ABSTRACT
Marek’s disease is a lymphoproliferative disease causing a serious threat in poultry production. Field strains of Marek’s disease virus (MDVs) are continuously re-emerging, causing great economical losses to the poultry industry worldwide in spite of the intensive vaccination and restrictive management policy used. Histopathological and molecular characterizations of MDVs are essential for monitoring the changes of viruses and evaluating the effectiveness of existing vaccines. During 2016, 190 visceral tumour tissues representing 30 vaccinated chicken flocks from the Gifu prefecture, Japan, were analysed. A pathological examination revealed the presence of lymphoproliferative lesions in the visceral organs. Polymerase chain reaction screening of tissue specimens using specific primers for avian leucosis virus, reticuloendotheliosis virus, and MDV was positive only for MDV. The polymerase chain reaction products of meq, pp38, virus-induced IL-8 homology, and glycoprotein MDV genes were sequenced and used for homology, phylogenetic, and similarity level analysis with the published reference of MDVs in the database. The results revealed high similarity between the field isolates, vv and vv+ strains of MDV from the USA and China. Several point mutations in the nucleotide sequence of the field isolates and their deduced amino acid sequences were detected in those genes. The present molecular analyses indicated that nucleotide and amino acid changes could be valuable criteria for differentiation and determination of the pathogenicity and oncogenicity of MDVs according to the Avian Disease and Oncology Laboratory pathotyping in vivo studies. Furthermore, the results suggest that development of a new vaccine must be considered to overcome this devastating avian oncogenic viral disease.
Introduction
Marek’s disease (MD) is an infectious re-emerging viral disease of chickens caused by Marek’s disease virus (MDV), Gallid herpesvirus II (Payne & Rennie, Citation1976; Buckmaster et al., Citation1988), inducing neoplastic infections in poultry in the form of T-cell lymphomas in the visceral organs and peripheral nerves (Ding et al., Citation2007).
In recent years, the incidence of MD has commonly increased in many countries, including China (Tian et al., Citation2011), Egypt (Hassanin et al., Citation2013), India (Raja et al., Citation2008), Europe (Woźniakowski et al., Citation2014, Citation2015), and Japan (Murata et al., Citation2013), resulting in considerable economic losses in the poultry flocks.
MD has been well controlled since 1970 (Okazaki et al., Citation1970; Witter, Citation1972) by using the herpesvirus of turkey (HVT) vaccine, and then by a bivalent vaccine (a mixture of HVT and the serotype 2 strain SB-1), which was introduced in the mid-1980s (Witter & Lee, Citation1984). Finally, the “Rispens” vaccine (attenuated serotype 1 strain CVI988) (Rispens et al., Citation1972) was introduced for widespread use in the 1990s with further increase in the virulence of field virus strains (De Boer et al., Citation1986). All the above-mentioned vaccines are still in use in poultry farms since their introduction. The virulence and MD-associated losses in vaccinated flocks have subsequently increased (Witter, Citation1983, Citation1997) despite an intensive vaccination policy using the above-mentioned vaccines. Emergence of hypervirulent strains with a further increase in the virulence of field virus strains (Wozniakowski et al., Citation2010; Gong et al., Citation2013; Hassanin, et al., Citation2013) was suggested as the main cause of this vaccination failure.
The MDV genome encodes more than 200 genes, and several genes unique for oncogenic MDVs have been identified to date (Lee et al., Citation2000; Lupiani et al., Citation2004). The gene encoding a major lytic and transformation maintaining phosphoprotein antigen (pp38) together with the Marek’s EcoRI-Q-encoded protein (meq) and virus-induced IL-8 homology (vIL-8) genes were reported to play a role in viral oncogenicity and pathogenicity (Tian et al., Citation2011). The Meq protein is one of the most important MDV proteins that is only present in MDV-1 strains; it is highly expressed in MDV-1-transformed cell lines and tumour samples (Jones et al., Citation1992). Recently, meq gene mutation has attracted attention as a possible cause for the increased oncogenicity (Shamblin et al., Citation2004; Wozniakowski et al., Citation2010; Woźniakowski et al., Citation2014), although many other genes also play important roles in the development of lymphomas (Jarosinski et al., Citation2006).
pp38 is required for the induction of cytolytic infection in B lymphocytes and for the production of adequate levels of latently infected T lymphocytes in the lymphoid organs. In addition, pp38 has been shown to play a role in maintaining the transformation of T lymphocytes by preventing their apoptosis (Gimeno et al., Citation2005). Other reports have indicated a potential role of pp38 in immunomodulating the immune response against MDV. Herpesvirus glycoproteins such as that encoded by the surface glycoprotein gene (gL) mediate the fundamental aspects of infection, including initial attachment, membrane fusion, virion penetration, trafficking of virion components, virion assembly, and cell-to-cell spread (Rajcani & Vojvodova, Citation1998).
The vIL-8 gene is involved in early cytolytic infections in lymphoid organs, presumably the recruitment of B or T lymphocytes in vivo. It has a less impact on either virus reactivation from latency or virus shedding. Deletion of vIL-8 leads to weak activation of T cells, resulting in reduced numbers of target cells for transformation and significantly decreased pathogenicity and tumour incidence (Cui et al., Citation2004).
During 2016, an increased incidence of visceral lymphoma was detected in vaccinated broiler chicken flocks in the Gifu prefecture, Japan. Tumour tissue specimens from a suitable number (five to seven birds per flock) of tumour-bearing chickens were collected for histopathological and molecular evaluation.
In this study, in addition to morpho-pathological diagnosis, polymerase chain reaction (PCR) screening for MD, avian leucosis virus (ALV), and reticuloendotheliosis virus (REV) was performed.
The present study was conducted to document the up-to-date pathological alterations in Japanese poultry flocks that were spontaneously infected with MDV and to attempt to determine the causes of the recent increase in pathogenicity and virulence of this oncogenic virus, despite adherence to a strict vaccination policy. To achieve these objectives, we performed PCR identification and sequence analyses of these four unique MDV genes, meq, pp38, gL, and vIL-8, of field isolates obtained from chicken flocks vaccinated with HVT and CVI988/Rispens (the most effective currently used vaccine against MD). The association between enhanced virulence and common mutations in these major latency/transformation antigens was analysed.
