ABSTRACT
Infectious laryngotracheitis virus (ILTV) has a high proclivity to replicate in the larynx and trachea of chickens causing severe lesions. There is a lack of knowledge on the ability of ILTV to replicate in other respiratory associated tissues apart from in the trachea. The objective of this study was to investigate how tissues that first encounter the virus dictate further sites of viral replication during the lytic stage of infection. Replication patterns of the pathogenic strain 63140 and the chicken embryo origin (CEO) vaccine in the conjunctiva, the Harderian gland, nasal cavity and trachea were evaluated after ocular, oral, intranasal or intratracheal inoculation of specific pathogen-free chickens. Viral replication was assessed by detection of microscopic cytolytic lesions, detection of viral antigen and viral genome load. The route of viral entry greatly influenced virus replication of both strain 63140 and CEO vaccine in the conjunctiva and trachea, while replication in the nasal cavity was not affected. In the Harderian gland, independently of the route of viral entry, microscopic lesions characteristic of lytic replication were absent, whereas viral antigen and viral genomes for either virus were detected, suggesting that the Harderian gland may be a key site of antigen uptake. Findings from this study suggest that interactions of the virus with the epithelial-lymphoid tissues of the nasal cavity, conjunctiva and the Harderian gland dictate patterns of ILTV lytic replication.
Introduction
Infectious laryngotracheitis (ILT) is a highly contagious acute respiratory disease of chickens that results in severe losses due to mortality and/or decrease in egg production. The disease is caused by the avian alphaherpesvirus Gallid herpesvirus 1 (GaHV-1) commonly known as infectious laryngotracheitis virus (ILTV). Independent studies aimed at assessing the levels of pathogenicity among field isolates and vaccines found that evaluation of microscopic lesions in the trachea after intratracheal (IT) inoculation did not necessarily reflect the severity of the clinical presentation. When vaccines were administered intratracheally, although no severe clinical signs were observed, a few birds showed severe microscopic lesions in the trachea (Guy et al., Citation1990). After IT inoculation, microscopic lesions in the trachea were not reliable indicators of pathogenicity since isolates that induced high mortality rates produced moderate pathological changes in the trachea (Kirkpatrick et al., Citation2006). Furthermore, in two independent studies after intratracheal inoculation of the standard USDA challenge strain and the more recent outbreak-related ILTV strains, the presence of tracheal lesions did not necessarily reflect clinical signs of disease (Koski et al., Citation2015); also, neither microscopic lesions in the trachea nor clinical signs revealed differences in pathogenicity between strains (Vagnozzi et al., Citation2015). On the other hand, when various US field isolates were inoculated by the ocular-nasal route, differences in disease severity and viral tissue distribution were revealed (Oldoni et al., Citation2009), suggesting that the route of viral entry significantly influences the outcome of infection.
ILT is mainly controlled by vaccination with either live attenuated or recombinant viral vector vaccines (García et al., Citation2013). There are two types of live attenuated ILTV vaccines used to control the disease: vaccines of tissue culture origin (TCO) and vaccines of chicken embryo origin (CEO) (García et al., Citation2013). The CEO vaccines are recommended for mass administration by drinking water and spray, or for individual administration by eye drop vaccination while the TCO vaccine is recommended for use solely by eye drop administration (Gelenczei & Marty, Citation1964). The method of administration of live attenuated vaccines plays a crucial role in the degree of protection elicited by the vaccination (Fulton et al., Citation2000). Despite this knowledge, how the route of viral entry influences viral replication in the upper respiratory tract and in mucosal tissues of the nasal cavity, conjunctiva and the Harderian gland has not been studied. The nasal cavity, conjunctiva and the Harderian gland are structures that, although not anatomically connected to the respiratory system, together with the trachea are the first to come into contact with the virus during vaccination or natural infection (Härtle & Kaspers, Citation2014). In this study, potential routes of viral entry, accessible during mass vaccination or natural infection, were reproduced by individually inoculating chickens via the ocular (OC), intranasal (IN), oral (OR) or IT routes. The specific objective of this study was to determine how the route of viral entry influences the pattern of replication of the pathogenic strain 63140 and the CEO vaccine. Viral replication in tissues was assessed by detection of microscopic lesions characteristic of ILTV lytic replication, detection of viral antigen by immunohistochemistry (IHC) and quantification of the viral genome load. Findings from this study showed that the route of inoculation greatly influences the replication patterns of ILTV and revealed that tissues of the nasal cavity and conjunctiva, as well as the Harderian gland play a key role in early virus replication and consequently will dictate the outcome of infection.
