ABSTRACT
Sequence variability in the S1 gene determines the genotype of infectious bronchitis virus (IBV) strains. A single RT-PCR assay was developed to amplify and sequence the full S1 gene for six classical and variant IBVs (M41, D274, 793B, IS/885/00, IS/1494/06 and Q1) enriched in allantoic fluid (AF) or the same AF inoculated onto Flinders Technology Association (FTA) cards. Representative strains from each genotype were grown in specific-pathogen-free eggs and RNA was extracted from AF. Full S1 gene amplification was achieved using primer A and primer 22.51. Products were sequenced using primers A, 1050+, 1380+ and SX3+ to obtain short sequences covering the full gene. Following serial dilutions of AF, detection limits of the partial assay were higher than those of the full S1 gene. Partial S1 sequences exhibited higher-than-average nucleotide similarity percentages (79%; 352 bp) compared to full S1 sequences (77%; 1756 bp), suggesting that full S1 analysis allows greater strain differentiation. For IBV detection from AF-inoculated FTA cards, four serotypes were incubated for up to 21 days at three temperatures, 4°C, room temperature (approximately 24°C) and 40°C. RNA was extracted and tested with partial and full S1 protocols. Through partial sequencing, all IBVs were successfully detected at all sampling points and storage temperatures. In contrast, using full S1 sequencing it was not possible to amplify the gene beyond 14 days or when stored at 40°C. Data presented show that for full S1 sequencing, a substantial amount of RNA is needed. Field samples collected onto FTA cards are unlikely to yield such quantity or quality.
Abbreviations: AF: allantoic fluid; CD50: ciliostatic dose 50; FTA: Flinders Technology Association; IB: infectious bronchitis; IBV: infectious bronchitis virus
Introduction
Infectious bronchitis (IB) is an acute viral disease caused by infectious bronchitis viruses (IBVs) that mainly infect the respiratory system in broiler and layer chicken flocks (Cavanagh, Citation2005). Infection causes economic losses due to high mortality, poor body weight gain (Ignjatovic & Sapats, Citation2000), decreased egg production (reaching up to 70%) and reduced egg quality (increased eggshell pigment) in layers (Muneer et al., Citation2000). Further complications can occur in some cases, including cystic oviducts (Benyeda et al., Citation2009) and false layer syndrome (Crinion & Hofstad, Citation1972). Certain IBVs, including several Australian strains, have been associated with renal complications such as nephritis (Mahmood et al., Citation2011) and digestive complications, such as proventriculitis (Toffan et al., Citation2013).
IBV is a single-stranded RNA virus (approximately 27.6 kb in size) belonging to the family Coronaviridae. It contains four structural proteins: spike protein (S), small membrane envelope protein (E), membrane protein (M) and nucleoprotein (N). The spike protein comprises 1145 amino acids and undergoes post-translational cleavage to form S1 and S2 subunits (Jackwood & de Wit, Citation2013). The functions of spike include attachment to host cells, neutralization of antibodies and initiation of protective immunity (Johnson et al., Citation2003). Furthermore, it is the most variable of the IBV proteins, with most mutations and recombination events mapping to the S1 region (Kingham et al., Citation2000), which can lead to the emergence of new variant strains (Zhang et al., Citation2015).
The relationship between antigenic differences among IBV strains has been investigated (Jackwood & de Wit, Citation2013), and previous work has shown that partial S1 sequences have a stronger correlation with protective relatedness between strains, rather than antigenic relatedness (Ladman et al., Citation2006). In addition, variation in the nucleotide sequence of S1 may lead to alterations in the virus categorization, such as receptor-binding abilities (Jackwood & de Wit, Citation2013).
More than 25 serotypes have been recognized to date, often differing by up to 50% at the amino acid level for the S1 gene (Gelb et al., Citation2005). Only a minor amino acid sequence alteration, at the very least a 5% change (25–30 amino acids) in the S1 subunit, is required to affect the protection status conferred by vaccinations of the same serotype (Cavanagh, Citation2003). As a result, low cross-protection is provided by different vaccine virus serotypes (Kuo et al., Citation2010).
In recent years, sequence analysis has become a common method to identify strain types, typically being carried out on specific nucleotide regions of the S gene (Cavanagh, Citation2003) or the N gene (Williams et al., Citation1992). Previous studies have focused on analysing different nucleotide regions of the S1 gene (between 228 and 380bp) (Wang & Tsai, Citation1996; Al-Shekaili et al., Citation2015), leading to difficulties when comparing genotype analysis between studies.