Materials and methods
Sample collection and histopathological examination
Samples were collected during 2016 from 30 special broiler flocks of different breeds (62–80 days old) representing six farms in Gifu city, Japan (). All broilers were vaccinated by HVT and CVI988/Rispens and brought to the Pathology Laboratory, Faculty of Applied Biological Science of Gifu University, Japan, with a suspicion of infection with oncogenic viruses for necropsy and diagnosis.
Table 1. Description of selected MDV-infected flocks for Japanese samples assigned in the GenBank.
Chickens with progressive tumours in different visceral organs were sacrificed according to slaughterhouse guidelines in Japan and brought immediately after slaughtering to the laboratory for necropsy and diagnosis.
Five to seven chickens from each flock were immediately subjected to precise post-mortem examination, and all post mortem findings were recorded. Tissue specimens were immediately collected from all tumour-associated organs, including the liver, kidney, spleen, proventriculus, intestine, and sciatic nerves. A set of tissue specimens was immediately fixed in 10% neutral buffered formalin (average period of fixation was 3–4 days at room temperature) for histopathological and immunohistochemical examinations. Another set was collected and kept frozen at −20°C until use for DNA extraction and PCR for detection of the causative virus.
Histopathological and immunohistochemical examination
Fixed specimens were routinely processed through dehydration in ascending grades of ethanol, and then cleared in xylene and embedded in paraffin blocks. Some of the paraffin sections were stained with haematoxylin and eosin (H&E) for histopathological examinations and the rest were used for immunohistochemistry staining.
Immunostaining was performed using the EnVision+ System™ with a horseradish peroxidase-labelled polymer (Dako, Tokyo, Japan) (Sabattini et al., Citation1998). The label was visualized with the Liquid DAB + Substrate Chromogen System (Dako). Paraffin sections (4 μm) were prepared, dewaxed in xylene, and incubated with CD3 and CD20 primary antibodies (specific for T and B lymphocytes, respectively). Mouse-anti-human CD3 antibody (Sigma-Aldrich, St. Louis, MO, USA) was used at 1:3000 dilution and mouse-anti-human CD20 antibody (L26 clone; Dako, Carpinteria, CA, USA) was used at 1:200 dilutions. Antibody binding was visualized using the anti-mouse-IgG EnVision Kit™ (Dako). Sections were counterstained with Mayer's haematoxylin, mounted in aqueous mounting media, and examined under a light microscope. The immunostaining was performed at the Veterinary Pathology Laboratory, Gifu University, Japan.
DNA extraction
Total DNA and RNA extractions from the nerve and visceral tumour tissues, including the liver, spleen, kidney, and proventriculus, were performed according to Qiagen, AllPrep® DNA/RNA Mini kit (Hilden, Germany) manufacturer’s instructions. Total DNA and RNA were also extracted from the nerve and the same visceral organ tissues of the uninfected age-matched control birds from healthy farms that were reared under the same conditions.
PCR amplification of MDV genes
Each DNA extract was used as a template for PCR amplification using primers specific for the MDV meq, gL (Hassanin et al., Citation2013), pp38, and vIL-8 genes (Tian et al., Citation2011). The PCR amplification was performed in a 50 μl volume containing 4 μl of DNA, 4 μl of dNTPs, 1 μl (10 μmol) from each forward and reverse primer, 5 μl 10× Ex Taq buffer, 0.25 μl Ex Taq polymerase (Takara, Kyoto, Japan), and 34.75 μl distilled water. The thermal cycling for the meq gene was 95°C for 2 min followed by 40 cycles at 95°C for 30 s, 55°C for 30 s, and 72°C for 1 min, with a final extension at 72°C for 10 min. The meq-positive samples were used to amplify the UL1 (gL) sequence under the same amplification conditions. The meq-positive samples were also used for amplification of vIL-8 and pp38 genes under the following PCR condition: 94°C for 4 min; 35 cycles at 94°C for 1 min, 56°C for 1 min, 72°C for 1.5 min; and final elongation at 72°C for 10 min. All PCRs were carried out using the Takara Thermal Cycler® (Takara).
The 132-bp repeat region of the MDV-1 genome (Tian et al., Citation2011) is the target gene of PCR which can distinguish field MDV strain from vaccine “CVI988 strain.” The sequences of the primers used for this purpose were R1: 5′-ATG CGA TGA AAG TGC TAT GGA G-3′ and R2:5′-ATC CCT ATG AGA AAG CGC TTG A-3′.The PCR products were visualized and photographed in a 1% agarose gel containing 0.5 mg/ml ethidium bromide on a Gel Documentation System.
Molecular screening of MDV-positive samples for ALV and REV
To verify the absence of infection with ALV and REV in the chickens, all of the MDV-positive DNA samples were screened for the presence of REV and ALV using their specific previously published primers (Awad et al., Citation2010; Hassanin et al., Citation2013; Qin et al., Citation2013). The PCR condition for ALV detection was as follows: 95°C for 5 min; 35 cycles of 95°C for 30 s, 56°C for 30 s, and 72°C for 30 s; and a final extension at 72°C for 10 min. The PCR condition for REV detection was the same as that described above for the pp38 gene. Two DNA templates positive for REV and ALV (Awad et al., Citation2004, Citation2010) (kindly supplied by Dr Hatem, S. Abd El-Hamed, Faculty of Veterinary Medicine, Damanhour University, Egypt) were used as positive controls for REV and ALV infections, respectively. The MDV DNA positive template was supplied by Dr Tokuma Yanai and available at the Laboratory of Veterinary Pathology, Faculty of Applied Biological Sciences, Gifu University/Japan, which was used as a positive control for MDV.
DNA sequencing, sequence alignment, and phylogenic analysis
PCR amplicons were purified and prepared for sequencing using Qiaquick gel extraction kits® according to the manufacturer’s recommendations.
