ABSTRACT
Avian influenza virus (AIV) can cause serious zoonotic disease, thereby threatening the poultry industry and human health. An efficient and rapid detection approach is crucial to prevent and control the spread of avian influenza. In this study, a novel protein microarray was developed. Haemagglutinin proteins of H5 and H7 subtypes and nucleoprotein (NP) were purified and spotted onto the initiator-integrated poly-(dimethylsiloxane) as antigens. Monoclonal antibodies with inhibition effect were screened and utilized for the synchronous detection of three avian influenza antibodies in different species. In the protein microarray, the cut-off values were 40%, 50% and 30% inhibition for H5 antibody detection; 50%, 50% and 20% for NP antibody detection; 40%, 50% and 40% for H7 antibody detection in chicken, peacock and duck sera, respectively. The 95 serum samples were detected by microarray, and results were compared with the findings of AIV antibody test enzyme-linked immunosorbent assay (ELISA) or haemagglutination inhibition (HI) test. NP antibody detection in the microarray showed 100% (55/55) agreement ratio in chicken using ELISA. Compared with HI, H5 antibody detection in the microarray showed 100% (95/95) agreement ratio in chicken, peacock and duck, whilst those of H7 displayed 98.18% (54/55) agreement in chicken, 100% (20/20) in peacock and 90% (18/20) in duck. In conclusion, this novel protein microarray is a high-throughput and specific method for the detection of AIV antibodies and simultaneous distinction of antibodies against H5 and H7 subtypes. It can be applied to the serological diagnosis and epidemiological investigation of AIV.
RESEARCH HIGHLIGHTS
A novel protein microarray method has been developed.
The microarray can detect AIV antibodies and distinguish between H5 and H7 subtypes.
The study lays the foundation for simultaneous identification of multiple pathogens.
Introduction
Avian influenza virus (AIV) is a negative-sense single-stranded RNA virus that belongs to the influenza virus A genus of the Orthomyxoviridae family (Jarocka et al., Citation2014). AIV is a highly infectious disease that can occur in pandemics and localized outbreaks and can cause severe economic losses to the poultry industry (Capua & Alexander, Citation2004; Wade et al., Citation2018). AIV can cross species barriers and can infect humans, especially subtypes H5 and H7, thereby seriously threatening individual and global health (Li et al., Citation2015). In 2013, >130 cases of H7N9 human infection were confirmed in China, 37 of which died. The age distribution and source of human H7 infection cases have gradually changed, but the clinical symptoms have remained severe since 2013 (Wang et al., Citation2017). H5N1 constantly infects susceptible species, and the first case of human infection with H5N1, was reported in 1997 (Claas et al., Citation1998; Li et al., Citation2015; Van Kerkhove, Citation2013). H5N1 attracted major public attention because it can cause a high mortality rate in humans (Pang et al., Citation2015). The rapid detection of AIV and accurate identification of H5 and H7 subtypes are needed for the surveillance of the emergence of new viruses, outbreak management and disease control.
Different types of detection methods have been applied for influenza viruses. Nucleic acid-based detection methods, such as polymerase chain reaction (PCR), reverse-transcription PCR and real-time PCR, have been universally used in laboratories (Letant et al., Citation2007; Stone et al., Citation2004). Multiplex PCR has been rapidly developed because it can detect multiple viruses in one tube (Laamiri et al., Citation2016; Liu et al., Citation2018). Haemagglutination inhibition (HI) test is still recognized as the gold standard method for influenza virus serological detection, but the preparation of red blood cells is complicated (Hirst, Citation1942). Enzyme-linked immunosorbent assay (ELISA) was developed based on antigen–antibody specific reaction and is widely applied because it is high-throughput and convenient (Chappell et al., Citation2014; Hemmatzadeh et al., Citation2013). However, ELISA has significant limitations in the simultaneous detection of numerous subtypes and strains of AIV. As a result of technological development, protein- or gene-microarray approaches have shown considerable potential for disease diagnosis, and can enhance detection quality and shorten analysis duration. These approaches can satisfy the needs of simultaneous multiple virus detection within a single experiment and can screen large numbers of pathological samples (Wang et al., Citation2010).