For many years, the Massachusetts (Mass) serotype, originally isolated in Massachusetts in the 1930s, was the only recognized serotype (via virus neutralization). Jungherr et al. (Citation1956) reported a Connecticut IBV type, which was not cross-protected or cross-neutralized against the Mass IBV strain (Jungherr et al., Citation1956). During the 1960s, the Australian nephropathogenic strains were first identified and connected to uraemia (Cumming, Citation1963), including Australia/N1/62 which is considered to be the first strain isolated in the continent (Jackwood, Citation2012). In 1984, researchers in the Doorn Institute, The Netherlands, first isolated the Dutch strain D207 from Mass-vaccinated flocks (Davelaar et al., Citation1984). The 793B serotype was identified in the 1990s (Parsons et al., Citation1992), with sequence data distinct from Dutch and Mass strains (Cavanagh, Citation2005). The QX genotype was first described in 1996 in China, and was associated with severe nephritis, proventriculitis, tracheitis, decreased egg production and false layer syndrome (De Wit et al., Citation2011). Later, another variant, known as Q1, which can also cause proventriculitis (Yu et al., Citation2001), was detected from layer flocks showing respiratory signs. While certain genotypes like 793B and Mass have global distribution (Cook et al., Citation1996), others are considered to have regional importance, such as IS/885/00 (Meir et al., Citation2004) and IS/1494/06 (GenBank accession number EU780077).
Recently, there has been growing interest in complete S1 sequencing, with reports from Thailand, Sweden and India (among others) aiming to further understand the molecular characterization of IBV (Pohuang et al., Citation2011; Abro et al., Citation2012; Kamble et al., Citation2016). In addition to the classical IBVs, recombinant viruses have been identified in the field. Sequencing the full S1 gene, rather than a partial segment, will aid in the identification of such variants. In addition, it was suggested during the 8th International Symposium on avian Corona–Pneumoviruses and complicating pathogens (2014) that the full S1 gene should be sequenced to determine the genotype of IBV isolates. Work in Italy has outlined the benefits of sequencing the full S1 gene and classification of IBV strains into a number of distinct lineages (Valastro et al., Citation2016). An earlier study aimed to obtain full S1 sequences by using two primers to amplify and sequence around 1700 bp from the S1 gene, after purifying and digesting with restriction enzymes (Kwon et al., Citation1993). The same primers were used in another study (Abdel-Moneim et al., Citation2006), while a separate investigation looked into the differences in S1 and partial N gene sequences (Huang et al., Citation2004).
Flinders Technology Association (FTA) cards have been utilized for a number of IBV studies looking to detect the virus from clinical or laboratory samples (Moscoso et al., Citation2005; Ganapathy et al., Citation2015). While the cards are useful for transporting genomic material worldwide, the quality and quantity of RNA eluted vary depending on the initial amount of IBV RNA, storage duration and temperature (Moscoso et al., Citation2005), and the extraction methodology. Previous work has outlined that for IBV, a partial S1 amplification and sequencing from FTA card extraction is successful to a viral concentration of 100.2 EID50/ml (Awad et al., Citation2012). For IBV, it has been shown that RNA can be successfully extracted from field samples embedded onto FTA cards, and following RT-PCR and sequencing, genotypes can be determined based on the partial S1 gene (Ganapathy et al., Citation2015; Ball et al., Citation2016). Moscoso et al. (Citation2005) and Awad et al. (Citation2014) also demonstrated the effects of different temperature storage of IBV-inoculated FTA cards (Moscoso et al., Citation2005; Awad et al., Citation2014). However, to date, the feasibility of full S1 gene sequencing from FTA cards has not been examined.
The aim of this study was to develop and evaluate a single RT-PCR assay in order to amplify and sequence the full S1 gene of six common IBV genotypes grown in allantoic fluid. The sensitivity of the scheme was investigated using RNA extracted from allantoic fluid, both directly and after inoculation onto FTA cards, and field samples submitted on FTA cards. The influence of storage temperatures on detectability of these IBV strains, through full and partial S1 PCR and sequencing, was also assessed.
Materials and methods
Virus strains
The following virulent IBV strains were used in this study: 793B (106.4 CD50/ml), M41 (106.3 CD50/ml), D274 (106.75 CD50/ml), QX (106.3 CD50/ml), Q1 (105.5 CD50/ml), IS/1494/06 (106.3 CD50/ml) and IS/885/00 (106.3 CD50/ml) (Ganapathy et al., Citation2012; Chhabra et al., Citation2015). Viruses were passaged in embryonated specific-pathogen-free chicken eggs and titrated in tracheal organ cultures to determine the median ciliostatic dose per ml (CD50/ml) (Cook et al., Citation1976).
RNA extraction from AF and FTA cards
Extraction from AF
Viral RNA was extracted using the QIAamp viral RNA Mini Kit (Qiagen, Manchester, UK), according to the manufacturer’s instructions, from 100 µl of neat or diluted allantoic fluid.