Materials and methods
ILTV strains
The Georgia broiler strain 63140 (Vagnozzi et al., Citation2015) and the CEO vaccine (Trachivax® MERCK, Animal Health, NJ, USA) were utilized in this study. The pathogenic strain 63140 was propagated in chicken kidney cells obtained from 3- to 4-week-old specific pathogen-free (SPF) chickens (Rodriguez-Avila et al., Citation2008). The CEO vaccine was reconstituted as recommended by the manufacturers. After inoculation, both viruses were titrated in chicken kidney cells as previously described (Rodriguez-Avila et al., Citation2008) to confirm the median tissue culture infective dose (TCID50) administered.
Experimental design
Two independent studies were performed. In study I the replication of the pathogenic strain 63140 was examined and in study II the replication of the CEO vaccine was evaluated. Replication in the trachea, conjunctiva, nasal cavity and the Harderian gland was evaluated when administered via the OC, OR, IN or IT routes. SPF fertile eggs were obtained from Sunrise (Stuarts Draft, VA, USA), and incubated for a total of 506 h at the Poultry Diagnostic Research Center, University of Georgia hatchery facilities.
Study I
A total of 128 SPF chickens were divided into eight groups of 16 chickens per group. Chickens were housed in isolation units, eight chickens per unit, with filtered air and negative pressure, and provided with feed and water ad libitum. At three weeks of age four groups of chickens were inoculated with the pathogenic strain 63140 by the OC, OR, IN or IT route. Four additional groups of chickens were mock inoculated with cell culture media by the same routes and used as negative controls. All groups of chickens received a dose of 103.5 TCID50 per chicken. The OC and IN inoculated groups of chickens received the dose in a 60 μl volume, 30 μl per eye or per naris, respectively. The IT and OR inoculated groups of chickens received the dose in a 100 μl volume. For OR inoculation the inoculum was placed in the palatine cleft of chickens. The mock-inoculated groups of chickens received cell culture media in a similar manner.
Study II
A total of 195 SPF one-day-old chickens were divided into eight groups of 26 to 24 chickens per group and housed and fed as described above. At two weeks of age four groups of chickens were inoculated with the CEO vaccine by the OC, OR, IN or IT routes as described above. Four additional groups of chickens were mock inoculated with cell culture media by OC, OR, IN or IT routes and served as negative controls. All groups inoculated with the CEO vaccine received five times (5×) the recommended commercial dose.
All bird experiments conducted in this study were performed under the Animal Use Proposal A2015 05-001-Y2-A0 approved by the Animal Care and Use Committee (IACUC) in accordance with regulations of the Office of the Vice President for Research at the University of Georgia.
Tissue collection
The conjunctiva, nasal cavity swabs, trachea and the Harderian gland were collected from four (Study I) and six chickens (Study II) per group at 3 and 5 days post-inoculation for PCR analysis. Sterile polyester-tipped applicators (Puritan®, Guilford, ME, USA) were utilized to collect samples from conjunctiva by rubbing the internal surface of the eyelids. Nasal cavity samples were collected from the ciliated pseudo-stratified columnar epithelium of the nasal cavity after making a transversal cut at half way down the beak and wiping the nasal cavity 3–4 times with a swab. A one-inch transverse section of the proximal trachea was aseptically dissected and fat and connective tissue removed from the serosa. Collection of the Harderian gland was performed as previously described (Neumann, Citation1976). Conjunctiva and nasal cavity swabs, trachea and Harderian gland tissues were immediately placed in tubes containing 1.4 mm ceramic spheres (Lysing matrix D, MP Biomedicals, Santa Ana, CA, USA) with 2 ml of sterile phosphate-buffered saline solution (0.0067M PO4 pH 7.0-7.2, Hyclone), 2% antibiotic–antimycotic and 2% foetal bovine serum (Atlanta® Biologicals, Flowery Branch, GA. USA) and stored at −80°C until processed for DNA extraction.
For histopathological examination the trachea, conjunctiva, nasal cavity and the Harderian gland were collected at three and five days post-inoculation from four chickens (Study I) and seven to six chickens (Study II) per group. The trachea and the Harderian gland were collected as described above, the conjunctiva was collected by making a transversal cut of the upper and lower eyelids (left and right) and the nasal cavity was obtained by making a transversal cut of the distal portion of the beak, followed by a cut of the central nasal fossa. All tissues were immediately placed in 10% buffered neutral formalin and fixed for 24 h.
