ABSTRACT
Bone fractures in birds are usually diagnosed with the aid of traditional radiography. However, this technique remains limited because of the difficulties associating this examination with real-time procedures. The aim of this study was to describe the use of B-mode ultrasound to assess the long bones of two avian orders. For the study, we used carcases of birds from the orders Falconiformes (n = 9) and Strigiformes (n = 12), with weights ranging from 108 to 1020 g. An ultrasound device with a 5–12 MHz linear probe was employed to produce images of the long bones (humerus, radius, ulna, femur and tibiotarsus). Ultrasound (US) measurements and physical measurements using a caliper were applied to compare the diameter of the bones. Images were also recorded from the US examination performed in two live patients attending the hospital with suspected bone fractures. No statistical difference was found between the two methods of measurement in carcases weighing up to 267 g (P > 0.01). The US examination provided relevant clinical information about the bone cortex and assisted in real-time surgical procedures.
RESEARCH HIGHLIGHTS
Long bones of Falconiformes and Strigiformes birds were assessed with B-mode ultrasound.
Ultrasound analysis is a relevant tool in clinical orthopaedics for avian species.
Ultrasound of the bone might be applied for monitoring of healing processes.
Introduction
Bone injuries in birds are of great concern due to their high prevalence and complex recovery. Particularly troubling are the fractures of the long bones of wings and legs, as the restitution of normal biomechanics is fundamental to preserve the hunting and flying capacities (Orosz, Citation2002). The diagnosis of bone injuries in birds is mostly performed with the aid of traditional radiography. However, radiation and the incapacity to associate the examination to real-time procedures are some of the disadvantages of this technique (Douma-den Hamer et al., Citation2016). The use of ultrasound (US) in orthopaedic practice is controversial, with most studies related to human medicine. For instance, Bolandparvaz et al. (Citation2013) reported that US cannot replace radiography in the diagnosis of bone fractures. However, others indicate US as a follow-up method for fracture healing assessment due to its relevance in orthopaedic practice as a rapid and accurate examination to identify early and minor changes due to long bone fractures (Patel & Tollefson, Citation2013; Kachewar & Kulkarni, Citation2014). Although studies performed in domestic mammals such as dogs and cats have corroborated the value of US in musculoskeletal disorders (Risselada et al., Citation2007), data on avian species are scarce. Avian species are characterized by having a thinner cortex, fewer surrounding structures and thinner skin than mammals (Orosz, Citation2002). Such anatomic differences may necessitate variation in the US technique. The purpose of this study was to explore the use of B-mode ultrasonography for the examination of the long bones (wings and legs: humerus, radius, ulna, femur and tibiotarsus) from raptor birds. The study was divided into two sections. The first section used bird carcases to determine positioning of the animal, US assessment of bone structures and US acquired measurements compared to those obtained with calipers. The second section involved the inclusion of this technique during orthopaedic surgery of a barn owl (Tyto furcata) and the examination of a tropical screech owl (Megascops choliba) with subcutaneous haemorrhage in the region of the right tibia.
Materials and methods
This project is registered in the Biodiversity Information and Authorization System (SisBio) N° 6635312. Carcases from adult Falconiformes (n = 9) and Strigiformes (n = 12) birds were thawed and examined at the veterinary hospital of the Universidade Federal do Pará (Brazil). The species used from the Falconiformes order were four hook-billed kites (Chondrohierax uncinatus), three roadside hawks (Rupornis magnirostris) and two savanna hawks (Buteogallus meridionalis). From the Strigiformes order were five barn owls (T. furcata), three tropical screech owls (M. choliba), two spectacled owls (Pulsatrix perspicillata) and two burrowing owls (Athene cunicularia).
The carcases were obtained from animals that arrived at the hospital for medical attention, but died during treatment. They were stored in a −20°C freezer at the anatomical collection of the university. Before death, one individual from the species A. cunicularia had lost its upper limbs as a consequence of traumatic lesions. Therefore, only femur and tibiotarsus were evaluated in this animal.
Ultrasound images were also obtained from two clinical cases of injured raptors, a barn owl and a tropical screech owl, with complete fractures of femur and tibiotarsus, respectively. This procedure was done to determine the applicability of US examination in clinical cases. A single ultrasonographer conducted all the examinations.