Extraction from FTA cards
FTA cards (WhatmanTM, VWR, Leicestershire, UK) were inoculated with 100 µl of ten-fold diluted AF for each virulent strain. The inoculated FTA cards were stored at three different temperatures: 4°C, 40°C and room temperature, defined as 22–26°C. For each sampling point (1, 2, 3, 7, 14, 21 days post-inoculation (dpi), a single, complete FTA circle from each dilution and each temperature was removed using sterile scissors, placed into 1000 µl of TE buffer ((10 mM Tris-HCl (pH 8.0), 1 mM EDTA (pH 8.0) (Thermo Fisher Scientific, Massachusetts, USA)) and incubated for 10–15 min (Sakai et al., Citation2015). After vortexing, 100 µl was taken and processed for RNA extraction using the QIAamp viral RNA Mini Kit (Qiagen) according to the manufacturer's instructions.
IBV RT-PCR and sequencing
Partial S1 RT-PCR
Extracted RNA was used for both partial and full S1 amplifications. For partial S1 amplification, RT-PCR targeting a 393 bp partial sequence of the S1 gene was conducted (Ganapathy et al., Citation2015; Ball et al., Citation2016), followed by gel electrophoresis.
Full S1 RT-PCR
For full S1 amplification, reverse transcription was conducted using the primer 22.51 (5′ GAACGTCTAAAACGACGTGTTCC 3′) (). The reaction mix comprised 5× first strand buffer [250 mM Tris-HCl (pH 8.3), 375 mM KCl, 15 mM MgCl2; Invitrogen], dithiothreitol (100 mM; Invitrogen), dNTPs (dATP, dCTP, dGTP, dTTP) (10 mM), RNasin ribonuclease inhibitor (20 U/µl; Promega), Superscript III Reverse Transcriptase (200 U/µl; Invitrogen), oligonucleotide (10 pmol), sterile distilled water (2.13 µl) and 0.5 µl of template RNA. Reverse transcription conditions were: 48°C for 45 min, and 94°C for 5 min. Generated cDNA was then amplified in a one-step PCR using primer A and primer 22.51 (). The PCR mixture included Supermix [22 mM Tris-HCl (pH 8.4), 55 mM KCl, 1.65 mM MgCl2, 220 µM dNTPs, 22 U/ml recombinant Taq DNA polymerase and stabilizers; Invitrogen], forward and reverse primers (10 pmol) and 0.5 µl of cDNA. Cycling conditions were: 35 cycles of denaturation at 94°C for 60 s, annealing at 54°C for 30 s, extension at 72°C for 60 s and a final extension at 72°C for 10 min. Products were visualized on an agarose gel.
Table 1. Primers used for amplification and sequencing of IBV strains in this study.
Table 2. Partial and full S1 detection limits and corresponding CD50/ml values from diluted AF.
Partial and full S1 sequencing
Positive amplicons (partial or full) were purified (ExoSAP-IT, Affymetrix, Santa Clara, CA, USA) and sent for commercial Sanger sequencing (Source BioScience, Nottingham, UK). For partial sequencing, the positive sense SX3+ primer (Worthington et al., Citation2008) was utilized. For full S1 sequencing, the following primers were used: primer A (Pohuang et al., Citation2011), SX3+ (Worthington et al., Citation2008), 1050+ and 1380+ (Falchieri et al., Citation2013) (). Sequences were then concatenated into a single contig using ChromasPRO v1.7.3. An additional assay was used for the amplification and sequencing of QX for inclusion with analysis (Falchieri et al., Citation2013). In brief, the primer RT QX negative (5′-CATCTTTAACGAACCATCTGG-3′) was used for the RT stage. For amplification, the primers QX S1 start positive (5′-CCAGTTGTGAATTTGAAGAAAGAACAAAAGACCGACTTAG-3′) and QX S1 end negative (5′-CGAACCATCTGGTTCAATACAAAATCTGC-3′) were used in one-step PCR amplification.
Phylogenetic analysis
Reads for the full S1 sequencing were initially examined for their quality and contigs were assembled from each sequencing primer (Primer A, 1050+, 1380+ and SX3+) using ChromasPRO v1.7.3 (http://technelysium.com.au/). Sequences were then trimmed to cover the full S1 gene. Partial S1 sequences [SX3+] were processed using the same methodology. BLAST comparisons against the NCBI database (GenBank) were carried out to confirm strain identity. Alignments for all S1 sequences and reference genomes were carried out in MEGA6 (Tamura et al., Citation2013), with phylogeny comparisons using the maximum likelihood calculation with 1000 bootstrap replicates. Reference strains used were D274 (X15832), 793B (AF093794), IS/885/00 (AY279533), IS/1494/06 (EU780077), Q1 (AF286302), QX (KF297571), M41 (AY561711), H120 vaccine C (KU736750), H120 vaccine D (KU736751), Ma5 vaccine (KU736747), Beaudette strain (DQ001336), Ark-DPI (KX529820), IBV Quebec strain (AF349621), IBV T strain (AY775779) and Connecticut vaccine (EU283058).
