((B)).
Figure 1. Construction and characterization of ΔcsgA and ΔbcsA mutants. (A) Mutant validation. M, DNA Ladder; 1, Fragments amplified from WT strain; 2, cat gene insertion; 3, Excision of cat gene. (B) Growth curves of WT, ΔcsgA, and ΔbcsA mutants.
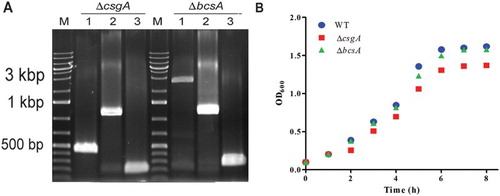
Figure 2. Biofilm assays. (A) CV staining of bacteria grown on 96-well plates. (B) CV staining quantification by measuring optical density (OD550). (C) Biofilm formation in glass tubes incubated at 28°C for 48 h. (D) Field emission scanning electron microscope analysis of bacteria grown on coverslips at 28°C for 24 h.
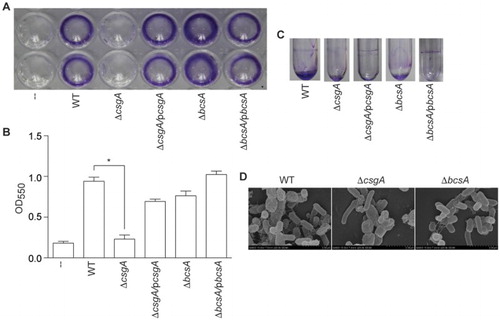
In glass tube assays, both ΔcsgA and ΔbcsA failed to form rings at the liquid–air interface ((C)). In field emission scanning electron microscope analyses, the WT strain had meshwork-like structures surrounding the bacteria, while ΔcsgA produced thin and smooth cell surface structures, and ΔbcsA produced only few extracellular components ((D)). Thus, deleting ΔcsgA or ΔbcsA appears to reduce biofilm formation in Salmonella Pullorum strain S6702.
Identification of biofilm components
Colony morphologies and colours were next investigated by growing the strains on Congo red plates. After growth at 28°C for 4 days, while the WT strain appeared red, dry and rough, the mutant colonies were smooth. ΔcsgA produced a pink/white colony, indicating a lack of curli production, and ΔbcsA produced a brown/white colony, indicating a lack of cellulose production ((A)). In calcofluor staining assays, ΔbcsA strain exhibited reduced fluorescence, also indicating that the mutation affects cellulose production ((B)).
Protein patterns and LPS profiles
Comparative examination of protein patterns on SDS-PAGE showed that both WT and ΔbcsA produced a 15.3-kDa curli protein, but ΔcsgA did not ((A), arrow). Silver staining of purified LPS components showed that LPS patterns differed among the WT, ΔcsgA and ΔbcsA strains ((B)).
Cell culture assays
ΔcsgA exhibited significantly reduced adhesion and invasion into HeLa cells, as compared with the WT and ΔbcsA strains (). In HD11 macrophages, ΔbcsA showed significantly greater invasion and proliferation, as compared to WT, whereas ΔcsgA had a decreased proliferation rate ().
Figure 5. Adhesion and invasion assays. Adhesion to and invasion of HeLa cells.
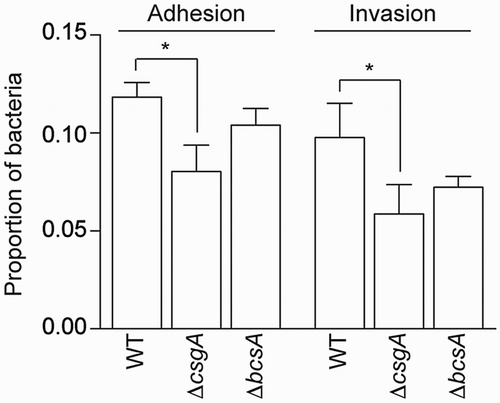
Table 1. Proliferation of strains in HD11 cells.