Histopathology
Four-micrometre sections from the formalin-fixed, paraffin-embedded, trachea, conjunctiva, nasal cavity and Harderian gland (right and left) were made and stained with haematoxylin and eosin (H&E). All H&E stained sections were examined for the presence of microscopic lesions typical of ILTV cytolitic replication (syncytial cell formation and intranuclear inclusion bodies).
Immunohistochemistry
Detection of ILTV antigen was performed in tissue sections where microscopic lesions typical of ILTV lytic replication were disputable. Viral antigen was detected by immunohistochemistry (IHC) staining of formalin-fixed, paraffin-embedded tissue sections using ILTV monoclonal antibody (Guy et al., Citation1992). Briefly, deparaffinized 4 μm tissue sections were placed on positively charged slides. Sections were incubated with 3% hydrogen peroxide for 5 min to block endogenous peroxidase activity, followed by incubation with Proteinase K (Dako, Carpinteria, CA, USA) for five minutes at room temperature. Between 3% hydrogen peroxide and Proteinase K treatments, sections were washed three times with a 1:10 phosphate-buffered saline (PBS) (NaPO4 monobasic, NaPO4 dibasic and NaCl, pH 7.2) for 1 min per rinse. Sections were then incubated with a 1:3000 dilution of ILTV monoclonal antibody in phosphate-buffered saline (Guy et al., Citation1992) and incubated at room temperature in a humidity chamber for one hour. Sections were then incubated with a secondary antibody mouse and rabbit UnoVue™ polymer (Diagnostic BioSystem, Pleasanton, CA, USA) for 15 min. After incubation with primary and secondary antibody, tissue sections were washed five times with a 0.1% solution of Tween® 20 (Sigma-Aldrich®, St. Louis, MO, USA) in PBS for one min per wash. Finally, diaminobenzidene (Diagnostic Biosystem) was added to the sections according to manufacturer’s instructions and incubated for 5 min at room temperature. Sections were carefully washed using distilled H2O, counterstained with Gill’s haematoxylin and 1% ammonium hydroxide for 1 min, dehydrated and mounting media (Flo-Texx®, Thermo Fisher Scientific, Waltham, MA, USA) was added for microscopic examination. A brown precipitate indicative of the peroxidase-conjugated antibody reaction with the diaminobenzidene substrate was observed in tissue sections positive for ILTV antigen. The conjunctiva, nasal cavity and trachea tissues with unequivocal microscopic lesions characteristic of ILTV cytolytic replication and sections where ILTV antigen was detected by IHC were tallied and reported as percentages. All the Harderian gland sections were stained by IHC. Cells in the Harderian gland positive for ILTV antigen were counted. The average number of ILTV-positive cells per gland was estimated for groups of chickens inoculated with 63140 or CEO via the OC, OR, IN or IT routes.
DNA extraction
Total DNA was extracted from the trachea, eye-conjunctiva swabs, nasal cavity swabs and the Harderian glands using the MegaZorb® DNA extraction mini-prep 96-well kit (Promega, Madison, WI, USA) as previously described by Vagnozzi et al. (Citation2010).
Duplex real-time PCR
Duplex real-time PCR assay that amplifies a fragment of the UL44 viral gene and a fragment of the chicken α2-collagen gene was performed as previously described (Vagnozzi et al., Citation2012). Briefly, each sample was normalized to the chicken α2-collagen gene and the relative amount of viral DNA in each sample was estimated as ΔΔCt = ΔCt ILTV inoculated – ΔCt mock inoculated expressed as log10 2-ΔΔCt.
Statistical analysis
The one-way analysis of variance Kruskal–Wallis test (P < 0.05) was utilized to determine the effect of route of inoculation on viral replication. The genome loads of 63140 and CEO vaccine for individual tissues were compared among routes (OC, OR, IN, IT) at days 3 and 5 post-inoculation.
The non-parametric Mann–Whitney U-test (P < 0.05) was utilized to emphasize differences in the number of ILTV antigen-positive cells in the Harderian gland from groups of chickens inoculated with either 63140 or CEO vaccine via the OC, OR, IN or IT route. The statistical analysis software used was the GraphPad PRISM 5.0 (GraphPad Software, Inc., La Jolla, CA) http://www.graphpad.com/company/.