Field samples
Twenty-five tissues and swab samples collected from flocks in the Middle East, which were previously confirmed as IBV positive, were used (Ganapathy et al., Citation2015). These samples were imprinted onto FTA cards. RNA was extracted from these samples and subjected to the full S1 RT-PCR described above.
Results
Detection limits for partial versus full S1
Results showed that the minimum detection limits for the full S1 gene from virus-enriched AF were 10−2 for all strains except for M41, which was 10−3 (103.5 CD50/ml). In contrast, the detection limits for the partial S1 were at 10−7 for all strains except for Q1, which was 10−6 (100.5 CD50/ml) (; supplementary Figure 1).
Nucleotide sequence and phylogenetic analysis
The seven partial S1 sequences exhibited a higher average of nucleotide similarity (79%) when compared to the full S1 sequences (77%) with the range being 72–84% for both the partial and full S1 (). This was true for both the classical and variant strains. M41 demonstrated a similarity of 77% (full) and 80% (partial) to D274, whereas the closely related IS/885/00 strain had 82% (full) and 83% (partial) similarity with IS/1494/06. Similarly, the Q1 strain had 77% (full) and 79% (partial) similarity with QX. shows the position of each IBV strain included in this study, alongside other strains obtained from the GenBank.
Figure 1. Phylogenetic tree based on both the partial (grey circle) and full (black triangle) S1 nucleotide sequence among the IBV strains used in this study and reference strains. Analysis inferred using maximum likelihood analysis with Tamura 3-parameter and 1000 bootstrap replicates.
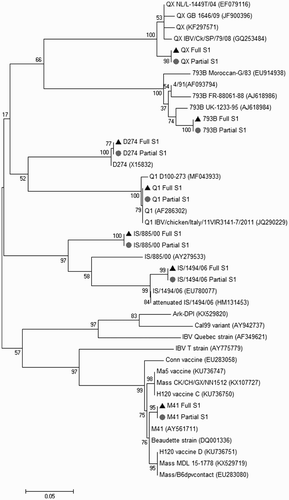
Table 3. Nucleotide identity (%) comparison of the seven IBV strains used in this study based on the full S1 and partial S1.
Amino acid analysis
Similar to the nucleotide sequences, full S1 amino acid sequences had a lower similarity compared to partial sequences overall (). Strain comparisons showed that M41 had 77% and 73% similarity to D274 and 793B, respectively, compared to the partial amino acid sequence where similarity was higher (80% for both). IS/885/00 showed 82% (full) and 83% (partial) similarity compared with IS/1494/06. Similarly, Q1 had 77% (full) and 79% (partial) similarity compared with QX.
Table 4. Amino acid identity (%) comparison of the seven IBV strains used in this study based on the full S1 and partial S1.
Sensitivity of IBV detection following inoculation onto FTA cards
IBV detection using both full and partial S1 RT-PCR varied between strains (). Partial RT-PCR detected all strains, at all temperatures, up to 21 dpi. However, the assay was the least sensitive for the FTA cards stored at 40°C at 21 dpi. For example, IS/1494/06 was only detected at the neat concentration for 40°C, compared to room temperature (10−3 dilution factor; 103.5 CD50/ml) and 4°C (10−4 dilution factor; 102.5 CD50/ml).
Table 5. IBV detection limits (CD50/ml) using the partial S1 assay from FTA cards following IBV strain inoculation and up to 21 days’ incubation at varying temperatures.
For the full S1 detection, we only detected IBV from the cards stored at 4°C and room temperature (). Additionally, the lowest dilution we identified IBV at was 10−1. No cards stored at 40°C were positive for the full S1 gene, and no IBV was detected at any temperatures past 14 dpi.
Table 6. IBV detection limits (CD50/ml) using the full S1 assay from FTA cards following IBV strain inoculation and up to 21 days of storage at different temperatures.
Sensitivity of IBV detection from field samples
Of the 25 tested samples, 76% were from tissue samples and 24% from swabs. We were able to amplify the full S1 gene for three samples (12%), whereas all samples were positive using partial S1 amplification.
Discussion
The current study aimed to establish and test a universal RT-PCR scheme used for detection and sequencing of the full S1 gene of six globally distributed IBV strains (M41, 793B, D274, IS/1494/06, IS/885/00 and Q1). The common practice for IBV research and diagnosis is to extract RNA for RT-PCR and sequencing. Subsequently, part of the S1 gene is analysed and cross-compared for differentiation between the strains (Wang & Huang, Citation2000). Many laboratories rely on a partial S1 gene assay, but a single full S1 gene assay capable of amplifying a number of common IBV serotypes has not been reported to date. One reason is that conserved sequences of approximately 20 nucleotides within the S1 gene are limited among genetically different IBV strains, decreasing the availability of applicable universal oligonucleotides (Adzhar et al., Citation1996).