In this study, we described a protein microarray approach to detect various subtypes of AIV antibodies and simultaneously distinguish the antibodies of H5 and H7 subtypes. Initiator integrated poly-(dimethysiloxane) (iPDMS) membrane, as a novel material, was utilized in protein microarray to reduce nonspecific protein adsorption to an absolute zero level (Ma et al., Citation2010). Three monoclonal antibodies (mAbs; against H5, H7 and NP) with blocking effects were used as tools to establish this detection method. The blocking protein microarray is extremely similar in concept to blocking ELISA. Without changing any of the test reagents, serum detection from different susceptible hosts, including chicken, duck, peacock and other wild birds, is possible. The method boasts superior throughput, simple manipulation, high specificity and sensitivity and can be applied to epidemiological investigations and to assess the humoral response to vaccination in different species.
Materials and methods
Reagents and serum samples
The recombinant plasmids (pET-32a-H3N2-NP, pCold I -H5-HA and pCold I -H7-HA) were obtained by our laboratory, and three mAbs against H5, H7 and NP were purchased from Jianyao Biotechnology Company, Suzhou, China (Table S1). IPDMS membrane (15 × 15 mm2) was obtained from Boshi Trading Company (Suzhou, China). Horseradish peroxidase-labelled goat anti-mouse antibody (HRP-YKS) was purchased from KPL (MD, USA). Peroxidase conjugate stabilizer and chemiluminescence substrate were purchased from Thermo Fisher (Waltham, MA, USA).
Negative sera were collected from different ages of specific-pathogen-free (SPF) chickens and ducks. Positive chicken serum of AIV (H1, H2, H3, H4, H5, H6, H7, H8, H9, H11, H12, H13 and H14), Newcastle disease virus (NDV-LaSota), infectious bronchitis virus (IBV), avian leukosis virus (ALV-J) and infectious bursal disease virus (IBDV) were prepared from SPF chickens in isolators. The 75 clinical serum samples from chicken and duck used in this study were collected from farms, and 20 peacock serum samples were obtained from Nanjing Hongshan Forest Zoo. The animals in this study were treated according to the rules described by the Animal Ethics Procedures and Guidelines of the People’s Republic of China and Institutional Animal Care and Use Committee of Nanjing Agricultural University (SYXK [Su] 2017-0007).
Instruments
The contact printer SmartArrayer 48 (CapitalBio, Beijing, China) was used to prepare the protein microarray. Protein microarray was incubated using a temperature shaker (Thermo Fisher, USA). Microscopy images were obtained with an Amersham Imager 600 (GE, Boston, MA, USA), and signals were acquired using GenePix Pro 6.0 software.
Proteins purification
Three prokaryotic expression plasmids (pET-32a-H3N2-NP, pCold I -H5-HA and pCold I -H7-HA) were expressed in E. coli BL21 (DE3). The recombinant E. coli was induced by 1 mM isopropylthio-b-d-thiogalactoside for 10 h at 16°C. Histidine-tagged proteins were purified by affinity chromatography by using Ni-nitrilotriacetic acid agarose (Qiagen, Hilden, Germany). SDS-PAGE and western blot were performed to analyse the purified proteins. The recombinant proteins were stored at −80°C.
SDS-PAGE and western blot analysis
The protein samples were separated by 10% SDS-PAGE and either stained with Coomassie brilliant blue G-250 (Bio-Rad, Hercules, CA, USA) or transferred onto a 0.45 μm nitrocellulose membrane (Millipore, Billerica, MA, USA). The membranes were blocked with 5% skimmed milk powder in PBS containing 0.05% Tween-20 at 37°C for 1 h, incubated at 37°C for 1 h with the corresponding mAbs, then incubated with HRP-conjugated goat anti-mouse IgG for 45 min at 37°C. The immunoreactive bands were detected with ECL (Thermo, Rockford, IL, USA) detection solution and visualized using an Amersham Imager 600.
Protein microarray preparation
The purified proteins of H5-HA, H7-HA and NP with printing buffer, containing 0.3 M phosphate buffer, 0.2% glycerin, 0.01% Triton X-100 and 1.5% mannitol, were diluted at different concentrations to determine the best antigen concentration, and printing buffer was used as the negative buffer. Different protein and negative buffer concentrations were printed to the predetermined locations of the iPDMS by using a contact printer SmartArrayer 48 with approximately 0.6 nl of printing solution for each sample.