Virulence assays in chickens
One-day-old SPF chickens were orally challenged with different amounts of WT or mutant strains and LD50s values were determined. WT and ΔbcsA had similar LD50s (108.01 and 108.03), respectively, whereas ΔcsgA had a significantly higher LD50 of 108.83, indicating some degree of attenuation (). At 12 h post-challenge, the amounts of ΔcsgA and ΔbcsA present in the liver and spleen were similar to WT level. At 48 h post-challenge, the amount of ΔcsgA in the liver was significantly lower than WT level (P < 0.01). In contrast, the amount of ΔbcsA in the spleen was significantly higher than WT level (P < 0.01) ((A,B)).
Figure 6. Virulence assays in chickens. The bacterial loads in one-day-old SPF chickens at (A) 12 h and (B) 48 h post-challenge are shown.
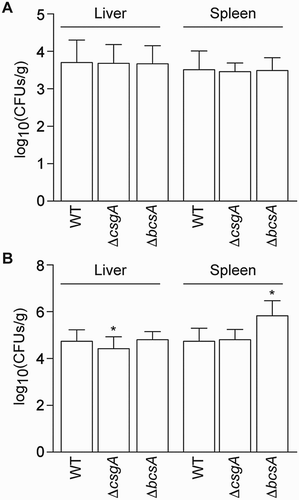
Table 2. Determination of LD50 in one-day-old SPF chickens after oral challenge and monitored over a two-week experimental period.
Discussion
Pullorum disease caused by Salmonella Pullorum is a common disease that leads to substantial economic losses (Li et al., Citation2013). Salmonella are capable of forming biofilms on a wide variety of surfaces (Korber et al., Citation1997; Römling et al., Citation2003; Lu et al., Citation2011). Increased understanding of Salmonella biofilm formation mechanisms may result in improved control of salmonellosis in humans and birds. Curli fimbriae are involved in the colonization of rigid surfaces and enhance both adhesion and cell-to-cell interactions during biofilm development (Austin et al., Citation1998). The csgA gene is involved in curli formation and its expression is regulated by csgD, which activates the csgBAC operon. In addition, cellulose is another component of the biofilm extracellular matrix, which is activated by the adrA gene at the post-transcriptional level through direct interaction with one or more of the gene products of bacterial cellulose synthesis operons bcsABZC-bcsEFG (Zogaj et al., Citation2001; Gerstel & Römling, Citation2003; Grantcharova et al., Citation2010).
To determine the effects of the csgA and bcsA genes on biofilm formation and virulence in Salmonella Pullorum, we constructed deletion mutations in these genes. We found that ΔcsgA was defective in curli production while ΔbcsA was defective in cellulose production. Both deficiencies resulted in reduced biofilm formation in several in vitro assays. In a previous study (Lu et al., Citation2012), a Salmonella Pullorum ompR mutant did not produce any curli protein but produced WT levels of cellulose. This strain did not form biofilms, indicating that curli may play an important role in biofilm formation in Salmonella Pullorum. Our findings provide evidence that the csgA gene plays a critical role in biofilm formation in Salmonella Pullorum, while the bcsA gene has a reduced impact on biofilm formation.
LPS is also involved in biofilms. Deletion of ddhC and waaG genes in Salmonella Typhimurium, which are involved in the LPS synthesis, had opposing effects on biofilm formation in these mutants, depending on osmolality (Anriany et al., Citation2006). rfaG (also called waaG) and rfbH (also called ddhC) deletions in Salmonella Pullorum altered LPS profiles but not biofilm formation (Lu et al., Citation2012). In our study, the LPS molecules of the ΔcsgA and ΔbcsA in Salmonella Pullorum were smaller than that of WT. Further investigation is needed to understand the exact reasons for this phenomenon.