The full S1 protocol described in this study gave a lower average of S1 nucleotide similarity between genotypes when compared to the partial S1 assay, demonstrating that full S1 sequencing is a more accurate method to differentiate between strains. This is partly due to a single nucleotide variation affecting 0.3% similarity for a partial sequence (350 bp), compared to 0.06% for a full S1 gene sequence (1600 bp). Such changes may cause an alteration in the virulent properties (Fang et al., Citation2005).
For research, and more widely for the diagnostic differentiation of IBVs, genotyping is commonly conducted using a partial sequence containing a hypervariable region of S1. However, such an approach shows inconsistencies compared to full S1 genotyping (Mo et al., Citation2013). Importantly, phylogenetic assessment in this study demonstrated that both the full and partial sequencing were able to distinguish individual genotypes. However, it should be noted that relying solely on partial sequences for phylogeny may give a false representation, as comparisons are dependent on the region sequenced. As a result, using the full S1 gene sequence demonstrates a better representation of strain differences (Valastro et al., Citation2016). Due to this, using the full S1 sequence when possible would be advantageous and would allow for more accurate comparisons between studies.
Not all laboratories are able to isolate and propagate IBV. Instead, a number of countries are dependent on utilizing FTA cards to transport potential IBV-positive samples (such as tracheal or oropharyngeal swabs, tissues of lungs, caecal tonsil and kidneys) for diagnosis, as these cards are a safe method of storing and shipping genomic material (Ganapathy et al., Citation2015). To date, there has been no published attempt to extract and sequence a full S1 genome from samples embedded onto FTA cards. This study showed that, for RNA extracted from AF or FTA cards, the partial S1 RT-PCR demonstrated greater detection efficiency compared to the full S1. This may be due to the significant difference in amplicon length: 0.39 and 1.72 kb for the partial and full S1 gene, respectively. Successful molecular characterization of small sequence lengths (383 bp) of virus RNAs from FTA cards, including those that were stored under non-favourable environmental conditions (at 41°C for at least 15 days), has been previously demonstrated (Moscoso et al., Citation2005). In addition, recent work has shown difficulty in amplifying a viral nucleotide product greater than 900 bp, despite detecting a 290 bp product from the same sample after six months of storage (Sakai et al., Citation2015). This work has shown that the partial assay was able to detect IBV RNA inoculated onto FTA cards for up to 21 dpi for all storage conditions, including at 40°C – a common summer temperature in many Middle East countries. In contrast, this was not possible for the full S1 gene (1700 bp), even when applying the phenol-chloroform method for extraction (Awad et al., Citation2014). Furthermore, incubating the FTA cards in TE buffer overnight for further RNA elution, accompanied with occasional vortexing, resulted in no change in the amount of viral load when quantified using qRT-PCR. From data presented here, it appears that the sensitivity for the larger target (full S1) detection is affected by virus titre, and duration and temperature of storage.
Following the successful detection and sequencing of partial and full S1 genes of 793B, M41, D274, QX, Q1, IS/885/00 and IS/1494/06, an attempt to utilize the above S1 protocols for field samples collected via FTA cards from the Middle East (Ganapathy et al., Citation2015) was carried out. From a total number of 25 partial S1-positive IBV samples, only three samples were positive via similarity to the full S1. This detection level reflects the low sensitivity of this assay when FTA card samples were stored in different conditions to this study. Further optimization of the full S1 RT-PCR, including optimization of RNA extraction from the FTA cards, may be necessary to increase detection efficiency. In our study, we attempted to mimic field practices, where oropharyngeal swabs or tissue samples collected at necropsy or IBV-enriched AFs are embedded onto FTA cards, then stored and/or transported at different temperatures to local or international laboratories for detection of IBVs. It appears that temperatures up to 40°C, with storage for up to 21 days, have no negative impact on the recovery of sufficient RNA for a successful partial S1 RT-PCR and sequencing. However, it recovers inadequate RNA for a full S1 RT-PCR or sequencing.
In conclusion, the full S1 scheme presented in this study is mostly suitable for IBV-enriched samples (e.g. AF). Clinical samples (either swabs or tissues) that have been directly obtained from the field or in vivo experiments, including those imprinted on FTA cards, are unlikely to provide sufficient genome quantities for the full S1 detection. The partial S1 scheme was effective in detecting and differentiating IBV in enriched AFs and those embedded onto FTA cards.
Supplemental Material
Download PNG Image (626.5 KB)Disclosure statement
No potential conflict of interest was reported by the authors.