Results
Histopathology examination and IHC in the conjunctiva, nasal cavity and trachea
The percentage of conjunctiva, nasal cavity and trachea with unequivocal microscopic lesions characteristic of ILTV lytic replication (syncytial cell formation, and intranuclear inclusion bodies) and tissue sections where ILTV antigen was detected by IHC were tallied and reported as a percentage (). At five days post-inoculation via the OC route with strain 63140 evidence of lytic replication was observed in 100% of the conjunctiva samples collected ((a)). For groups of chickens inoculated with the CEO vaccine via the OC route, vaccine replication in the conjunctiva was detected in 64% and 21% of the tissues collected at days three and five post-inoculation, respectively ((b)). After IT inoculation replication of either 63140 or the CEO vaccine in the conjunctiva was absent, while replication of either virus was evident in the tracheas collected at three and five days post-inoculation (). After OC inoculation, replication in the trachea was only detected in chickens inoculated with pathogenic strain 63140 ((c)) but not in chickens inoculated with the CEO vaccine ((d)).
Figure 1. Haematoxylin and eosin (H&E) staining of conjunctiva and trachea sections of chickens inoculated with ILTV pathogenic strain 63140 ((a,c,e,g)) or CEO vaccine strain ((b,d,f,h)) and collected at five days post-inoculation. Presence of syncytial cell formation and intranuclear inclusion bodies in the conjunctiva ((a)) and trachea ((c)) of chickens inoculated with strain 63140 via the OC route, and in the conjunctiva of chickens inoculated with the CEO strain via the OC ((b)) and IN ((f)) routes. Absence of lesions in the conjunctiva ((e)) and trachea ((g)) of 63140-inoculated chickens and in the trachea ((h)) and conjunctiva ((d)) of chickens inoculated with the CEO strain via the IN and OC routes, respectively.
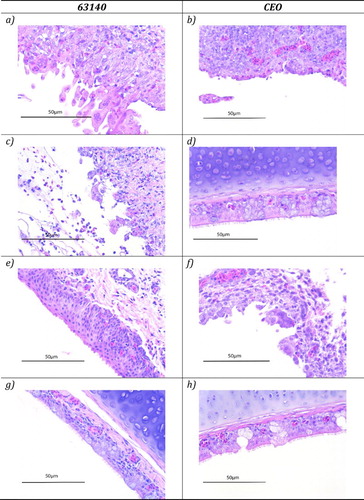
Table 1. Percentage of ILTV-positive samples as determined by histopathology examination and IHC.
Evidence of cytolytic replication was detected for both viruses after five days post-inoculation in the nasal cavity independently of the route of inoculation (). However, after OR or IN inoculation with 63140 no viral lytic replication was observed in the conjunctiva ((e)) and trachea ((g)). In groups of chickens inoculated with the CEO vaccine strain via the OR or IN routes, absence of viral replication was observed in the trachea ((h)), while replication in the conjunctiva was detected when the vaccine was administered via the OR (15%) or IN (25%) routes of inoculation ((f)).
Microscopic examination and IHC staining of the Harderian gland
Neither microscopic lesions characteristic of ILTV lytic replication nor massive lymphocytic infiltrations were identified in the Harderian gland after inoculation via the OC, OR, IN or IT routes with either 63140 ((a)) or CEO ((b)). Instead ILTV antigen was detected in the Harderian gland from chickens inoculated with 63140 or CEO vaccine. ILTV antigen was detected in cells scattered throughout the stroma of the gland ((c,d)).
Figure 2. Haematoxylin and eosin (H&E) and immunohistochemistry (IHC) staining of the Harderian gland sections collected at five days post-inoculation of chickens that received ILTV pathogenic strain 63140 or the CEO vaccine strain via the OC route. Absence of syncytial cell formation, intranuclear inclusion bodies and lymphocyte infiltration in the Harderian gland sections ((a,b)). Viral antigen detection in the Harderian gland sections ((c,d)).