Establishment of protein microarray detection method
The protein microarray procedure is summarized in (A). First, the microarray was rinsed three times with wash buffer (TBST), and 60 μl of serum samples diluted in washing buffer were added to each well. Wash buffer without serum was used as the negative control, whilst positive reference serum was used as positive control, incubated for 30 min on the shaker at 500 rpm at 37°C and washed three times with wash buffer. Then, 100 µl of mixed mAb, diluted using peroxidase conjugate stabilizer, was added to each well, incubated at 37°C for 30 min, and washed three times. Next, the samples were incubated with 100 µL of 1 mg/ml HRP-YKS diluted 1:10,000 in peroxidase conjugate stabilizer for another 30 min on the shaker (500 rpm, 37°C), followed by the same washing steps. Finally, 15 µl of chemiluminescence substrate was added to the microarray, and images were taken at a wavelength of 645 nm by using an Amersham Imager 600. Chemiluminescent signals were acquired using GenePix Pro 6.0 software and the inhibition percentage value was calculated. The scanning image of the microarrays presented in (B) shows that the negative control spots presented strong signals, whilst the positive control did not show any signal or exhibited weak signal. Weak signals would appear if the mAbs were not completely blocked.
Figure 1. Scheme and scanning image of protein microarrays for antibody detection against AIV. (A) Scheme of protein microarrays. (B) Results of protein microarray chemiluminescent signals. 1: Negative control, 2: NDV-positive serum, 3: IBV-positive serum, 4: H7-positive serum, 5: H5-positive serum, 6: H5 + H7-positive serum, 7: H1-positive serum, 8: H6-positive serum, 9: H9-positive serum, and 10: H12-positive serum.
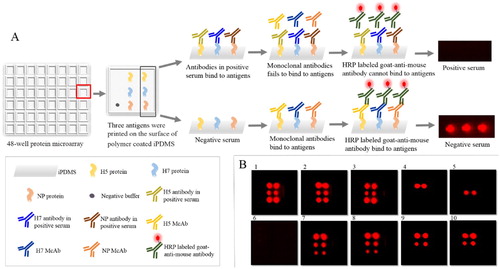
Inhibition percentage = (1 − SI of sample / SI of negative) × 100%, where the SI of sample = signal intensity of sample serum − signal intensity of negative buffer, SI of negative = signal intensity of negative control − signal intensity of negative buffer.
To determine the best antigen concentration, we diluted purified H5-HA and H7-HA proteins to 0.2, 0.1, 0.05, 0.025, 0.0125 and 0.00625 mg/ml, and the purified NP protein to 0.05, 0.025 and 0.0125 mg/ml. To optimize mAb dilution, we tested the twofold serial dilutions of H5 and H7 mAbs with peroxidase conjugate stabilizer from 1:500 to 1:4000, and NP mAb dilution ranged from 1:20,000 to 1:640,000. After the most suitable concentration and antigen and mAbs dilution were determined, serum samples with twofold serial dilutions (1:2, 1:4, 1:8, 1:16, 1:32 and 1:64) were added to the microplates to determine the optimum service concentration. The optimal conditions were determined by the relatively high inhibition percentage and then used in the following assays.
The cut-off value of the protein microarray was determined by the inhibition rates of 64 negative sera from chicken and duck. The cut-off value was calculated as follows: cut-off value = mean + 2SD (standard deviation values). A total of 64 negative samples were tested using the HI test (Pedersen, Citation2008) and AIV antibody test ELISA kit (IDEXX, Westbrook, ME, USA) according to the manufacturer’s procedure.
Protein microarray sensitivity and cross-reactivity
The limit of detection corresponds to the lowest HI titre of the serum in which the protein microarray result is positive. To determine this limit, we performed the assay with the twofold serial dilutions of HI positive serum which is a known titre from the immunized (H5 and H7) chickens.
To test the cross-reactivity of the microarray among different subtypes and other poultry viruses, we hybridized the protein microarrays with the positive sera against 13 subtypes of AIV, NDV-LaSota, IBV, IBDV and ALV-J. The AIV subtypes were H1N1, H2N2, H3N2, H4N6, H5N1, H6N5, H7N7, H8N4, H9N2, H11N9, H12N5, H13N6 and H14N5.
Reproducibility experiments
To conduct the repeatability test, we chose eight field serum samples (four positive samples and four negative samples). For intra-assay (within-plate) reproducibility, the two replicates of each serum sample were tested within the same plate. For inter-assay (between-run) reproducibility, the two replicates of each sample were detected in separate plates.
Field serum sample testing
A total of 55 chicken field serum samples were tested by the IDEXX AIV antibody test ELISA kit, protein microarray and HI test. The antibodies against H5 and H7 were identified by HI test. Afterwards, the diagnostic sensitivity and diagnostic specificity of the protein microarray were determined. HI test and microarray were also used to detect the antibody production after the immunization of 40 serum samples from duck and peacock, respectively.