Additional information
Funding
References
- Abdel-Moneim, A.S., El-Kady, M.F., Ladman, B.S. & Gelb, J., Jr. (2006). S1 gene sequence analysis of a nephropathogenic strain of avian infectious bronchitis virus in Egypt. Virology Journal, 3, 78. doi: 10.1186/1743-422X-3-78
- Abro, S.H., Renstrom, L.H., Ullman, K., Isaksson, M., Zohari, S., Jansson, D.S., Belak, S. & Baule, C. (2012). Emergence of novel strains of avian infectious bronchitis virus in Sweden. Veterinary Microbiology, 155, 237–246. doi: 10.1016/j.vetmic.2011.09.022
- Adzhar, A., Shaw, K., Britton, P. & Cavanagh, D. (1996). Universal oligonucleotides for the detection of infectious bronchitis virus by the polymerase chain reaction. Avian Pathology, 25, 817–836. doi: 10.1080/03079459608419184
- Al-Shekaili, T., Baylis, M. & Ganapathy, K. (2015). Molecular detection of infectious bronchitis and avian metapneumoviruses in Oman backyard poultry. Research in Veterinary Science, 99, 46–52. doi: 10.1016/j.rvsc.2014.12.018
- Awad, F., Baylis, M., Jones, R.C. & Ganapathy, K. (2014). Evaluation of Flinders Technology Associates cards for storage and molecular detection of avian metapneumoviruses. Avian Pathology, 43, 125–129. doi: 10.1080/03079457.2014.885114
- Awad, F., Forrester, A., Baylis, M. & Ganapathy, K. (2012). Detection of multiple poultry respiratory pathogens from FTA® card. In M. Leirz, U. Huffels-Redman, E.F. Kaleta, & J. Heckman (Eds.), VII International Symposium on Avian Corona and Pneumovirus Infections (pp. 136–137). Rauischholzhausen: Druckerei Schroder.
- Ball, C., Forrester, A. & Ganapathy, K. (2016). Detection of variant infectious bronchitis viruses in Sri Lanka (2012–2015). Archives of Virology, 161, 1697–1699. doi: 10.1007/s00705-016-2831-x
- Benyeda, Z., Mato, T., Suveges, T., Szabo, E., Kardi, V., Abonyi-Toth, Z., Rusvai, M. & Palya, V. (2009). Comparison of the pathogenicity of QX-like, M41 and 793/B infectious bronchitis strains from different pathological conditions. Avian Pathology, 38, 449–456. doi: 10.1080/03079450903349196
- Cavanagh, D. (2003). Severe acute respiratory syndrome vaccine development: experiences of vaccination against avian infectious bronchitis coronavirus. Avian Pathology, 32, 567–582. doi: 10.1080/03079450310001621198
- Cavanagh, D. (2005). Coronaviruses in poultry and other birds. Avian Pathology, 34, 439–448. doi: 10.1080/03079450500367682
- Chhabra, R., Forrester, A., Lemiere, S., Awad, F., Chantrey, J. & Ganapathy, K. (2015). Mucosal, cellular, and humoral immune responses induced by different live infectious bronchitis virus vaccination regimes and protection conferred against infectious bronchitis virus Q1 strain. Clinical and Vaccine Immunology, 22, 1050–1059. doi: 10.1128/CVI.00368-15
- Cook, J.K.A., Darbyshire, J.H. & Peters, R.W. (1976). The use of chicken tracheal organ cultures for the isolation and assay of avian infectious bronchitis virus. Archives of Virology, 50, 109–118. doi: 10.1007/BF01318005
- Cook, J.K.A., Orbell, S.J., Woods, M.A. & Huggins, M.B. (1996). A survey of the presence of a new infectious bronchitis virus designated 4/91 (793B). Veterinary Record, 138, 178–180. doi: 10.1136/vr.138.8.178
- Crinion, R.A. & Hofstad, M.S. (1972). Pathogenicity of four serotypes of avian infectious bronchitis virus for the oviduct of young chickens of various ages. Avian Diseases, 16, 351–363. doi: 10.2307/1588800
- Cumming, R.B. (1963). Infectious avian nephrosis (uraemia) in Australia. Australian Veterinary Journal, 39, 145–147. doi: 10.1111/j.1751-0813.1963.tb04255.x
- Davelaar, F., Kouwenhoven, B. & Burger, A. (1984). Occurrence and significance of infectious bronchitis virus variant strains in egg and broiler production in the Netherlands. Veterinary Quarterly, 6, 114–120. doi: 10.1080/01652176.1984.9693924
- De Wit, J., Cook, J.K. & Van der Heijden, H.M. (2011). Infectious bronchitis virus variants: a review of the history, current situation and control measures. Avian Pathology, 40, 223–235. doi: 10.1080/03079457.2011.566260
- Falchieri, M., Lupini, C., Cecchinato, M., Catelli, E., Kontolaimou, M. & Naylor, C.J. (2013). Avian metapneumoviruses expressing infectious bronchitis virus genes are stable and induce protection. Vaccine, 31, 2565–2571. doi: 10.1016/j.vaccine.2013.03.055
- Fang, S.G., Shen, S., Tay, F.P.L. & Liu, D.X. (2005). Selection of and recombination between minor variants lead to the adaptation of an avian coronavirus to primate cells. Biochemical and Biophysical Research Communications, 336, 417–423. doi: 10.1016/j.bbrc.2005.08.105