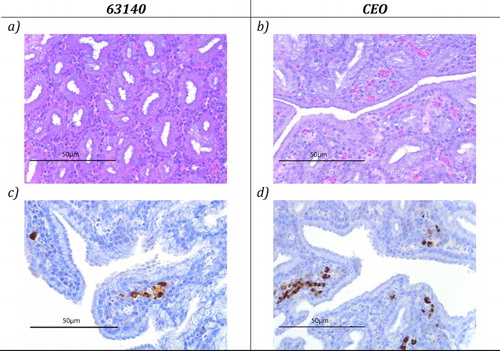
The average number of ILTV antigen-positive cells per Harderian gland was tallied (). Independently of the route of inoculation, no differences in the number of ILTV antigen-positive cells were found in Harderian gland from chickens inoculated with either the 63140 strain or the CEO vaccine.
Table 2. Mean number of ILTV antigen-positive cells per Harderian gland.
Viral genome load
As expected no viral genome load was detected in mock-inoculated groups of chickens (data not shown). shows the individual genome load of 63140 ((a–d)) and CEO ((e–h)) at days 3 and 5 post-inoculation in conjunctiva ((a,e)), nasal cavity ((b,f)), the Harderian gland ((c,g)) and trachea ((d,h)) when chickens received the viruses via the OC, OR, IN or IT routes. Genome load per tissue was compared among routes of inoculation for each virus. Genome load in conjunctiva at day five post-inoculation in chickens inoculated with the 63140 strain via the OC route was higher but not statistically different to the genome load detected in the conjunctiva from chickens that received the virus via the OR, IN or IT routes. Chickens that received the CEO vaccine via the OC route showed significantly high (P < 0.05) genome load in conjunctiva at days 3 and 5 post OC inoculation ((e)), while chickens inoculated via the OR route showed significantly high (P < 0.05) genome load in the nasal cavity ((f)). In both instances, CEO genome load correlated with the presence of lesions in the conjunctiva and nasal cavity epithelium. The levels of 63140 and CEO genome load in the nasal cavity of chickens inoculated via the OC, IN and IT did not reflect the microscopic lesions detected in the epithelium of the nasal cavity. Groups of chickens inoculated intratracheally with either 63140 or CEO showed significantly higher (P < 0.05) genome load in the trachea than when administered via the OC, OR or IN routes ((d,h)) which reflected the presence of tracheal lesions. Genome load of 63140 in the Harderian gland was no different among chickens inoculated by the OC, OR, IN or IT routes ((c)). However, in the Harderian gland of chickens that received the CEO vaccine via the OR route the genome load of the vaccine was significantly higher (P < 0.05) than that detected in groups of chickens that received the vaccine via the OC, IN and IT routes.
Figure 3. Individual genome load of the pathogenic ILTV strain 63140 and CEO vaccine. Genome loads (Log10 2−ΔΔCt) of 63140 ((a–d)) and CEO vaccine ((e–h)) at 3 and 5 days post-inoculation. Genome loads of 63140 and CEO vaccine for each tissue were compared among groups of birds inoculated via the ocular (OC), oral (OR), intranasal (IN) or intratracheal (IT) route by the one-way analysis of variance Kruskal–Wallis test. A significant genome load decrease (P < 0.05) is indicated by a lambda symbol (λ) and a significant genome load increase (P < 0.05) is indicated by an asterisk (*). The horizontal line depicts the mean genome load per tissue and the vertical line depicts the standard deviation in the group.
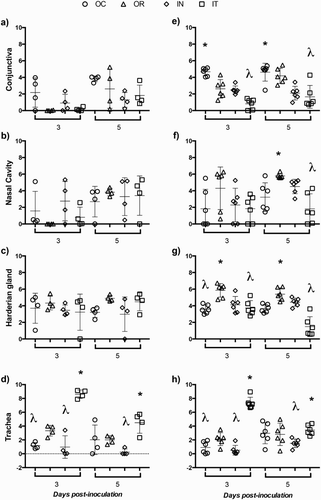
Discussion
After exposure to ILTV, microscopic changes in the respiratory epithelium and conjunctiva varied with the stage of infection. Early microscopic changes in the trachea mucosa are observed by two days post-infection, including the loss of goblet cells and infiltration of the mucosa by inflammatory cells. As the viral infection progresses, epithelial cells of the trachea and the conjunctiva enlarge and become oedematous. Multinucleated cells (syncytia) and inclusion bodies are formed and lymphocytes, histiocytes and plasma cells migrate into the mucosa and sub-mucosa of the trachea epithelium, lesions that are characteristic of ILTV lytic infection. Inclusion bodies disappear as infection progresses due to necrosis and desquamation of the epithelium (García et al., Citation2013). Several studies have shown that after intratracheal inoculation with pathogenic ILTV strains, moderate to severe lesions, as those described above, are produced in the trachea mucosa (Guy et al., Citation1990; Kirkpatrick et al., Citation2006; Vagnozzi et al., Citation2015), while live attenuated vaccines produce milder lesions in the trachea (Guy et al., Citation1990).