The purified PCR products of all MDV-positive samples were sequenced using the AB3500XL Genetic analyser (AB Applied Biosystems, HITACHI, Tokyo, Japan) at the genome centre of Gifu University, Japan, using gene-specific primers.
The obtained nucleotide sequences of the meq, pp38, vIL-8, and gL genes of MDV and the deduced amino acid sequences were edited using the Sequence Scanner Software 2 program (http://www.appliedbiosystems.com). Primer sequences were omitted prior to phylogenetic analysis. The primer sequences were omitted prior to phylogenetuc analysis; the edited sequences were compared with those of other reference MDVs for homology and phylogenetic analyses using MEGA 6 software (www.megasoftware.net/). The phylogenetic trees were generated using the neighbour-joining (N-J) tree method, and the reliability of the internal branches was assessed by 1000 bootstrap replications. The reference sequences of MDV genes were retrieved from the GenBank database, and their accession numbers are listed in supplementary Table 1.
Results
Gross pathological findings
Grossly, the peripheral nerves of many cases showed no macroscopic changes. However, some chickens showed swelling, oedema, loss of striation, and discolouration of their peripheral nerves.
The gross picture of the visceral organs showed severe enlargement in one or all of the following organs: the liver, spleen, proventriculus, intestine, and kidneys. The lesion distribution ranged from diffuse to multifocal, with white raised foci on the surface. Nodules were deeply seated within the parenchyma of the organs’ cut-sections. The size of the nodules ranged from pin-point foci to large nodules of 1–2 cm in diameter ((a)). Some cases showed only diffuse enlargement without apparent nodular focal lesions.
Figure 1. (a) Numerous white neoplastic nodules of variable size throughout the enlarged liver lobes. (b) Peripheral nerve showing mild lymphoblastic cellular infiltration in between the nerve axons (type C MDV nerve lesions). H&E, bar = 50 μm. (c) Liver showing diffuse heavy infiltration of pleomorphic lymphoblastic cells with highly mitotic activities (arrowhead) with scarcely distributed remnants of degenerated hepatocytes (arrows). H&E, bar = 20 μm. (d) Proventriculus of MDV-infected chicken showing mitotic division of pleomorphic lymphoblastic cells (arrowhead), H&E, bar = 20 μm. (e) Kidney showing marked lymphoblastic cellular infiltration (arrowheads) among remnants of degenerated and necrosed renal tubules (arrows). H&E, bar = 50 μm. (f) Hepatocytic lymphoma of MDV-infected chicken immunolabelled with CD3 antibody, counterstained with Mayer’s haematoxylin, immunohistochemistry, bar = 20 μm.
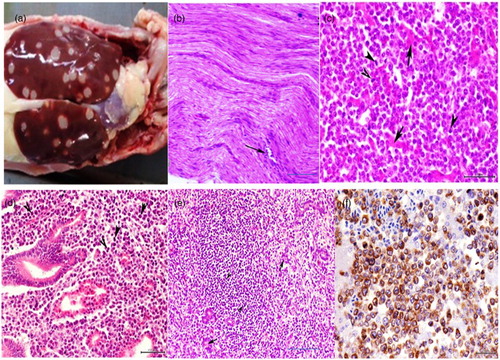
Histopathological findings
Histopathological examination of the peripheral nerves revealed a predominance of type C nerve lesions represented by very scarce small lymphocytic infiltrations ((b)) of nerve axons and the perineuronal nerve sheath. However, histopathological examination of the visceral organs revealed clear lymphoproliferative changes in the liver, spleen, kidney, lung, proventriculus, and intestine, which were more severe in intensity and distributions. The parenchyma of some of the severely affected organs was completely replaced by lymphoid elements.
In the liver, focal and diffuse pleomorphic cell infiltration was clearly detected. These cells are a mixture of lymphoblasts and small- and medium-sized lymphocytes together with a few plasma cells and macrophages. Many mitotic figures were detected in the infiltrating lymphoblasts ((c)). The infiltrating cell accumulations were frequently distributed in the small foci or large nodules around the small-sized blood vessels.
In the spleen, neoplastic cell proliferation was more obvious, and the spleen was completely replaced by tumour cells. Pleomorphic lymphoblastic and lymphocytic cells that distorted the normal texture of the organ were predominant.
In the proventriculus and intestine, marked neoplastic cellular invasion was frequently observed in the mucosa, submucosal layer, and muscularis ((d)). These cells were composed of small, medium, and large lymphoblastic cells, which showed higher mitotic division.
In the kidney, lymphoid cell proliferation was detected in the affected cases. Infiltration of small- and medium-sized lymphocytes as well as large neoplastic lymphoblasts and a few macrophages and plasma cells was detected between the renal tubules. The adjacent renal tubules showed variable necrobiotic changes, and in more severe cases the parenchyma was replaced by connective tissues ((e)).
In the lungs, a large number of pleomorphic lymphoblasts and lymphocytes distorted the normal texture of the organ.
All of the examined cases showed no pathological alterations in the heart and brain tissues.
Immunohistochemistry
Sections from all H&E-examined tissues were stained with CD3 and CD20. The results revealed that in all organs affected, most of the infiltrating or proliferating cells forming the lesion were positive for CD3 ((f)) and negative for CD20. A few scattered large-sized cells were negative for both CD3 and CD20.
Copy numbers of 132-bp repeats in MDV-infected chicken
The copy numbers of 132-bp repeats in the genome of field isolates were examined; the length of one copy of 132-bp repeats is 182-bp long, containing one 132-bp repeat and 50 nucleotides from the primers, the length of two copies of 132-bp repeats are 314 bp, and the length of three copies of 132-bp repeats are 446 bp by analogy. All Japanese MDV field strains examined in this study contained two copies at 314 bp while the (HVT& CVI988/Rispens) vaccines did not amplify the 132-bp repeat (Supplementary Figure 1).
Detection of MDV by PCR
All 30 vaccinated chicken flocks representing the six farms were positive for the meq, pp38, vIL-8, and gL genes by conventional PCR (Supplementary Figure 2). However, the samples that were collected from the age-matched healthy farms were all negative for MDV.