- Ganapathy, K., Ball, C. & Forrester, A. (2015). Genotypes of infectious bronchitis viruses circulating in the Middle East between 2009 and 2014. Virus Research, 210, 198–204. doi: 10.1016/j.virusres.2015.07.019
- Ganapathy, K., Wilkins, M., Forrester, A., Lemiere, S., Cserep, T., McMullin, P. & Jones, R.C. (2012). QX-like infectious bronchitis virus isolated from cases of proventriculitis in commercial broilers in England. Veterinary Record, 171, 597. doi: 10.1136/vr.101005
- Gelb, J., Weisman, Y., Ladman, B.S. & Meir, R. (2005). S1 gene characteristics and efficacy of vaccination against infectious bronchitis virus field isolates from the United States and Israel (1996 to 2000). Avian Pathology, 34, 194–203. doi: 10.1080/03079450500096539
- Huang, Y.-P., Lee, H.-C., Cheng, M.-C. & Wang, C.-H. (2004). S1 and N gene analysis of avian infectious bronchitis viruses in Taiwan. Avian Diseases, 48, 581–589. doi: 10.1637/7186-033004R
- Ignjatovic, J. & Sapats, S. (2000). Avian infectious bronchitis virus. Revue Scientifique et Technique, 19, 493–508. doi: 10.20506/rst.19.2.1228
- Jackwood, M.W. (2012). Review of infectious bronchitis virus around the world. Avian Diseases, 56, 634–641. doi: 10.1637/10227-043012-Review.1
- Jackwood, M.W. & de Wit, J.J. (2013). Infectious bronchitis. In D.E. Swayne, J.R. Glisson, L.R. McDougald, J.R. Glisson, L.K. Nolan, D.L. Suarez, & V. Nair (Eds.), Diseases of Poultry 13th edn. (pp. 139–159). Ames: John Wiley.
- Johnson, M.A., Pooley, C., Ignjatovic, J. & Tyack, S.G. (2003). A recombinant fowl adenovirus expressing the S1 gene of infectious bronchitis virus protects against challenge with infectious bronchitis virus. Vaccine, 21, 2730–2736. doi: 10.1016/S0264-410X(03)00227-5
- Jungherr, E.L., Chomiak, T.W. & Luginbuhl, R.E. (1956). Proceedings of the 60th annual meeting of United States Livestock Sanitary Association Immunologic Differences in Strains of Infectious Bronchitis (pp. 203–209), Chicago, Illinois.
- Kamble, N.M., Pillai, A.S., Gaikwad, S.S., Shukla, S.K., Khulape, S.A., Dey, S. & Mohan, C.M. (2016). Evolutionary and bioinformatic analysis of the spike glycoprotein gene of H120 vaccine strain protectotype of infectious bronchitis virus from India. Biotechnology and Applied Biochemistry, 63, 106–112. doi: 10.1002/bab.1298
- Kingham, B.F., Keeler, C.L., Jr., Nix, W.A., Ladman, B.S. & Gelb, J., Jr. (2000). Identification of avian infectious bronchitis virus by direct automated cycle sequencing of the S-1 gene. Avian Diseases, 44, 325–335. doi: 10.2307/1592547
- Kuo, S.M., Wang, C.H., Hou, M.H., Huang, Y.P., Kao, H.W. & Su, H.L. (2010). Evolution of infectious bronchitis virus in Taiwan: characterisation of RNA recombination in the nucleocapsid gene. Veterinary Microbiology, 144, 293–302. doi: 10.1016/j.vetmic.2010.02.027
- Kwon, H.M., Jackwood, M.W. & Gelb, J., Jr. (1993). Differentiation of infectious bronchitis virus serotypes using polymerase chain reaction and restriction fragment length polymorphism analysis. Avian Diseases, 37, 194–202. doi: 10.2307/1591474
- Ladman, B.S., Loupos, A.B. & Gelb, J., Jr. (2006). Infectious bronchitis virus S1 gene sequence comparison is a better predictor of challenge of immunity in chickens than serotyping by virus neutralization. Avian Pathology, 35, 127–133. doi: 10.1080/03079450600597865
- Mahmood, Z.H., Sleman, R.R. & Uthman, A.U. (2011). Isolation and molecular characterization of Sul/01/09 avian infectious bronchitis virus, indicates the emergence of a new genotype in the Middle East. Veterinary Microbiology, 150, 21–27. doi: 10.1016/j.vetmic.2010.12.015
- Meir, R., Rosenblut, E., Perl, S., Kass, N., Ayali, G., Perk, S. & Hemsani, E. (2004). Identification of a novel nephropathogenic infectious bronchitis virus in Israel. Avian Diseases, 48, 635–641. doi: 10.1637/7107
- Mo, M.L., Li, M., Huang, B.C., Fan, W.S., Wei, P., Wei, T.C., Cheng, Q.Y., Wei, Z.J. & Lang, Y.H. (2013). Molecular characterization of major structural protein genes of avian coronavirus infectious bronchitis virus isolates in southern China. Viruses, 5, 3007–3020. doi: 10.3390/v5123007
- Moscoso, H., Raybon, E.O., Thayer, S.G. & Hofacre, C.L. (2005). Molecular detection and serotyping of infectious bronchitis virus from FTA filter paper. Avian Diseases, 49, 24–29. doi: 10.1637/7220
- Muneer, M.A., Chaudhry, K.M. & Khawaja, K.N. (2000). Losses due to infectious bronchitis virus infection in laying and breeding hens. Pakistan Veterinary Journal, 20, 64–70.