Similar to in the trachea, after IN inoculation with pathogenic ILTV strains, viral replication in the nasal cavity produced acute sloughing, desquamation and formation of syncytia in the epithelium (Bang & Bang, Citation1967; Purcell & McFerran, Citation1969), while milder lesions were detected in the nasal epithelium after administration of the SA2 vaccine by drinking water, eye drop (Robertson & Egerton, Citation1981) or spray (Clarke et al., Citation1980). In all instances replication of the SA2 vaccine strain was rarely detected in the larynx and was absent in the trachea.
The conjunctiva, eyes and nasal cavity contain structures like the conjunctiva-associated lymphoid tissue (CALT) (van Ginkel et al., Citation2012), the paranasal gland, the nasal-associated lymphoid tissue (NALT) (Kang et al., Citation2013; de Geus et al., Citation2015) and the paraocular Harderian gland (van Ginkel et al., Citation2009). These structures, although not anatomically connected to the respiratory system, harbour organized lymphoid follicles and intraepithelial lymphocytes which act as innate barriers to antigens (Härtle & Kaspers, Citation2014). Upon exposure to the antigen, strong mucosal and systemic immune responses are generated in the Harderian gland (van Ginkel et al., Citation2009) and CALT (van Ginkel et al., Citation2012). The lymphoid epithelium in the CALT (Fix & Arp, Citation1991) and NALT (Kang et al., Citation2013) has the ability to uptake antigens. Full maturity of the CALT was manifested by an increased particle uptake as early as three weeks of age (van Ginkel et al., Citation2012), while NALT was considered fully developed by two weeks of age (Kang et al., Citation2013). When fully developed, the uptake of antigen by the NALT was significantly more effective than antigen uptake by the CALT (de Geus et al., Citation2015).
In the field, when chickens are placed in an infected environment they are immediately exposed to ILTV through multiple routes, OR, nasal, OC and IT, by ingesting infected material or inhaling aerosol virus-containing droplets. In this study, we wanted to control the route of viral entry to investigate how tissues that first encounter the virus dictate further sites of viral replication during the lytic stage of infection. The viral dose necessary to elicit lesions indicative of lytic infection in the trachea has been previously determined for 63140 (Vagnozzi et al., Citation2015) but is not known for the CEO vaccine. In this experiment the CEO vaccine was administered at a 5× dose per bird to increase the likelihood of detecting lesions characteristic of lytic infection by the vaccine virus. Although it is known that maturation of CALT and NALT is age dependent, in these experiments SPF birds were inoculated with the CEO vaccine at two weeks of age since in the field vaccination at three weeks of age is not practised, while inoculation with 63140 was performed at three weeks of age to prevent increased mortality at an early age.
Results from this experiment clearly showed that the route of viral entry greatly influenced replication patterns of the pathogenic strain 63140 and the CEO vaccine in the trachea and conjunctiva but not in the nasal cavity. Both viruses showed a high proclivity to replicate in the conjunctiva epithelium when administered via the OC route as indicated by ILTV cytolytic lesions. It is possible that after administration via the OC route the decreased ability of the CALT to uptake antigen allowed the virus easy access to permissive epithelial cells favouring lytic replication in the conjunctiva. However, when strain 63140 was administered via the OR or IN routes replication in the conjunctiva and trachea was absent. One possibility is that through OR or IN routes of viral entry the virus is propelled into the NALT where local immune responses will eventually diminish lytic replication in the conjunctiva and trachea. In contrast to chickens inoculated with 63140, microscopic lesions were detected in the conjunctiva of chickens inoculated via the OR or IN routes with the CEO vaccine, an indication that the CALT of two-week-old chickens may not be able to halt virus replication in the conjunctiva. On the other hand, the absence of microscopic lesions in the trachea after OC, OR or IN inoculation suggests that interaction of the CEO vaccine with a mature NALT eventually prevented the establishment of lytic replication in the trachea.