A Philips HDI 4000 (Philips Medical Systems, Bothell, WA, USA) US with a 5–12 MHz linear probe was used to produce and record images of the selected bones: humerus (H), ulna (U), radius (R), femur (F) and tibiotarsus (TT). The tarsometatarsus was omitted since the scales in the skin around the tibiotarsus partially reflected the waves and did not allow the proper visualization of the structures. A stand-off pad, besides not being available, would not be useful in this case. The area of interest was cleaned, the feathers were either displaced or clipped depending on the examined region, and US gel (Aquasonics, Parker Laboratories, Fairfield, NJ, USA) was applied before the start of the examination. Each bird carcase or live bird was placed in dorsal recumbency with the head toward the operator and the limb to be evaluated uppermost for all approaches. For the orthopaedic surgery of the barn owl, tramadol hydrochloride (5 mg/kg) was administered as a preanaesthetic medication and the anaesthetic induction was made with isofluorane using a mask. The examination of the tropical screech owl with fracture of tibiotarsus, due to its critical status, was performed without anaesthesia, but it was performed efficiently and succinctly to reduce patient stress. The digital calipers within the US software were used to measure the diameter (perpendicular to the long axis) of the bone from the interface of the bone surface and soft tissues to the contralateral bone surface and soft tissue interface ((A)). To confirm the visualization of a complete section of the bone, a metallic pin was placed on the opposite side of the probe as an anatomical landmark, demonstrating the passage of the waves through the bone tissues when the metal pin was visualized on the screen. Then, bones were dissected and measured by a caliper as a reference value. Both US and caliper measurements were obtained from the midpoint of the diaphysis of the bone and the data were compared using a paired t-test. Differences were considered statistically significant when P < 0.01. For the raptor that did not have the forelimbs, only the data collected from the hindlimbs were statistically compared.
Figure 1. A. Lateral longitudinal view of the femur of a burrowing owl (A. cunicularia). The bone surface of both sides of the cortex (arrows) and the medullary cavity in between can be observed. B. Lateral view of the humerus of a spectacled owl (P. perspicillata). The image shows a cortex in the near field (white arrow) and deep, parallel reverberation artefacts (arrowheads) preventing visualization of deeper structures.
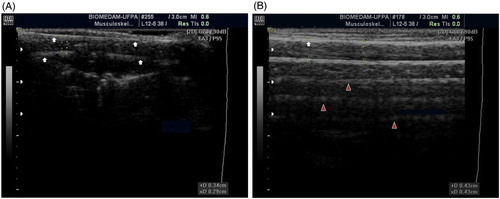
Results and discussion
A total of 431 images were obtained for this study. The selected approaches from each bone, looking for accessible US regions where fewer feathers had to be removed, are shown in . Ultrasound depth varied between 2.4 and 4.0 cm for all species. The species weighing less than 350 g, such as burrowing owl (152 ± 7.5 ), hook-billed kite (261 ± 6.7 g), tropical screech owl (108 ± 6.1 g) and roadside hawk (246 ± 8.2 g) were labelled as small species and a higher gain was used when compared with the larger species. Images of smaller species showed a greater anatomical correspondence, as the waves penetrated the bone surface displaying a complete bone section including both faces of a defined, hyperechogenic cortex and, in the middle, the respective medullary cavity ((A)). Even though the cortex in the far-field of the image was less echogenic, the quality of image allowed a satisfactory clinical examination ((A)). For the small species, no statistical significant differences between the measurements of the caliper and US were found, as shown in .
Figure 2. A. Lateromedial view of the tibiotarsus of a hook-billed kite (C. uncinatus). The image shows an incomplete fracture (confirmed after bone dissection) in one of the bone faces while the other face remains intact. B. Lateromedial view of the tibiotarsus of a burrowing owl (A. cunicularia). A metallic object can be observed as an echogenic dot deep to the second face of the bone cortex (arrowhead) confirming the passage of the ultrasound waves through the bone cortex.
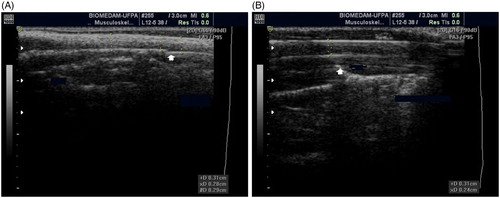
Table 1. Sonographic approaches for each raptor long bone.
Table 2. Mean (±SD) diameter (cm) of the bones from adult raptors of four different species.
On the other hand, species that weighed greater than or equal to 350 g, e.g. savanna hawk (939 ± 41 g), spectacled owl (680 ± 9.0 g) and barn owl (392 ± 12 g), were considered as large species, and a lower gain was preferred due the highly reflective interface. The bone surface was visualized as a sharp, echogenic, continuous line and structures beyond it were rarely displayed on the screen. In large species, reverberation artefacts and acoustic shadowing were observed, preventing consistent ultrasound examination and diameter measurements. Therefore, it was not possible to perform statistical comparisons for this group of birds. Nevertheless, the image offered objective information in relation to the morphology and topography of the bone surface ((B)). A metal pin was imaged in the medullary cavity and deep to the bone section, as a dynamic, hyperechoic dot or line depending on the angle of intonation, verifying the complete visualization of structures beyond the bone surface ((B)). When reverberation occurred, the bone morphology deep to the reverberation was not reflected and neither were metal objects deeper from the bone fracture. In burrowing owl and tropical screech owl, the epiphyses of the bone were visualized. However, given their irregular and denser surface, the image was less distinct. The contour of the bone epiphyses was observed in hook-billed kite and roadside hawk with an acoustic shadow deep to the bone surface ((A–B)). The US examination performed in two live patients allowed the visualization of bone fracture as a disruption in the continuity of the bone surface ((A)). The US allowed real-time monitoring of the manual approximation and surgical fixation of the bone fragments in a barn owl, while displaying the bone section and the pin inserted in the medullary cavity ((B)). Large birds have long bones that are easily accessible to palpation when presenting fractures that are usually complete and often exposed, facilitating the diagnosis (Helmer & Redig, Citation2006). The detection of subtle changes in soft tissues, or the monitoring of a bone healing process, are US utilities that must be considered in order to determine the actual relevance of this examination for the avian patient. Therefore, our next step will be to monitor fractures in live patients, throughout the time of their recovery, applying US. Importantly, this study demonstrated the viability of the US examination in the avian patient and the obtained results were comparable with studies in humans and other animals (Risselada et al., Citation2003; Maschburn et al., Citation2004; Risselada et al., Citation2007; Patel & Tollefson, Citation2013). The US provided useful information about the bone tissue that would potentially allow the avian veterinarian to make decisions and monitor procedures in real time and on a routine basis. The US measurements in small animals may improve the precision of medical and surgical procedures or simply be used as a dynamic biometric tool.