The PCR products of the representative positive samples for MDV meq, vIL-8, gL, and pp38 genes were further sequenced for alignment analysis with the reference strains and for generation of the phylogenetic tree.
The results of PCR using specific primers for REV and ALV were all negative, indicating that neither of these viruses was the cause of the disease condition in all of the examined cases.
Nucleotide and amino acid identities of the meq, pp38, vIL-8, and gL genes between isolated and other reference MDVs submitted in the Genbank
The meq, pp38, vIL-8, and gL gene sequences of the MDV isolates were delineated and submitted to the GenBank database ().
Homology analysis of the meq gene showed that the homologies of the nucleotide and deduced amino acid sequences of the six Gifu isolates were 98–99% and 99.1–100.0%, respectively. Ninety-nine per cent and 98% identity was detected between the MDVs from Gifu and those from other areas of Japan. The nucleotide sequence alignment between the MDVs from Gifu and those from other countries revealed 98–99% homology, while the amino acid sequences showed variable identities: It was only 80% with the vaccinal strains (CVI988. 814, 3004) and Egypt 5 isolate, and 96–97% with the American very virulent plus (vv+); Chinese virulent (v), vv, vv+ strains; and Egyptian isolates.
For the pp38 gene, the homologies of the nucleotide and deduced amino acid sequences of three Gifu isolates were 99% and 99–100.0%, respectively, and were 99% and 99–100%, respectively, between MDVs from Gifu and those from other countries. A homology of 100% was observed with the American and Chinese vv and vv+ strains; however, no sequences of the pp38 gene of MDVs isolated in other areas of Japan are currently available in the GenBank. Therefore, this is the first report of an MDV pp38 gene sequence from Japan.
For the vIL-8 gene, the homologies of the nucleotide and deduced amino acid sequences of the five isolates were 98–100% and 99–100%, respectively, and were 98–99% and 99–100% between MDV isolates from Gifu and those from other countries. However, no sequences of the vIL-8 gene of MDV isolated in other areas of Japan are currently available in the GenBank; thus, this is also the first report of an MDV vIL-8 gene sequence from Japan.
As for the gL gene, the homologies of the nucleotide and deduced amino acid sequences of the five isolates were 99–100%, and was 99–100% and 94–100% between MDV isolates from Gifu and those from other countries, respectively. Homology of 100% was observed with the American vv and vv+ strains, while the vaccinal strains showed a homology of only 94%. However, no sequences of the gL gene of MDV isolated in other areas of Japan are currently available in GenBank; thus, this is the first report of the MDV gL gene sequence from Japan.
Alignment analysis of nucleotide and deduced amino acid sequences of meq, pp38, vIL-8, and gL genes
Alignment analysis of the nucleotide and its deduced amino acid sequences of the Gifu isolates and the published MDVs were performed. The GA strain was considered as the reference strain, and nucleotide mutations, insertions, and/or deletions were observed. Nucleotide mutations at several positions were observed in the meq and vIL-8, and at two positions in the pp38 gene. Some of these mutations were transcribed, resulting in subsequent mutation of the deduced amino acids, whereas others were silent nucleotide mutations. The amino acid mutations in the meq gene of MDVs displayed similarity at several positions, including positions 71, 77, 80, 88, 93, 115, 119, 139, 153, 176, 195, 218, 264, 281, 294, 297, 300, and 301. The amino acid mutations at positions 115, 176, 195, 218, 281, 294, 297, 300, and 301 were detected in the field MDVs from Japan. However, the amino acid mutations at positions 176, 218, 281, 294, 297, 300, and 301 were unique in the present Gifu isolates. Two of four mutations (176 and 218) unique for vv+ strains (positions 119, 153, 176, and 218) were also present in the Gifu isolate (S3 A, B Figure).
As for the pp38 gene, the complete nucleotide and deduced amino acid sequences of three isolates were compared with those of the other 27 reference MDVs, and the results showed regularity in mutations at positions 81 (C→G), 320 (A→G), and 326 (A→G), resulting in amino acid mutations at position 107 (glutamine→arginine) in the vaccinal and mild strains (CVI988 and TQ12), and at position 109 (glutamate→glycine) in virulent MDVs from the USA (S4 A, B Figure).
The nucleotide and deduced amino acid sequences of the vIL-8 gene in six isolates were compared with those of the other 25 reference MDVs, and the results showed regularity in the mutations at positions 11 (T→C), 13 (T→C), 59 (G→C), 164 (G→C), 197 (G→A), 228 (T→C), 267 (A→G), 295 (T→G), and 612 (G→A), resulting in amino acid mutations at positions 4 (leucine→serine), 20 (glycine→alanine), 55 (cystine→serine), and 66 (glycine→glutamine). The results also revealed deletion of two nucleotides at positions 640 and 641, and positions 674 and 675 in all strains in comparison to the GA strain, resulting in mutation from aspartic acid→alanine, and from valine→unidentified amino acid at positions 213, 214, and 225. The mutations at positions 20 (glycine→alanine), 135 (glutamic acid), and 240 (leucine) are here reported for the first time in Gifu isolates. An insertion at position 516–617 of the nucleotide sequences resulted in an amino acid mutation at position 240 (leucine) (Supplementary Figure 5(A, B)).
For gL, no consistent or regular mutations were detected in either the Gifu isolates or in the reference strains.
Phylogenetic analysis based on the nucleotide and deduced amino acid sequences of meq, pp38, and vIL-8 genes
Phylogenetic analysis based on the meq sequences of six isolates and the reference strains showed that the analysed MDVs strains could be separated into two clusters (cluster 1 and cluster 2). The six MDVs isolated from Gifu and other virulent MDVs from Japan, Egypt, and China were included in cluster 1, while the vaccine strains, mild virulent strains, virulent, and very virulent strains from the USA, and other virulent strains from Japan were included in cluster 2. In addition, the phylogenetic tree of the meq gene showed that the MDVs in cluster 2 could be further grouped into two branches: a virulent strain from the USA, three Japanese isolates, and one Egyptian isolate were included in one branch, while the vaccine strains or mild virulent strains and two other Japanese isolates were included in another branch ().