Curli are adhesive fimbriae of Salmonella enterica and Escherichia coli. In enterohemorrhagic and enteropathogenic E. coli, single mutations in csgA or bcsA do not significantly alter bacterial adherence to human colonic HT-29 epithelial cells, while a double csgA/bcsA mutant was significantly less adherent, indicating a synergistic role of curli and cellulose in cell adherence (Saldaña et al., Citation2009). Similarly, cellulose-deficient Salmonella Enteritidis mutants showed no difference in bacterial adherence and invasion assays of eukaryotic cells (Solano et al., Citation2002). However, mutations of csgA and csgB in Salmonella Enteritidis affected attachment to alfalfa sprouts differently (Barak et al., Citation2005). Our data indicated that the ΔcsgA strain had significantly reduced adhesion and invasion into HeLa cells, while ΔbcsA did not. In HD11 macrophages, ΔbcsA exhibited enhanced proliferation, in agreement with previous studies indicating that preventing cellulose synthesis increases virulence, whereas stimulation of cellulose synthesis inside macrophages decreases virulence (Pontes et al., Citation2015).
The ΔcsgA mutant of Salmonella Typhimurium was not attenuated during murine infection (White et al., Citation2008). However, cellulase BcsZ, which negatively regulates cellulose biosynthesis, enabled Salmonella to colonize the host in the murine typhoid fever infection model (Ahmad et al., Citation2016). In a chicken oral challenge model, a csgA deletion mutant from Salmonella Enteritidis showed no significant differences in colonization of chicken caeca, invasion into the liver and spleen, or shedding in faeces, as compared to the WT strain (Rajashekara et al., Citation2000). A csgB insertion mutant was defective only in intestinal colonization (Addwebi et al., Citation2014). In our study, the ΔcsgA mutant was attenuated for virulence in a chicken oral challenge model, while the ΔbcsA mutant showed similar virulence as the WT strain S6702. The attenuated phenotype of the ΔcsgA mutant may be due to reduced adhesion and invasion of epithelial cells or due to decreased intracellular proliferation in macrophages. These data help to clarify the respective roles of csgA and bcsA in Salmonella Pullorum biofilm formation and virulence.
Highlights.doc
Download MS Word (32.5 KB)Acknowledgements
We thank Dr Qin Tao for suggestions and the testing centre of Yangzhou University for assistance with emission scanning electron microscopy experiments.
Disclosure statement
No potential conflict of interest was reported by the authors.
Additional information
Funding
References
- Addwebi, T.M., Call, D.R. & Shah, D.H. (2014). Contribution of Salmonella Enteritidis virulence factors to intestinal colonization and systemic dissemination in 1-day-old chickens. Poultry Science, 93, 871–881. doi: 10.3382/ps.2013-03710
- Ahmad, I., Rouf, S.F., Sun, L., Cimdins, A., Shafeeq, S., Le Guyon, S., Schottkowski, M., Rhen, M. & Römling, U. (2016). BcsZ inhibits biofilm phenotypes and promotes virulence by blocking cellulose production in Salmonella enterica serovar Typhimurium. Microbial Cell Factories, 15, 177. doi: 10.1186/s12934-016-0576-6
- Anriany, Y., Sahu, S.N., Wessels, K.R., McCann, L.M. & Joseph, S.W. (2006). Alteration of the rugose phenotype in waaG and ddhC mutants of Salmonella enterica serovar Typhimurium DT104 is associated with inverse production of curli and cellulose. Applied and Environmental Microbiology, 72, 5002–5012. doi: 10.1128/AEM.02868-05
- Austin, J.W., Sanders, G., Kay, W.W. & Collinson, S.K. (1998). Thin aggregative fimbriae enhance Salmonella enteritidis biofilm formation. FEMS Microbiology Letters, 162, 295–301. doi: 10.1111/j.1574-6968.1998.tb13012.x
- Barak, J.D., Gorski, L., Naraghi-Arani, P. & Charkowski, A.O. (2005). Salmonella enterica virulence genes are required for bacterial attachment to plant tissue. Applied and Environmental Microbiology, 71, 5685–5691. doi: 10.1128/AEM.71.10.5685-5691.2005