- Parsons, D., Ellis, M.M., Cavanagh, D. & Cook, J.K. (1992). Characterisation of an infectious bronchitis virus isolated from vaccinated broiler breeder flocks. Veterinary Record, 131, 408–411. doi: 10.1136/vr.131.18.408
- Pohuang, T., Chansiripornchai, N., Tawatsin, A. & Sasipreeyajan, J. (2011). Sequence analysis of S1 genes of infectious bronchitis virus isolated in Thailand during 2008-2009: identification of natural recombination in the field isolates. Virus Genes, 43, 254–260. doi: 10.1007/s11262-011-0635-3
- Sakai, T., Ishii, A., Segawa, T., Takagi, Y., Kobayashi, Y. & Itou, T. (2015). Establishing conditions for the storage and elution of rabies virus RNA using FTA((R)) cards. Journal of Veterinary Medical Science, 77, 461–465. doi: 10.1292/jvms.14-0227
- Tamura, K., Stecher, G., Peterson, D., Filipski, A. & Kumar, S. (2013). MEGA6: Molecular Evolutionary Genetics Analysis version 6.0. Molecular Biology and Evolution, 30, 2725–2729. doi: 10.1093/molbev/mst197
- Toffan, A., Bonci, M., Bano, L., Bano, L., Valastro, V., Vascellari, M., Capua, I. & Terregino, C. (2013). Diagnostic and clinical observation on the infectious bronchitis virus strain Q1 in Italy. Veterinaria Italiana, 49, 347–355.
- Valastro, V., Holmes, E.C., Britton, P., Fusaro, A., Jackwood, M.W., Cattoli, G. & Monne, I. (2016). S1 gene-based phylogeny of infectious bronchitis virus: an attempt to harmonize virus classification. Infection, Genetics and Evolution, 39, 349–364. doi: 10.1016/j.meegid.2016.02.015
- Wang, C.H. & Huang, Y.C. (2000). Relationship between serotypes and genotypes based on the hypervariable region of the S1 gene of infectious bronchitis virus. Archives of Virology, 145, 291–300. doi: 10.1007/s007050050024
- Wang, C.H. & Tsai, C.T. (1996). Genetic grouping for the isolates of avian infectious bronchitis virus in Taiwan. Archives of Virology, 141, 1677–1688. doi: 10.1007/BF01718291
- Williams, A.K., Li, W., Sneed, L.W. & Collisson, E.W. (1992). Comparative analyses of the nucleocapsid genes of several strains of infectious bronchitis virus and other coronaviruses. Virus Research, 25, 213–222. doi: 10.1016/0168-1702(92)90135-V
- Worthington, K.J., Currie, R.J. & Jones, R.C. (2008). A reverse transcriptase-polymerase chain reaction survey of infectious bronchitis virus genotypes in Western Europe from 2002 to 2006. Avian Pathology, 37, 247–257. doi: 10.1080/03079450801986529
- Yu, L., Wang, Z., Jiang, Y., Low, S. & Kwang, J. (2001). Molecular epidemiology of infectious bronchitis virus isolates from China and Southeast Asia. Avian Diseases, 45, 201–209. doi: 10.2307/1593029
- Zhang, T., Han, Z., Xu, Q., Wang, Q., Gao, M., Wu, W., Shao, Y., Li, H., Kong, X. & Liu, S. (2015). Serotype shift of a 793/B genotype infectious bronchitis coronavirus by natural recombination. Infection, Genetics and Evolution, 32, 377–387. doi: 10.1016/j.meegid.2015.03.034