Figure 2. Phylogenetic analysis based on nucleotide sequences of the meq gene of Gifu isolates and other reference MDVs. The phylogenetic tree was constructed using MEGA version 6.0 by the N-J method with 1000 bootstrap replicates.
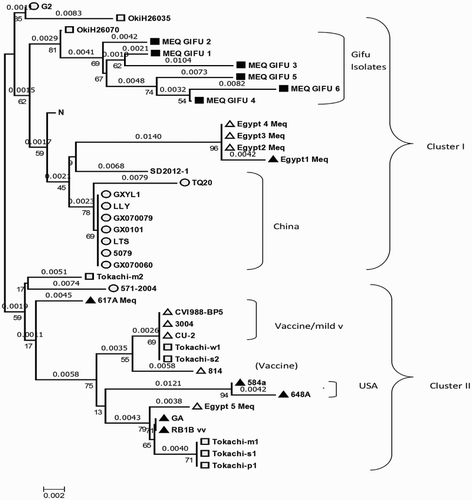
The phylogenetic tree of the pp38 gene showed clustering of the three Gifu isolates with v, vv, and vv+ strains from the USA, China, and India together with a mild and one vaccinal strain in one cluster. The other cluster included two vaccinal strains, mild virulent and virulent strains ((a)).
Figure 3. (a) Phylogenetic analysis based on nucleotide sequences of the pp38 (a) and vIL-8 (b) gene of Gifu isolates and other reference MDVs. (b)The phylogenetic tree was constructed using MEGA version 6.0 by the N-J method with 1000 bootstrap replicates.
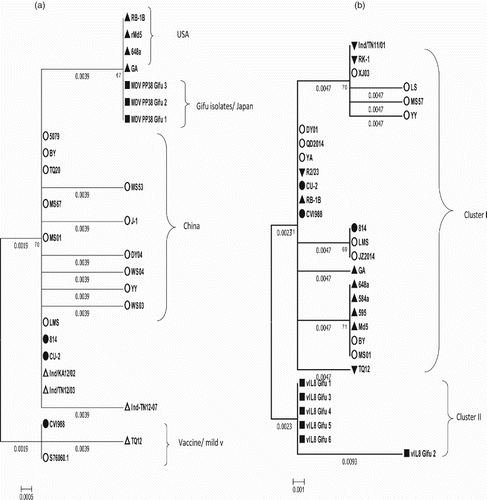
The phylogenetic tree of the vIL8 gene showed that the six MDVs isolated from Gifu were included in one cluster, while the rest of the reference strains, including v, vv, and vv+ strains from the USA and China together with the mild virulent and vaccinal strains, were included in the other cluster ((b)).
No obvious clustering or branching was observed in the phylogenetic tree of the gL gene.
Discussion
MD, caused by MDV, is a highly contagious neoplastic disease of chicken that can be prevented by vaccination. However, in recent years, many cases of vaccine failure have been reported worldwide as chickens develop signs of MD in spite of proper vaccination (Witter, Citation1997; Kamaldeep et al., Citation2007; Gong et al., Citation2013; Hassanin et al., Citation2013; Abd-Ellatieff et al., Citation2017). Distinct polymorphism and point mutations in the meq gene of MDV have been reported to be associated with virulence and oncogenicity (Tian et al., Citation2011). The present study was carried out with the objective to update information on the pathological alterations of the disease and characterize the field isolates of MDV on the basis of sequencing of the four MDV-unique genes meq, pp38, gL, and vIL-8.
Samples from a total of 190 suspected cases of MD were collected and processed for pathological and molecular diagnosis. Detection of the presence of MDV in these samples was confirmed by PCR. A polymorphism study was carried out by sequencing the nearly complete lengths of the meq, pp38, vIL-8, and gL genes to analyse the diversity in all MDV isolates.
The nerve histopathological lesions were mild despite the presence of prominent lymphoma distributed in the different visceral organs. In our opinion, this apparent contradiction could be explained on the basis of the difference in the virulence of field strains and the use of different vaccinal strains over the past 40 years makes the pathological picture become non-pathognomonic (Abd-Ellatieff et al., Citation2017). Pathological forms of the disease that were classified previously according to nerve histopathological changes in relation to visceral lesions become more mixed and are not easily diagnosed histopathologically (Payne & Biggs, Citation1967; Fujimoto et al., Citation1971; Kamaldeep et al., Citation2007).
Transactivation of the meq gene most likely leads to cell transformation and lymphoma formation (Levy et al., Citation2003; Ajithdoss et al., Citation2009). The length of the meq gene from mild Marek's disease virus (mMDV) strains such as CU-2, and vaccinal strains (CVI988, 814, and 3004) was 177 bp longer than those of virulent MDV strains (Lee et al., Citation2000; Chang et al., Citation2002), which result in a 59 amino acid insertion. Insertion of these 59 amino acids has a suppressive effect on Meq protein expression (Chang et al., Citation2002); therefore, detection of a short meq sequence in the present study further confirmed that virulent field MDVs are the cause of the present condition.
Sequence analysis revealed several point mutations. Mutations at positions 80, 115, 139, and 176 of Meq amino acid sequences are considered to be virulent genetic characteristics of the MDVs in China (Tian et al., Citation2011). Amino acid mutations at the second proline repeats (positions 176 and 218) were present in Gifu isolates, which together with mutations at positions 119, 153, 176, and 218 are considered unique for vv+ strains. Moreover, it was reported that MDV strains of increased virulence (vv and vv+ MDVs) contain interruptions in the direct repeats of prolines at the second position, with the most virulent MDVs having the greatest number of interruptions (P→Q, P→A, and P→A at positions 153, 176, and 276, respectively) (Shamblin et al., Citation2004).