In contrast to histological changes induced by the Newcastle disease virus (Survashe et al., Citation1979) and infectious bronchitis virus (Toro et al., Citation1996) no evidence of lesions characteristic of ILTV lytic replication was detected in the Harderian gland where only viral antigen and nucleic acid were detected. Therefore, during ILTV infections the Harderian gland in the absence of tissue damage may function as a key site of virus uptake. Further analysis is warranted to identify the cells in the gland that uptake ILTV antigen.
Viral genome load was a reliable indicator of lytic viral replication only in the trachea when viruses were administered intratracheally, in the conjunctiva when the CEO vaccine was administered via the OC route, or in the nasal cavity when the CEO vaccine was administered via the OR route. However, when strain 63140 was administered via OC, OR or IN routes moderate loads of viral genomes were detected in the conjunctiva and nasal cavity which did not reflect the level of viral lytic replication lesions found in these sites. The increased incidence of microscopic lesions in the conjunctiva and the nasal cavity may be an indicator of the pathogenicity of strain 63140.
Overall the route of inoculation, particularly viral entry through the OC, OR and IN routes, greatly influenced lytic replication patterns of pathogenic strain 63140 and the CEO vaccine in the trachea and conjunctiva. Findings from this study suggest that interactions of the virus with the epithelial-lymphoid tissues of the nasal cavity, conjunctiva and the Harderian gland would dictate patterns of ILTV lytic replication, which consequently will determine the outcome of infection. Furthermore, this study suggests that after mucosal administration (OR, OC and IN) lytic replication of the CEO vaccine in the trachea may not be necessary to induce protection. Further studies then are warranted to examine interactions of the CEO vaccine with cells of the CALT and NALT in order to unmask the mechanism by which CEO elicits a local immune response in these sites. Subsequently this information will aid in the development of new live attenuated vaccines against the disease.
Disclosure statement
No potential conflict of interest was reported by the authors.
Additional information
Funding
References
- Bang, B.G. & Bang, F.B. (1967). Laryngotracheitis virus in chickens. A model for study of acute nonfatal desquamating rhinitis. Journal of Experimental Medicine, 125, 409–428. doi: 10.1084/jem.125.3.409
- Clarke, J.K., Robertson, G.M. & Purcell, D.A. (1980). Spray vaccination of chickens using infectious laryngotracheitis virus. Australian Veterinary Journal, 56, 424–428. doi: 10.1111/j.1751-0813.1980.tb02635.x
- de Geus, E.D., Degen, W.G., van Haarlem, D.A., Schrier, C., Broere, F. & Vervelde, L. (2015). Distribution patterns of mucosally applied particles and characterization of the antigen presenting cells. Avian Pathology, 44, 222–229. doi: 10.1080/03079457.2015.1026797
- Fix, A.S. & Arp, L.H. (1991). Particle uptake by conjunctiva-associated lymphoid tissue (CALT) in turkeys. Avian Diseases, 35, 100–106. doi: 10.2307/1591301
- Fulton, R.M., Schrader, D.L. & Will, M. (2000). Effect of route of vaccination on the prevention of infectious laryngotracheitis in commercial egg-laying chickens. Avian Diseases, 44, 8–16. doi: 10.2307/1592502
- García, M., Spatz, S. & Guy, J. (2013). Infectious laryngotracheitis. In D.E. Swayne, J.R. Glisson, L.R. McDougald, L.K. Nolan, D.L. Suarez & V.L. Nair (Eds.), Diseases of Poultry (13th ed., pp. 161–179). Ames, Iowa: Wiley-Blackwell.
- Gelenczei, E.F. & Marty, E.W. (1964). Studies on a tissue-culture-modified infectious laryngotracheitis virus. Avian Diseases, 8, 105–122. doi: 10.2307/1587827
- Guy, J.S., Barnes, H.J. & Morgan, L.M. (1990). Virulence of infectious laryngotracheitis viruses: comparison of modified-live vaccine viruses and north carolina field isolates. Avian Diseases, 34, 106–113. doi: 10.2307/1591340
- Guy, J.S., Barnes, H.J. & Smith, L.G. (1992). Rapid diagnosis of infectious laryngotracheitis using a monoclonal antibody-based immunoperoxidase procedure. Avian Pathology, 21, 77–86. doi: 10.1080/03079459208418820
- Härtle, S. & Kaspers, B. (2014). The avian respiratory immune system. In K.A. Schat, B. Kaspers & P. Kaiser (Eds.), Avian Immunology (2nd ed., pp. 251–263). Boston, MA: Academic Press.