Results
Recombinant protein identification
The recombinant proteins H5-HA, H7-HA and AIV-NP were expressed successfully. As shown in supplementary Figure S1(A), recombinant H5-HA was expressed at approximately 36 kD, and the H5-HA protein reacted strongly with H5 mAb in the western blot analysis (Figure S1(B)). SDS-PAGE analysis confirmed that a H7-HA protein of approximately 36 kD was expressed (Figure S2(A)). We performed western blot analysis using a H7 mAb (Figure S2(B)). Figure S3 shows that the recombinant protein AIV-NP was purified and had a good immune reaction with NP mAb.
Protein microarray optimization
The optimal purified antigen and mAb concentrations were identified. To obtain the high intensity chemiluminescence signal which is appropriate to observe and calculate the inhibition percentage, we determined 0.2 mg/ml H5 and H7 antigens and 0.05 mg/ml NP antigen to be optimal ((A–C)), and the optimal dilutions of the H5, H7 and NP mAbs were 1:2000, 1:2000 and 1:320,000 ((D–F)), respectively. The positive and negative sera were serially diluted twofold, as shown in (G–I). The best dilution ratio for the serum samples was 1:32.
Figure 2. Optimization of protein microarray working conditions. Different purified antigen concentrations (0.2, 0.1, 0.05, 0.025, 0.0125 and 0.00625 mg/ml) were immobilized for the H5 (A), H7 (B) and NP (C) antigen concentrations. The mAb concentrations were determined via a dilution assay for H5 mAb (D), H7 mAb (E) and NP mAb (F). After the antigen and antibody concentrations were determined, the optimal incubation conditions for the serum were tested. Inhibition percentage using different positive and negative serum dilutions (G–I). The vertical dotted lines indicate the selected dilution for serum incubation.
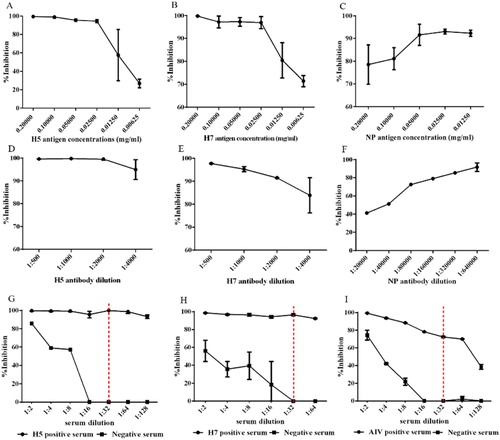
To determine the cut-off level of the inhibition percentage for the protein microarray, we examined 64 negative serum samples from chickens and ducks. The microarray results were analysed separately for each species and are presented in . Regarding the 54 chicken serum samples, according to the formula described above, the cut-off values of H5, H7 and NP were 40%, 40% and 50%, whilst those of the 10 negative duck samples were 0%, 40% and 20%. We do not own the peacock’s negative serum, and the cut-off value of H5 protein microarray of duck’s negative serum is 0%. So, a 30% inhibition (the half of the mean value of positive serum) was chosen as a positive/negative cut-off of the duck serum of H5 microarray (Figure S4). Regarding peacock serum, the cut-off values of H5, H7 and H9 protein microarray were all 50%.
Protein microarray sensitivity
H5 and H7 sera were diluted at twofold serial dilution. The diluted serum was tested for HI titre, delivered on the array and incubated with the protein microarray. The inhibition percentage changed according to the serum dilution as shown in . Using protein microarray incubated with H5 serum, the detection limit of H5 and NP were 1 HI and 0.5 HI titres respectively; and the lowest detection lines for H7 and NP were 1 HI and 0.5 HI titres when incubated with H7 serum, respectively.
Cross-reactivity with other subtypes of influenza viruses and other avian viruses
To test the specificity of the protein microarray, we also hybridized the positive sera of IBV, IBDV, NDV-LaSota, ALV-J and AIV (H1, H2, H3, H4, H5, H6, H7, H8, H9, H11, H12, H13 and H14) with the protein microarrays. The H5 antigen reacted specifically with the positive sera for H5 subtype of AIV and showed no cross-reactivity with the positive sera for IBV, IBDV, NDV-LaSota and ALV-J. Similarly, H7 antigen only reacted with the positive serum of the H7 subtype of AIV. All positive sera for AIV can bind to NP antigen, whilst IBV, IBDV, NDV-LaSota and ALV-J cannot. The inhibition percentage of the negative reaction was significantly lower than the negative threshold (). These results indicated that this protein microarray can be used to test specific antibodies against H5, H7 and NP.