Distinct polymorphisms and point mutations have been identified in the gene encoding C-terminal sequences of the Meq protein (Shamblin et al., Citation2004). Likewise, several point mutations have been shown in 13 MDV Polish strains, which were correlated with the MDV virulence (Wozniakowski et al., Citation2010).
pp38 is required to establish cytolytic infection in B lymphocytes, to produce an adequate level of latently infected T cells and to maintain the transformed status of lymphocytes by preventing apoptosis. Pp38 also plays a role in viral reactivation in the latent period and in cell transformation (Gimeno et al., Citation2005). Detection of pp38 can differentiate between virulent MDVs (MDV-1 strains) and vaccinal strains as CVI988 vaccination will not lead to false-positive results when MDV tumour tissues are collected from chickens 7 weeks of age or older (Cao et al., Citation2013) as the level of the CVI988 vaccine virus is so low at 7 weeks of age that pp38 from CVI988 is unlikely to be detected at this age, and therefore does not cause false-positive results.
In the present report, analysis of the nucleotides and its deduced amino acid sequences of pp38 revealed an amino acid mutation at position 109 (glycine→glutamic acid), which is equivalent to the mutation at position 326 (G→A), which is considered a feature of virulent MDVs isolated from the USA (Shamblin et al., Citation2004). The results also showed a similarity between the present isolates and the virulent reference strain used in this study at position 320 (A) in comparison to (G) in the vaccinal strains (CVI988). It was also reported that a single nucleotide polymorphism (SNP) at base 320 is consistent between CVI988 (which has “G” at this position) and all virulent strains (which have “A”) (Cui et al., Citation1991; Spatz et al., Citation2007). Very recently, the single nucleotide polymorphism at position 320 was reported to be a reliable biomarker for differentiating and quantifying CVI988 and virulent strains (Baigent et al., Citation2016).
After alignment of the amino acid sequence of the pp38 gene of all MDVs, the mutation at position 107 was detected in mild and vaccinal strains (TQ12, CVI988) only, and glycine was replaced by glutamate at position 109 in the isolates from China; v, vv, and vv+ MDVs (GA, RB1B, 584A, and 648A) from the USA; and the isolates from Gifu. Therefore, the mutation (glycine →glutamate) at position 109 can be considered as a feature of virulence.
The vIL-8 gene is encoded within the genome of MDV and shows closest homology to mammalian and avian IL-8 molecules. It is expressed during cytolytic infection. The function of vIL-8 is the attraction of T cells, especially after vIL-8 receptors are up-regulated by interferon-γ. vIL-8 might be important for the switch of infection from B to T lymphocytes (Schat & Xing, Citation2000). This gene was shown to be highly conserved in v, vv, and vv+ strains (648A, RB1B, MD11, and GA strains) (Zhang et al., Citation2002). A comparison of amino acid sequences revealed several point mutations at positions 20 (glycine→alanine), 135 (*→glutamic acid), and 240 (insertion of leucine) in the present Gifu isolates; at positions 4 (leucine→serine) and 99 (glycine→glutamic acid) in Chinese strains; and at position 66 (cysteine→serine) in vv and vv+ strains (648a, 584a, 595, and Md5). Thus, changes in virulence as a result of mutation in this gene are strongly expected.
A phylogenetic analysis based on the meq gene revealed that these isolates were found in the same cluster with recent Japanese, Chinese, and Egyptian isolates, while mild and vaccine strains formed a separate cluster. The phylogenetic analysis based on the pp38 gene revealed the close relatedness to the vv and vv+ American and Chinese isolates, while mMDV and vaccine strains formed a separate cluster. Moreover, phylogenetic analysis based on the vIL-8 gene revealed that these isolates formed a separate cluster showing the close relatedness to the vv and vv+ American and Chinese isolates.
In conclusion, the nucleotide and amino acid changes in the main oncoproteins Meq, pp38, and vIL-8 could be a criterion in the differentiation and determination of the pathogenicity and oncogenicity of MDV strains. Similar conclusions have been reached for MDV-serotype 1-unique genes such as meq (Jones et al., Citation1992), pp38 (Cui et al., Citation1991), and vIL-8 (Parcells et al., Citation2001), which were reported to be associated with viral oncogenicity and pathogenicity.
The emergence of more virulent MDV strains is a significant problem for the poultry industry, and thus more studies are required to update all of the causes of the increased pathogenicity and virulence of this oncogenic virus. In addition, a challenge study using the field isolates and a reference vv MDV strain is required to confirm these findings. Investigations aiming to develop a new more effective vaccine are required to overcome this devastating avian oncogenic viral disease threatening the poultry industry.
Supplemental Table
Download MS Word (92 KB)Supplemental Figure
Download MS Word (10.1 MB)Disclosure statement
No potential conflict of interest was reported by the authors.
References
- Abd-Ellatieff, H.A., Abou-Rawash, A.-R.A., Ellakany, H.F. & Yanai, T. (2017). Th1 and Th2 cytokines activity during transformation and lymphoma formation stage in chicken naturally infected with Marek’s disease virus. IOSR Journal of Agriculture and Veterinary Science, 10, 72–80. doi: 10.9790/2380-1001017280
- Ajithdoss, D.K., Reddy, S.M., Suchodolski, P.F., Lee, L.F., Kung, H.-J. & Lupiani, B. (2009). In vitro characterization of the Meq proteins of Marek’s disease virus vaccine strain CVI988. Virus Research, 142, 57–67. doi: 10.1016/j.virusres.2009.01.008
- Awad, A.M., Abd El-Hamid, H.S., Abou Rawash, A.A. & Ibrahim, H.H. (2010). Detection of reticuloendotheliosis virus as a contaminant of fowl pox vaccines. Poultry Science, 89, 2389–2395. doi: 10.3382/ps.2010-00899
- Awad, A.M., Ellakkany, H.F., Eid, A.E. & Abd El-Hamid, H.S. (2004, September 25–27). Case report: avian leukosis virus subgroup-J (ALV-J) infection in broiler breeder chickens. Proceedings of the 6th Scientific Conference of the Egyptian branch of the World Veterinary Poultry Association.