Figure 3. A. Lateral view of the humerus of a roadside hawk (R. magnirostris). It is possible to visualize the deep cortex of the diaphysis (white arrow) but not the epiphysis. There is acoustic shadowing preventing visualization of the deeper cortex (arrowhead). B. Craniocaudal view of the femur of a tropical screech owl (M. choliba). The bone diaphysis (arrows) and epiphysis (arrowhead) are shown.
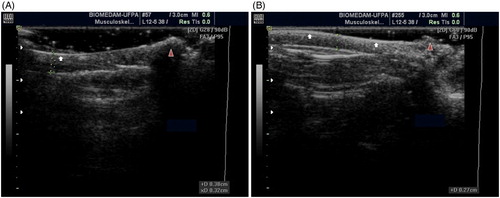
Figure 4. A. Craniocaudal view of the tibiotarsus of a tropical screech owl (M. choliba); the animal arrived at the hospital prostrate and with subcutaneous haemorrhage in the tibial region of the right leg. The image shows a complete oblique fracture with displacement in the mid-diaphysis of the bone (arrows). B. Lateral view of the femur of a barn owl (T. furcata); a metallic pin (white arrow) appears as a linear hyperechoic structure between the near and far cortices of the diaphysis (arrowhead). The image was obtained during the surgical correction of a complete oblique fracture.
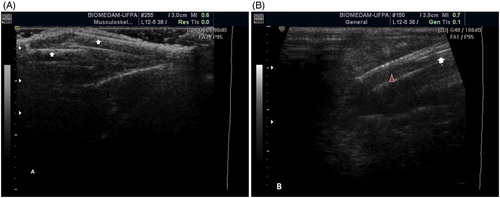
Disclosure statement
No potential conflict of interest was reported by the authors.
References
- Bolandparvaz, S., Moharamzadeh, P., Jamali, K., Pouraghaei, M., Fadaje, M., Sefjdbakht, S. & Shahsavari, K. (2013). Comparing diagnostic accuracy of bedside ultrasound and radiography for bone fracture screening in multiple trauma patients. American Journal of Emergency Medicine, 31, 1583–1585. doi: 10.1016/j.ajem.2013.08.005
- Douma-den Hamer, D., Blanker, M.H., Edens, M.A., Buijteweg, L.N., Boomsma, M.F., van Helden, S.H., Mauritz, G.J. & van Griensven, M. (2016). Ultrasound for distal forearm fracture: a systematic review and diagnostic meta-analysis. PLoS One, 11, e0155659. doi: 10.1371/journal.pone.0155659
- Helmer, P. & Redig, P.T. (2006). Surgical resolution of orthopedic disorders. In G. Harrison & T. Lightfoot (Eds.), Clinical Avian Medicine Online 1st edn (pp. 761–774). Palm Beach, FL: Spix Publishing.
- Kachewar, S.G. & Kulkarni, D.S. (2014). Utility of diagnostic ultrasound in evaluating fracture healing. Journal of Clinical and Diagnostic Research, 8, 179–180.
- Marshburn, T., Legome, E., Sargsyan, A., Li, S.M., Noble, V.A., Dulchavsky, S.A., Sims, C. & Robinson, D. (2004). Goal directed ultrasound in the detection of long bone fractures. Journal of Trauma, 57, 329–332. doi: 10.1097/01.TA.0000088005.35520.CB
- Orosz, S. (2002). Clinical considerations of the thoracic limb. Orthopedics, 5, 31–48.
- Patel, R.M. & Tollefson, B.J. (2013). Bedside ultrasound detection of long bone fractures. Journal of the Mississippi State Medical Association, 54, 159–162.
- Risselada, M., Kramer, M. & Van Bree, H. (2003). Approaches for ultrasonographic evaluation of long bones in the dog. Veterinary Radiology and Ultrasound, 44, 214–220. doi: 10.1111/j.1740-8261.2003.tb01274.x
- Risselada, M., Van Bree, H., Kramer, M., Duchateau, L., Verleven, P. & Saunders, J.H. (2007). Ultrasonographic assessment of fracture healing after plate osteosynthesis. Veterinary Radiology and Ultrasound, 48, 368–372. doi: 10.1111/j.1740-8261.2007.00258.x