Reproducibility experiments
The intra-assay and inter-assay repeatability tests were performed on four positive and four negative chicken serum samples. The results of the same sera were consistent both in intra-assay and inter-assay tests, and all these results were consistent with the HI and ELISA results. This finding suggested that the protein microarray was reproducible (Table S3).
Field serum sample testing
The field serum samples from 55 chickens were tested by IDEXX AIV antibody ELISA test kit, HI test methods and the present microarray. Compared with IDEXX AIV antibody ELISA test kit, the results showed that 55 samples were positive for antibodies against AIV; the diagnostic sensitivity and diagnostic specificity of NP antibodies in the protein microarray were both 100%. Compared with HI, the diagnostic sensitivity of H5 and H7 antibodies in the protein microarray were both 100%, whilst the diagnostic specificity of H5 antibodies was 100%, and that of H7 was 97.06% (). A total of 40 serum samples were obtained from ducks and peacocks, all of which were applied to the protein microarray and compared with the HI results. The agreement ratio of the microarray was 90% (18/20) in ducks and 100% (20/20) in peacocks (). The data demonstrated that the protein microarray in this study had good specificity and sensitivity for AIV antibody detection compared with the HI test.
Table 1. Comparison of the AIV antibody test ELISA kit, HI test and protein microarray with 55 field serum samples.
Table 2. Duck and peacock serum sample detection.
Discussion
AIV is classified according to viral surface proteins. Haemagglutinin has 18 subtypes (H1–H18), and neuraminidase has 11 subtypes (N1–N11); these haemagglutinin and neuraminidase subtypes can reassort into many combinations (Zhang et al., Citation2016). The different subtypes of AIV have been found in different species, such as chickens, ducks, dogs and pigs, thereby resulting in considerable economic losses. AIV can infect people, and public health is under serious threat. The huge harm and loss caused by H5 and H7 subtypes of highly pathogenic avian influenza to human beings lead to several public concerns. Many techniques for AIV detection have been reported, but these techniques detect a specific subtype of a species, thereby making detection inconvenient. Protein microarrays, as an emerging technology, are an effective tool for a high-throughput approach and can provide a platform for characterization of hundreds of thousands of proteins (Sutandy et al., Citation2013; Zhao et al., Citation2015). At present, protein microarrays play an important role in numerous applications, such as food safety, disease diagnosis, drug screening and environmental protection (Anderson et al., Citation2011; Berrade et al., Citation2011). However, the application of protein microarrays in poultry disease detection is rare. In the present study, a blocking protein microarray was developed to detect the serum antibodies of AIV on the basis of a new solidly supported material named iPDMS membrane. This membrane can reduce nonspecific reactions and improve sensitivity (Huang et al., Citation2015). This blocking protein microarray method can be applied to detect influenza antibodies in the serum of all birds and simultaneously distinguish H5 or H7 subtypes. Serological assays can be used to determine whether susceptible species or humans have a history of infection, thereby providing vital clues for AIV surveillance and diagnosis.
MAbs have many advantages, such as high specificity, strong sensitivity and unlimited supply. MAbs are effective tools and broadly applied in different detection methods. NP is a relatively conserved protein in AIV. Anti-NP mAbs are generally utilized in the diagnosis of all influenza virus infections (Phuong et al., Citation2018). MAbs against the HA protein of the influenza virus can often be used to distinguish its subtypes (Jensen et al., Citation2013). In this paper, multiple mAbs were experimentally screened, and three of them were specific to the HA protein of H5 and H7 subtypes, as well as to the NP protein of AIV. Three mAbs demonstrated good blocking effects on the protein microarray approach and were used to develop an inhibition protein microarray for detection of a serological response against AIV. The blocking effect of the three mAbs also indicated that the epitopes recognized by them may be the dominant epitopes of H5, H7 and NP proteins during viral infection.
The protein microarray had good specificity without cross-reaction when used to test serum samples of other influenza virus subtypes and avian diseases such as AIV, NDV-LaSota, IBV, IBDV and ALV-J. The serum with known HI titres was incubated in protein microarray, and the results demonstrated that the detection limit of the H5 and H7 antibodies were both 1 HI titres in the protein microarray. When serum HI titres were titrated based on positive H5 and H7 subtypes, the detection limits of the NP antibodies were both 0.5 HI titres. The protein microarray and HI test were compared based on serum sample detection, and the results indicated that the diagnostic sensitivity and specificity of the H5 and H7 antibodies in protein microarray reached >97% in chicken serum. The agreement ratios of the sera of duck and peacock were almost 100% compared with that of the HI. At present, H5, H7 and H9 commercial vaccines are often used to prevent influenza virus infection in China, and the detection of antibody produced after immunization is considered to be very important. The HI test is generally reserved to distinguish between AIV subtypes and to evaluate vaccine effectiveness, but it is time-consuming and labourious. Our assay can simultaneously distinguish the antibodies between different avian influenza subtypes and H5, H7 AIVs. In future research, we will use protein microarrays to differentiate additional antibodies against avian influenza subtypes and establish a link between the inhibition percentage and HI titre which increase the efficiency and convenience of influenza antibody detection.