- Baigent, S.J., Nair, V.K. & Le Galludec, H. (2016). Real-time PCR for differential quantification of CVI988 vaccine virus and virulent strains of Marek’s disease virus. Journal of Virological Methods, 233, 23–36. doi: 10.1016/j.jviromet.2016.03.002
- Buckmaster, A.E., Scott, S.D., Sanderson, M.J., Boursnell, M.E., Ross, N.L. & Binns, M.M. (1988). Gene sequence and mapping data from Marek’s disease virus and herpesvirus of turkeys: implications for herpesvirus classification. Journal of General Virology, 69, 2033–2042. doi: 10.1099/0022-1317-69-8-2033
- Cao, W., Mays, J., Dunn, J., Fulton, R., Silva, R. & Fadly, A. (2013). Use of polymerase chain reaction in detection of Marek’s disease and reticuloendotheliosis viruses in formalin-fixed, paraffin-embedded tumorous tissues. Avian Diseases, 57, 785–789. doi: 10.1637/10542-032713-ResNote.1
- Chang, K.-S., Lee, S.-I., Ohashi, K., Ibrahim, A. & Onuma, M. (2002). The detection of the meq gene in chicken infected with Marek’s disease virus serotype 1. Journal of Veterinary Medical Science, 64, 413–417. doi: 10.1292/jvms.64.413
- Cui, X., Lee, L.F., Reed, W.M., Kung, H.-J. & Reddy, S.M. (2004). Marek’s disease virus-encoded vIL-8 gene is involved in early cytolytic infection but dispensable for establishment of latency. Journal of Virology, 78, 4753–4760. doi: 10.1128/JVI.78.9.4753-4760.2004
- Cui, Z., Lee, L.F., Liu, J.L. & Kung, H.-J. (1991). Structural analysis and transcriptional mapping of the Marek’s disease virus gene encoding pp38, an antigen associated with transformed cells. Journal of Virology, 65, 6509–6515.
- De Boer, G., Groenendal, J., Boerrigter, H., Kok, G. & Pol, J. (1986). Protective efficacy of Marek’s disease virus (MDV) CVI-988 CEF65 clone C against challenge infection with three very virulent MDV strains. Avian Diseases, 30, 276–283. doi: 10.2307/1590529
- Ding, J., Cui, Z. & Lee, L.F. (2007). Marek’s disease virus unique genes pp38 and pp24 are essential for transactivating the bi-directional promoters for the 1.8 kb mRNA transcripts. Virus Genes, 35, 643–650. doi: 10.1007/s11262-007-0129-5
- Fujimoto, Y., Nakagawa, M., Okada, K., Okada, M. & Matsukawa, K. (1971). Pathological studies of Marek’s disease: I the histopathology on field cases in Japan. Japanese Journal of Veterinary Research, 19, 7–26.
- Gimeno, I., Witter, R., Hunt, H., Reddy, S., Lee, L. & Silva, R. (2005). The pp38 gene of Marek’s disease virus (MDV) is necessary for cytolytic infection of B cells and maintenance of the transformed state but not for cytolytic infection of the feather follicle epithelium and horizontal spread of MDV. Journal of Virology, 79, 4545–4549. doi: 10.1128/JVI.79.7.4545-4549.2005
- Gong, Z., Zhang, L., Wang, J., Chen, L., Shan, H., Wang, Z. & Ma, H. (2013). Isolation and analysis of a very virulent Marek’s disease virus strain in China. Virology Journal, 10, 155. doi: 10.1186/1743-422X-10-155
- Hassanin, O., Abdallah, F. & El-Araby, I.E. (2013). Molecular characterization and phylogenetic analysis of Marek’s disease virus from clinical cases of Marek’s disease in Egypt. Avian Diseases, 57, 555–561. doi: 10.1637/10337-082912-Reg.1
- Jarosinski, K.W., Tischer, B.K., Trapp, S. & Osterrieder, N. (2006). Marek’s disease virus: lytic replication, oncogenesis and control. Expert Review of Vaccines, 5, 761–772. doi: 10.1586/14760584.5.6.761
- Jones, D., Lee, L., Liu, J.-L., Kung, H.-J. & Tillotson, J.K. (1992). Marek disease virus encodes a basic-leucine zipper gene resembling the fos/jun oncogenes that is highly expressed in lymphoblastoid tumors. Proceedings of the National Academy of Sciences, 89, 4042–4046. doi: 10.1073/pnas.89.9.4042
- Kamaldeep, P., Sharma, C. & Narang, G. (2007). Occurrence of Marek’s disease in vaccinated poultry flocks of Haryana (India). International Journal of Poultry Science, 6, 372–377. doi: 10.3923/ijps.2007.372.377
- Lee, L.F., Wu, P., Sui, D., Ren, D., Kamil, J., Kung, H.J. & Witter, R.L. (2000). The complete unique long sequence and the overall genomic organization of the GA strain of Marek’s disease virus. Proceedings of the National Academy of Sciences, 97, 6091–6096. doi: 10.1073/pnas.97.11.6091
- Levy, A.M., Izumiya, Y., Brunovskis, P., Xia, L., Parcells, M.S., Reddy, S.M., Lee, L., Chen, H.-W. & Kung, H.-J. (2003). Characterization of the chromosomal binding sites and dimerization partners of the viral oncoprotein Meq in Marek’s disease virus-transformed T cells. Journal of Virology, 77, 12841–12851. doi: 10.1128/JVI.77.23.12841-12851.2003
- Lupiani, B., Lee, L.F., Cui, X., Gimeno, I., Anderson, A., Morgan, R.W., Silva, R.F., Witter, R.L., Kung, H.-J. & Reddy, S.M. (2004). Marek’s disease virus-encoded meq gene is involved in transformation of lymphocytes but is dispensable for replication. Proceedings of the National Academy of Sciences of the United States of America, 101, 11815–11820. doi: 10.1073/pnas.0404508101
- Murata, S., Hashiguchi, T., Hayashi, Y., Yamamoto, Y., Matsuyama-Kato, A., Takasaki, S., Isezaki, M., Onuma, M., Konnai, S. & Ohashi, K. (2013). Characterization of Meq proteins from field isolates of Marek’s disease virus in Japan. Infection, Genetics and Evolution, 16, 137–143. doi: 10.1016/j.meegid.2012.12.032
- Okazaki, W., Purchase, H. & Burmester, B. (1970). Protection against Marek’s disease by vaccination with a herpesvirus of turkeys. Avian Diseases, 14, 413–429. doi: 10.2307/1588488
- Parcells, M.S., Lin, S.-F., Dienglewicz, R.L., Majerciak, V., Robinson, D.R., Chen, H.-C., Wu, Z., Dubyak, G.R., Brunovskis, P., Hunt, H.D., Lee, L.F. & Kung, H.-J. (2001). Marek’s disease virus (MDV) encodes an interleukin-8 homolog (vIL-8): characterization of the vIL-8 protein and a vIL-8 deletion mutant MDV. Journal of Virology, 75, 5159–5173. doi: 10.1128/JVI.75.11.5159-5173.2001
- Payne, L. & Biggs, P. (1967). Studies on Marek’s disease. II. Pathogenesis. Journal of the National Cancer Institute, 39, 281–302.