- Barrow, P.A. & Neto, O.C.F. (2011). Pullorum disease and fowl typhoid – new thoughts on old diseases: a review. Avian Pathology, 40, 1–13. doi: 10.1080/03079457.2010.542575
- Crawford, R.W., Gibson, D.L., Kay, W.W. & Gunn, J.S. (2008). Identification of a bile-induced exopolysaccharide required for Salmonella biofilm formation on gallstone surfaces. Infection and Immunity, 76, 5341–5349. doi: 10.1128/IAI.00786-08
- Datsenko, K.A. & Wanner, B.L. (2000). One-step inactivation of chromosomal genes in Escherichia coli K-12 using PCR products. Proceedings of the National Academy of Sciences, 97, 6640–6645. doi: 10.1073/pnas.120163297
- Gerstel, U. & Römling, U. (2003). The csgD promoter, a control unit for biofilm formation in Salmonella typhimurium. Research in Microbiology, 154, 659–667. doi: 10.1016/j.resmic.2003.08.005
- Grantcharova, N., Peters, V., Monteiro, C., Zakikhany, K. & Römling, U. (2010). Bistable expression of CsgD in biofilm development of Salmonella enterica serovar Typhimurium. Journal of Bacteriology, 192, 456–466. doi: 10.1128/JB.01826-08
- Kim, Y., Oh, S., Ahn, E.Y., Imm, J.-Y., Oh, S., Park, S. & Kim, S.H. (2007). Proteome analysis of virulence factor regulated by autoinducer-2–like activity in Escherichia coli O157: H7. Journal of Food Protection®, 70, 300–307. doi: 10.4315/0362-028X-70.2.300
- Korber, D., Choi, A., Wolfaardt, G., Ingham, S. & Caldwell, D. (1997). Substratum topography influences susceptibility of Salmonella enteritidis biofilms to trisodium phosphate. Applied and Environmental Microbiology, 63, 3352–3358.
- Li, Q., Hu, Y., Chen, J., Liu, Z., Han, J., Sun, L. & Jiao, X. (2013). Identification of Salmonella enterica serovar Pullorum antigenic determinants expressed in vivo. Infection and Immunity, 81, 3119–3127. doi: 10.1128/IAI.00145-13
- Lu, Y., Chen, S., Dong, H., Sun, H., Peng, D. & Liu, X. (2012). Identification of genes responsible for biofilm formation or virulence in Salmonella enterica serovar Pullorum. Avian Diseases, 56, 134–143. doi: 10.1637/9806-052411-Reg.1
- Lu, Y., Dong, H., Chen, S., Chen, Y., Peng, D. & Liu, X. (2011). Characterization of biofilm formation by Salmonella enterica serovar Pullorum strains. African Journal of Microbiology Research, 5, 2428–2437.
- Mangalappalli-Illathu, A.K. & Korber, D.R. (2006). Adaptive resistance and differential protein expression of Salmonella enterica serovar Enteritidis biofilms exposed to benzalkonium chloride. Antimicrobial Agents and Chemotherapy, 50, 3588–3596. doi: 10.1128/AAC.00573-06
- Mu, X., Huan, H., Xu, H., Gao, Q., Xiong, L., Gao, R. & Liu, X. (2013). The transfer-messenger RNA-small protein B system plays a role in avian pathogenic Escherichia coli pathogenicity. Journal of Bacteriology, 195, 5064–5071. doi: 10.1128/JB.00628-13
- Peng, D., Hong, W., Choudhury, B.P., Carlson, R.W. & Gu, X.-X. (2005). Moraxella catarrhalis bacterium without endotoxin, a potential vaccine candidate. Infection and Immunity, 73, 7569–7577. doi: 10.1128/IAI.73.11.7569-7577.2005
- Pontes, M.H., Lee, E.-J., Choi, J. & Groisman, E.A. (2015). Salmonella promotes virulence by repressing cellulose production. Proceedings of the National Academy of the Sciences USA, 112, 5183–5188. doi: 10.1073/pnas.1500989112
- Rajashekara, G., Munir, S., Alexeyev, M.F., Halvorson, D.A., Wells, C.L. & Nagaraja, K.V. (2000). Pathogenic role of SEF14, SEF17, and SEF21 fimbriae in Salmonella enterica serovar Enteritidis infection of chickens. Applied and Environmental Microbiology, 66, 1759–1763. doi: 10.1128/AEM.66.4.1759-1763.2000
- Reed, L.J. & Muench, H. (1938). A simple method of estimating fifty per cent endpoints. American Journal of Epidemiology, 27, 493–497. doi: 10.1093/oxfordjournals.aje.a118408
- Römling, U., Bian, Z., Hammar, M., Sierralta, W.D. & Normark, S. (1998). Curli fibers are highly conserved between Salmonella typhimurium and Escherichia coli with respect to operon structure and regulation. Journal of Bacteriology, 180, 722–731.