ELISA is a common method in monitoring AIV (He et al., Citation2013). Although it can simultaneously detect multiple samples, it is normally used for the detection of only one antigen. However, the protein microarray approach can detect not only multiple samples but can also distinguish the NP antibodies and antibodies against H5 and H7 subtypes. When clinical chicken serum was tested, the agreement ratio of the protein microarray and IDEXX AIV antibody test ELISA kit was 100%. Small serum volumes were required in this method which is also a major advantage because obtaining large volumes of sera from wild waterfowl species and shorebirds is difficult. In practice, the wild birds are very important transmitters in spreading AIV from one area to another.
A protein microarray method was established for the rapid detection of IBV antibody that takes only 15 min and results can be directly observed with the naked eye (Yan et al., Citation2018). However, this method can only detect the serum antibody of a single species due to the limitations of secondary antibody. This method takes approximately 1.5 h to test 48 serum samples in the protein microarray of this article, and equipment is still needed to obtain the results. However, by taking advantage of the blocking effect of mAbs, the protein microarray method can detect three influenza antibodies (i.e. H5, H7 and NP) of different species at the same time, and it has good sensitivity and specificity. In future studies, we will further accelerate the speed and improve the convenience of the protein microarray method to make its detection fast and the results easy to observe. Meanwhile, given that our current approach allows the high-throughput detection of pathogens, we will continue to increase the number of different kinds of antigens that are jet-printed to the predetermined locations of iPDMS for the detection of different diseases on the same microarray.
Conclusions
We developed a blocking protein microarray for AIV antibody and simultaneous detection of H5 and H7 antibody subtypes. We improved the specificity and sensitivity of this method by using the iPDMS membrane with good reproducibility. The accuracy was as high as that of HI test. Using mAb application, we can test serum samples of different species without changing reagents. A specific, sensitive and relatively simple blocking protein microarray method has been developed to detect influenza virus antibodies. Therefore, our study may expand the detection range of the microarrays and offer a basis for disease diagnosis in animals or humans, and other studies.
Supplemental Material
Download Zip (1 MB)Disclosure statement
No potential conflict of interest was reported by the authors.
Additional information
Funding
References
- Anderson, K.S., Sibani, S., Wallstrom, G., Qiu, J., Mendoza, E.A., Raphael, J., Hainsworth, E., Montor, W.R., Wong, J., Park, J.G., Lokko, N., Logvinenko, T., Ramachandran, N., Godwin, A.K., Marks, J., Engstrom, P. & Labaer, J. (2011). Protein microarray signature of autoantibody biomarkers for the early detection of breast cancer. Journal of Proteome Research, 10, 85–96. doi: 10.1021/pr100686b
- Berrade, L., Garcia, A.E. & Camarero, J.A. (2011). Protein microarrays: novel developments and applications. Pharmaceutical Research, 28, 1480–1499. doi: 10.1007/s11095-010-0325-1
- Capua, I. & Alexander, D.J. (2004). Avian influenza: recent developments. Avian Pathology, 33, 393–404. doi: 10.1080/03079450410001724085
- Chappell, L., Killian, M.L. & Spackman, E. (2014). Detection of influenza A antibodies in avian serum samples by ELISA. Methods in Molecular Biology, 1161, 151–167. doi: 10.1007/978-1-4939-0758-8_14