- Kang, H., Yan, M., Yu, Q. & Yang, Q. (2013). Characteristics of nasal-associated lymphoid tissue (NALT) and nasal absorption capacity in chicken. PLoS One, 8, e84097. doi: 10.1371/journal.pone.0084097
- Kirkpatrick, N.C., Mahmoudian, A., Colson, C.A., Devlin, J.M. & Noormohammadi, A.H. (2006). Relationship between mortality, clinical signs and tracheal pathology in infectious laryngotracheitis. Avian Pathology, 35, 449–453. doi: 10.1080/03079450601028803
- Koski, D.M., Predgen, A.S., Trampel, D.W., Conrad, S.K., Narwold, D.R. & Hermann, J.R. (2015). Comparison of the pathogenicity of the USDA challenge virus strain to a field strain of infectious laryngotracheitis virus. Biologicals, 43, 232–237. doi: 10.1016/j.biologicals.2015.05.005
- Neumann, U. (1976). The surgical removal of the Harderian gland of the hen. Zentralblatt Für Veterinärmedizin. Reihe A, 23, 323–330. doi: 10.1111/j.1439-0442.1976.tb01708.x
- Oldoni, I., Rodriguez-Avila, A., Riblet, S.M., Zavala, G. & García, M. (2009). Pathogenicity and growth characteristics of selected infectious laryngotracheitis virus strains from the United States. Avian Pathology, 38, 47–53. doi: 10.1080/03079450802632031
- Purcell, D.A. & McFerran, J.B. (1969). Influence of method of infection on the pathogenesis of infectious laryngotracheitis. Journal of Comparative Pathology, 79, 285–291. doi: 10.1016/0021-9975(69)90041-3
- Robertson, G.M. & Egerton, J.R. (1981). Replication of infectious laryngotracheitis virus in chickens following vaccination. Australian Veterinary Journal, 57, 119–123. doi: 10.1111/j.1751-0813.1981.tb00472.x
- Rodriguez-Avila, A., Oldoni, I., Riblet, S. & García, M. (2008). Evaluation of the protection elicited by direct and indirect exposure to live attenuated infectious laryngotracheitis virus vaccines against a recent challenge strain from the United States. Avian Pathology, 37, 287–292. doi: 10.1080/03079450802043742
- Survashe, B.D., Aitken, I.D. & Powell, J.R. (1979). The response of the Harderian gland of the fowl to antigen given by the ocular route. I. Histological changes. Avian Pathology, 8, 77–93. doi: 10.1080/03079457908418329
- Toro, H., Godoy, V., Larenas, J., Reyes, E. & Kaleta, E.F. (1996). Avian infectious bronchitis: viral persistence in the Harderian gland and histological changes after eyedrop vaccination. Avian Diseases, 40, 114–120. doi: 10.2307/1592380
- Vagnozzi, A., García, M., Riblet, S. & Zavala, G. (2010). Protection induced by infectious laryngotracheitis virus vaccines alone and combined with Newcastle disease virus and/or infectious bronchitis virus vaccines. Avian Diseases, 54, 1210–1219. doi: 10.1637/9362-040710-Reg.1
- Vagnozzi, A., Riblet, S.M., Williams, S.M., Zavala, G. & García, M. (2015). Infection of broilers with two virulent strains of infectious laryngotracheitis virus: criteria for evaluation of experimental infections. Avian Diseases, 59, 394–399. doi: 10.1637/11075-033115-Reg.1
- Vagnozzi, A., Riblet, S.M., Zavala, G. & García, M. (2012). Optimization of a duplex real-time PCR method for relative quantitation of infectious laryngotracheitis virus. Avian Diseases, 56, 406–410. doi: 10.1637/9883-081111-ResNote.1
- van Ginkel, F.W., Gulley, S.L., Lammers, A., Hoerr, F.J., Gurjar, R. & Toro, H. (2012). Conjunctiva-associated lymphoid tissue in avian mucosal immunity. Developmental & Comparative Immunology, 36, 289–297. doi: 10.1016/j.dci.2011.04.012
- van Ginkel, F.W., Tang, D.C., Gulley, S.L. & Toro, H. (2009). Induction of mucosal immunity in the avian Harderian gland with a replication-deficient ad5 vector expressing avian influenza h5 hemagglutinin. Developmental & Comparative Immunology, 33, 28–34. doi: 10.1016/j.dci.2008.07.018