- Römling, U., Bokranz, W., Rabsch, W., Zogaj, X., Nimtz, M. & Tschäpe, H. (2003). Occurrence and regulation of the multicellular morphotype in Salmonella serovars important in human disease. International Journal of Medical Microbiology, 293, 273–285. doi: 10.1078/1438-4221-00268
- Saldaña, Z., Xicohtencatl-Cortes, J., Avelino, F., Phillips, A.D., Kaper, J.B., Puente, J.L. & Girón, J.A. (2009). Synergistic role of curli and cellulose in cell adherence and biofilm formation of attaching and effacing Escherichia coli and identification of Fis as a negative regulator of curli. Environmental Microbiology, 11, 992–1006. doi: 10.1111/j.1462-2920.2008.01824.x
- Solano, C., García, B., Valle, J., Berasain, C., Ghigo, J.M., Gamazo, C. & Lasa, I. (2002). Genetic analysis of Salmonella enteritidis biofilm formation: critical role of cellulose. Molecular Microbiology, 43, 793–808. doi: 10.1046/j.1365-2958.2002.02802.x
- Solomon, E.B., Niemira, B.A., Sapers, G.M. & Annous, B.A. (2005). Biofilm formation, cellulose production, and curli biosynthesis by Salmonella originating from produce, animal, and clinical sources. Journal of Food Protection®, 68, 906–912. doi: 10.4315/0362-028X-68.5.906
- Tsai, C.-M. & Frasch, C.E. (1982). A sensitive silver stain for detecting lipopolysaccharides in polyacrylamide gels. Analytical Biochemistry, 119, 115–119. doi: 10.1016/0003-2697(82)90673-X
- White, A.P., Gibson, D.L., Grassl, G.A., Kay, W.W., Finlay, B.B., Vallance, B.A. & Surette, M.G. (2008). Aggregation via the red, dry, and rough morphotype is not a virulence adaptation in Salmonella enterica serovar Typhimurium. Infection and Immunity, 76, 1048–1058. doi: 10.1128/IAI.01383-07
- Wigley, P., Berchieri, A., Page, K., Smith, A. & Barrow, P. (2001). Salmonella enterica serovar Pullorum persists in splenic macrophages and in the reproductive tract during persistent, disease-free carriage in chickens. Infection and Immunity, 69, 7873–7879. doi: 10.1128/IAI.69.12.7873-7879.2001
- Yin, J., Cheng, Z., Wang, X., Xu, L., Li, Q., Geng, S. & Jiao, X. (2015). Evaluation of the Salmonella enterica serovar Pullorum pathogenicity island 2 mutant as a candidate live attenuated oral vaccine. Clinical and Vaccine Immunology, 22, 706–710. doi: 10.1128/CVI.00130-15
- Zogaj, X., Nimtz, M., Rohde, M., Bokranz, W. & Römling, U. (2001). The multicellular morphotypes of Salmonella typhimurium and Escherichia coli produce cellulose as the second component of the extracellular matrix. Molecular Microbiology, 39, 1452–1463. doi: 10.1046/j.1365-2958.2001.02337.x