- Claas, E.C., Osterhaus, A.D., van Beek, R., De Jong, J.C., Rimmelzwaan, G.F., Senne, D.A., Krauss, S., Shortridge, K.F. & Webster, R.G. (1998). Human influenza A H5N1 virus related to a highly pathogenic avian influenza virus. Lancet, 351, 472–477. doi: 10.1016/S0140-6736(97)11212-0
- He, F., Prabakaran, M., Tan, Y., Indira, K., Kumar, S.R. & Kwang, J. (2013). Development of dual-function ELISA for effective antigen and antibody detection against H7 avian influenza virus. BMC Microbiology, 13, 219. doi: 10.1186/1471-2180-13-219
- Hemmatzadeh, F., Sumarningsih, S., Tarigan, S., Indriani, R., Dharmayanti, N.L., Ebrahimie, E. & Igniatovic, J. (2013). Recombinant M2e protein-based ELISA: a novel and inexpensive approach for differentiating avian influenza infected chickens from vaccinated ones. PLoS One, 8, e56801. doi: 10.1371/journal.pone.0056801
- Hirst, G.K. (1942). Adsorption of influenza hemagglutinins and virus by red blood cells. Journal of Experimental Medicine, 76, 195–209. doi: 10.1084/jem.76.2.195
- Huang, M., Ma, Q., Liu, X., Li, B. & Ma, H. (2015). Initiator integrated poly(dimethysiloxane)-based microarray as a tool for revealing the relationship between nonspecific interactions and irreproducibility. Analytical Chemistry, 87, 7085–7091. doi: 10.1021/acs.analchem.5b00694
- Jarocka, U., Sawicka, R., Gora-Sochacka, A., Sirko, A., Zagorski-Ostoja, W., Radecki, J. & Radecka, H. (2014). An immunosensor based on antibody binding fragments attached to gold nanoparticles for the detection of peptides derived from avian influenza hemagglutinin H5. Sensors (Basel), 14, 15714–15728. doi: 10.3390/s140915714
- Jensen, T.H., Ajjouri, G., Handberg, K.J., Slomka, M.J., Coward, V.J., Cherbonnel, M., Jestin, V., Lind, P. & Jorgensen, P.H. (2013). An enzyme-linked immunosorbent assay for detection of avian influenza virus subtypes H5 and H7 antibodies. Acta Veterinaria Scandinavica, 55, 84. doi: 10.1186/1751-0147-55-84
- Laamiri, N., Fallgren, P., Zohari, S., Ben Ali, J., Ghram, A., Leijon, M. & Hmila, I. (2016). Accurate detection of avian respiratory viruses by use of multiplex PCR-based Luminex Suspension microarray assay. Journal of Clinical Microbiology, 54, 2716–2725. doi: 10.1128/JCM.00610-16
- Letant, S.E., Ortiz, J.I., Bentley Tammero, L.F., Birch, J.M., Derlet, R.W., Cohen, S., Manning, D. & McBride, M.T. (2007). Multiplexed reverse transcriptase PCR assay for identification of viral respiratory pathogens at the point of care. Journal of Clinical Microbiology, 45, 3498–3505. doi: 10.1128/JCM.01712-07
- Li, X.L., Yang, Y., Sun, Y., Chen, W.J., Sun, R.X., Liu, K., Ma, M.J., Liang, S., Yao, H.W., Gray, G.C., Fang, L.Q. & Cao, W.C. (2015). Risk distribution of human infections with avian influenza H7N9 and H5N1 virus in China. Scientific Reports, 5, 18610. doi: 10.1038/srep18610
- Liu, J., Yao, L., Zhai, F., Chen, Y., Lei, J., Bi, Z., Hu, J., Xiao, Q., Song, S., Yan, L. & Zhou, J. (2018). Development and application of a triplex real-time PCR assay for the simultaneous detection of avian influenza virus subtype H5, H7 and H9. Journal of Virological Methods, 252, 49–56. doi: 10.1016/j.jviromet.2017.11.005
- Ma, H., Wu, Y., Yang, X., Liu, X., He, J., Fu, L., Wang, J., Xu, H., Shi, Y. & Zhong, R. (2010). Integrated poly(dimethysiloxane) with an intrinsic nonfouling property approaching “absolute” zero background in immunoassays. Analytical Chemistry, 82, 6338–6342. doi: 10.1021/ac101277e
- Pang, Y., Rong, Z., Wang, J., Xiao, R. & Wang, S. (2015). A fluorescent aptasensor for H5N1 influenza virus detection based-on the core-shell nanoparticles metal-enhanced fluorescence (MEF). Biosensors and Bioelectronics, 66, 527–532. doi: 10.1016/j.bios.2014.10.052
- Pedersen, J.C. (2008). Hemagglutination-inhibition test for avian influenza virus subtype identification and the detection and quantitation of serum antibodies to the avian influenza virus. Methods in Molecular Biology, 436, 53–66.