- Payne, L. & Rennie, M. (1976). B and T Lymphocyte Responses in Marek’s Disease. In J. Clemmesen, & D.S. Yohn (Eds). Comparative Leukemia Research 1975 Vol. 43 (pp. 351–353). Tokyo/Basel: University of Tokyo Press/Karger Publishers.
- Qin, L., Gao, Y., Ni, W., Sun, M., Wang, Y., Yin, C., Qi, X., Gao, H. & Wang, X. (2013). Development and application of real-time PCR for detection of subgroup J avian leukosis virus. Journal of Clinical Microbiology, 51, 149–154. doi: 10.1128/JCM.02030-12
- Raja, A., Dhinakar, R.G., Bhuvaneswari, P., Balachandran, C. & Kumanan, K. (2008). Detection of virulent Marek’s disease virus in poultry in India. Acta Virologica, 53, 255–260. doi: 10.4149/av_2009_04_255
- Rajcani, J. & Vojvodova, A. (1998). The role of herpes simplex virus glycoproteins in the virus replication cycle. Acta Virologica, 42, 103–118.
- Rispens, B.H., van Vloten, H., Mastenbroek, N., Maas, H.J. & Schat, K.A. (1972). Control of Marek’s disease in the Netherlands. I. Isolation of an avirulent Marek’s disease virus (strain CVI 988) and its use in laboratory vaccination trials. Avian Diseases, 16, 108–125. doi: 10.2307/1588905
- Sabattini, E., Bisgaard, K., Ascani, S., Poggi, S., Piccioli, M., Ceccarelli, C., Ceccarelli, C., Pieri, F., Fraternali-Orcioni, G. & Pileri, S.A. (1998). The EnVision++ system: a new immunohistochemical method for diagnostics and research. Critical comparison with the APAAP, ChemMate, CSA, LABC, and SABC techniques. Journal of Clinical Pathology, 51, 506–511. doi: 10.1136/jcp.51.7.506
- Schat, K.A. & Xing, Z. (2000). Specific and nonspecific immune responses to Marek’s disease virus. Developmental & Comparative Immunology, 24, 201–221. doi: 10.1016/S0145-305X(99)00073-7
- Shamblin, C.E., Greene, N., Arumugaswami, V., Dienglewicz, R.L. & Parcells, M.S. (2004). Comparative analysis of Marek’s disease virus (MDV) glycoprotein-, lytic antigen pp38-and transformation antigen meq-encoding genes: association of meq mutations with MDVs of high virulence. Veterinary Microbiology, 102, 147–167. doi: 10.1016/j.vetmic.2004.06.007
- Spatz, S.J., Zhao, Y., Petherbridge, L., Smith, L.P., Baigent, S.J. & Nair, V. (2007). Comparative sequence analysis of a highly oncogenic but horizontal spread-defective clone of Marek’s disease virus. Virus Genes, 35, 753–766. doi: 10.1007/s11262-007-0157-1
- Tian, M., Zhao, Y., Lin, Y., Zou, N., Liu, C., Liu, P., Cao, S., Wen, X. & Huang, Y. (2011). Comparative analysis of oncogenic genes revealed unique evolutionary features of field Marek’s disease virus prevalent in recent years in China. Virology Journal, 8, 121. doi: 10.1186/1743-422X-8-121
- Witter, R. (1972). Turkey herpesvirus: lack of oncogenicity for turkeys. Avian Diseases, 16, 666–670. doi: 10.2307/1588684
- Witter, R. (1983). Characteristics of Marek’s disease viruses isolated from vaccinated commercial chicken flocks: association of viral pathotype with lymphoma frequency. Avian Diseases, 27, 113–132. doi: 10.2307/1590377
- Witter, R. (1997). Increased virulence of Marek’s disease virus field isolates. Avian Diseases, 41, 149–163. doi: 10.2307/1592455
- Witter, R. & Lee, L. (1984). Polyvalent Marek’s disease vaccines: safety, efficacy and protective synergism in chickens with maternal antibodies 1. Avian Pathology, 13, 75–92. doi: 10.1080/03079458408418510
- Woźniakowski, G., Mamczur, A. & Samorek-Salamonowicz, E. (2014). Molecular evolution of Marek’s disease virus (MDV) field strains in a 40-year time period. Avian Diseases, 58, 550–557. doi: 10.1637/10812-030614-Reg.1
- Woźniakowski, G., Mamczur, A. & Samorek-Salamonowicz, E. (2015). Common occurrence of Gallid herpesvirus-2 with reticuloendotheliosis virus in chickens caused by possible contamination of vaccine stocks. Journal of Applied Microbiology, 118, 803–808. doi: 10.1111/jam.12734
- Wozniakowski, G., Samorek-Salamonowicz, E. & Kozdrun, W. (2010). Sequence analysis of meq oncogene among Polish strains of Marek’s disease. Polish Journal of Veterinary Sciences, 13, 263.
- Zhang, X., Qin, A., Jin, W. & Liu, Y. (2002). Expression of viral interleukin-8 gene of Marek’s disease virus in Escherichia coli. Chinese Journal of Veterinary Science, 23, 521–524.