- Phuong, N.H., Kwak, C., Heo, C.K., Cho, E.W., Yang, J. & Poo, H. (2018). Development and characterization of monoclonal antibodies against nucleoprotein for diagnosis of influenza A virus. Journal of Microbiology and Biotechnology, 28, 809–815. doi: 10.4014/jmb.1801.01002
- Stone, B., Burrows, J., Schepetiuk, S., Higgins, G., Hampson, A., Shaw, R. & Kok, T. (2004). Rapid detection and simultaneous subtype differentiation of influenza A viruses by real time PCR. Journal of Virological Methods, 117, 103–112. doi: 10.1016/j.jviromet.2003.12.005
- Sutandy, F.X., Qian, J., Chen, C.S. & Zhu, H. (2013). Overview of protein microarrays. Current Protocols in Protein Science, Chapter 27, Unit 27.1.
- Van Kerkhove, M.D. (2013). Brief literature review for the WHO global influenza research agenda–highly pathogenic avian influenza H5N1 risk in humans. Influenza and Other Respiratory Viruses, 7, 26–33. doi: 10.1111/irv.12077
- Wade, A., Taiga, T., Fouda, M.A., MaiMoussa, A., Jean Marc, F.K., Njouom, R., Vernet, M.A., Djonwe, G., Mballa, E., Kazi, J.P., Salla, A., Nenkam, R., Poueme Namegni, R., Bamanga, H., Casimir, N.K.M., LeBreton, M., Nwobegahay, J.M., Fusaro, A., Zecchin, B., Milani, A., Gaston, M., Chepnda, V.R., Dickmu Jumbo, S., Souley, A., Aboubakar, Y., Fotso Kamnga, Z., Nkuo, C., Atkam, H., Dauphin, G., Wiersma, L., Bebay, C., Nzietchueng, S., Vincent, T., Biaou, C., Mbacham, W., Monne, I. & Cattoli, G. (2018). Highly pathogenic avian influenza A/H5N1 clade 2.3.2.1c virus in poultry in Cameroon, 2016–2017. Avian Pathology, 47, 559–575. doi: 10.1080/03079457.2018.1492087
- Wang, X., Jiang, H., Wu, P., Uyeki, T.M., Feng, L., Lai, S., Wang, L., Huo, X., Xu, K., Chen, E., Wang, X., He, J., Kang, M., Zhang, R., Zhang, J., Wu, J., Hu, S., Zhang, H., Liu, X., Fu, W., Ou, J., Wu, S., Qin, Y., Zhang, Z., Shi, Y., Zhang, J., Artois, J., Fang, V.J., Zhu, H., Guan, Y., Gilbert, M., Horby, P.W., Leung, G.M., Gao, G.F., Cowling, B.J. & Yu, H. (2017). Epidemiology of avian influenza A H7N9 virus in human beings across five epidemics in mainland China, 2013–17: an epidemiological study of laboratory-confirmed case series. The Lancet Infectious Diseases, 17, 822–832. doi: 10.1016/S1473-3099(17)30323-7
- Wang, X., Shi, L., Tao, Q., Bao, H., Wu, J., Cai, D., Wang, F., Zhao, Y., Tian, G., Li, Y., Qao, C. & Chen, H. (2010). A protein chip designed to differentiate visually antibodies in chickens which were infected by four different viruses. Journal of Virological Methods, 167, 119–124. doi: 10.1016/j.jviromet.2010.03.021
- Yan, L., Hu, J., Lei, J., Shi, Z., Xiao, Q., Bi, Z., Yao, L., Li, Y., Chen, Y., Fang, A., Li, H., Song, S., Liao, M. & Zhou, J. (2018). Novel protein chip for the detection of antibodies against infectious bronchitis virus. BMC Veterinary Research, 14, 284. doi: 10.1186/s12917-018-1586-x
- Zhang, R., Chen, T., Ou, X., Liu, R., Yang, Y., Ye, W., Chen, J., Yao, D., Sun, B., Zhang, X., Zhou, J., Sun, Y., Chen, F. & Wang, S.-P. (2016). Clinical, epidemiological and virological characteristics of the first detected human case of avian influenza A(H5N6) virus. Infection, Genetics and Evolution, 40, 236–242. doi: 10.1016/j.meegid.2016.03.010
- Zhao, Y., Wang, X., Chen, P., Zeng, X., Bao, H., Wang, Y., Xu, X., Jiang, Y., Chen, H. & Li, G. (2015). Detection of antibodies against avian influenza virus by protein microarray using nucleoprotein expressed in insect cells. Journal of Veterinary Medical Science, 77, 413–419. doi: 10.1292/jvms.14-0207