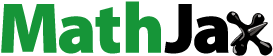
ABSTRACT
Fowl cholera is a highly contagious disease within the global duck farming industry. This study aimed at formulating and evaluating the protective efficacy of a combination vaccine containing a recombinant outer membrane protein H (rOmpH) of Pasteurella multocida strain X-73 with a live attenuated duck plague vaccine into a single dose. Four groups of ducks received different treatments and the groups were labelled as non-vaccinated, combined vaccination, duck plague vaccination and rOmpH vaccination, respectively. The combined vaccination group was comprised of live attenuated duck plague commercial vaccine with 100 µg rOmpH to a total volume of 0.5 ml/duck/intramuscular administration. All groups were challenged with avian P. multocida strain X-73 via intranasal administration. In addition, blood samples were collected monthly over a period of 6 months to determine the appropriate antibody level by indirect ELISA. The indirect ELISA results in the combination vaccine group revealed that the average levels of the serum antibody against the duck enteritis virus (0.477 ± 0.155) and fowl cholera (0.383 ± 0.100) were significantly higher than those values in the non-vaccinated control group (0.080 ± 0.027 and 0.052 ± 0.017), respectively (P < 0.05). Moreover, all vaccinated ducks were effectively protected from fowl cholera. This preliminary study indicated that a combination vaccine did not affect the antibody response in the subjects while protecting the ducks against experimental P. multocida infection. This combination vaccine should be considered part of an alternative pre-treatment strategy that could replace the monovalent vaccine.
Introduction
Bacterial and viral infectious diseases can lead to massive outbreaks in the poultry industry worldwide. In Thailand, the number of ducks increased continually from 22.7 to 29.5 million in the period from 2008 to 2018 (http://certify.dld.go.th/certify/index.php). These ducks were kept by large commercial farmers for the purposes of both meat and egg production, while numerous smaller farmers and owners of private households are also known to raise ducks on their properties and in their backyards. In accordance with the economic data that has been obtained from the Department of Livestock Development, it has been revealed that in 2017 the export value of ducks and their commercial products reached $0.2 billion US (http://certify.dld.go.th/certify/index.php). Importantly, the prevention of both infectious diseases and issues related to animal welfare have become significant concerns because the raising of ducks on an industrial scale is certainly a major component of the overall poultry industry.
Fowl cholera (avian pasteurellosis), one of the most important bacterial infectious diseases occurring in ducks, is caused by the bacterium Pasteurella multocida. It is considered a highly contagious disease that affects a range of avian species (Samuel et al., Citation2007). The disease can be divided into acute septicaemia and chronic forms, and can also occur as localized infections that are associated with high morbidity and mortality rates of up to 100% resulting in potentially huge economic losses (Pedersen et al., Citation2003; Samuel et al., Citation2007). The outer membrane protein H (OmpH), a major membrane protein found in the envelope and capsule of P. multocida, has displayed potential as a protective antigen with strong immunogenicity (Chevalier et al., Citation1993; Sthitmatee et al., Citation2011; Sthitmatee et al., Citation2013; Varinrak et al., Citation2019). It has been incorporated into a fowl cholera vaccine and may provide protective immunity against P. multocida in chickens and ducks (Sthitmatee et al., Citation2008; Thanasarasakulpong et al., Citation2015; Varinrak et al., Citation2017; Poolperm et al., Citation2018). Early studies have successfully formulated an intranasal vaccine by inducing the homologous serum immunoglobulin (Ig) Y and secretory IgA against avian P. multocida strain infection in vivo. The vaccine appeared to elicit good protection in chickens (Thanasarasakulpong et al., Citation2015). Moreover, the previous development of an intranasal fowl cholera vaccine against rOmpH of P. multocida in ducks was also found to induce an efficient antibody that could reduce adhesion to duck embryo fibroblast cells and could successfully induce high levels of lymphocyte proliferation. This vaccine also induced homologous serum IgY and secretory IgA (Poolperm et al., Citation2018).
One of the important infectious viral diseases found amongst ducks is the duck virus enteritis which is also known as duck plague. It is an acute contagious herpesvirus disease that occurs among Anseriformes (ducks, geese and swans) and is caused by the duck enteritis virus (DEV). Lethal DEV infection can cause 100% mortality in ducks (Dhama et al., Citation2017). Duck plague has resulted in significant economic losses in industries that are involved in the raising of domestic and wild waterfowl due to high mortality rates, condemnations of carcasses and decreased egg production levels in duck-producing countries such as Thailand (Leibovitz & Hwang, Citation1968; Kathryn et al., Citation2001; Dhama et al., Citation2017).
The administration of vaccinations is considered an efficient strategy to control diseases. Vaccines that have recently been developed for both duck plague and fowl cholera are classified as monovalent vaccines that require multiple immunizations. In general, there are two types of fowl cholera vaccines; the broth bacterin without adjuvant, and the bacterin containing aluminium hydroxide or oil that can serve as an adjuvant before being used (OIE, Citation2015). Specifically, birds are injected with the antigens that have been incorporated in a classic water-in-mineral oil emulsion as an adjuvant that is often associated with localized side-effects. These side-effects have been shown to induce inflammatory lesions such as granuloma and sterile abscess formation (Belloc et al., Citation2008). DEV attenuated live vaccine has been developed and has shown protective immunity against duck viral enteritis (Jansen et al., Citation1963; Huang et al., Citation2014). In addition, the recombinant DEV-vectored bivalent live vaccine displayed protection against the lethal H5N1 avian influenza virus. These features make DEV suitable for use as a bivalent live attenuated vaccine in ducks (Liu et al., Citation2011). It has become increasingly more challenging to formulate these combination vaccines without employing an oil adjuvant into the schedule (Skibinski et al., Citation2011). Consequently, combining multivalent vaccines into a single dose is considered an alternative strategy that could be used to replace monovalent vaccines. The administration of a combination vaccine would not only reduce the number of immunizations needed in birds but would also decrease the intensity of the localized side-effects that occur from the vaccine formulations by the fact that the amount of adjuvant would also be reduced.
Thus, the present study is aimed at formulating and evaluating the protective efficacy of a recombinant vaccine using the outer membrane protein H (rOmpH) of P. multocida strain X-73 combined with a live attenuated DEV vaccine as a bivalent vaccine against lethal P. multocida challenge. Moreover, the antibody responses against fowl cholera or duck plague vaccines were also determined by an in-house indirect ELISA.
Materials and methods
Birds
In this study, forty 8-week-old Khaki Campbell ducks (Anas platyrhynchos: APA Farm Co. Ltd., Chiang Mai, Thailand) were divided into four groups according to the four types of vaccines they were given (n = 10 ducks each group). The groups were labelled as the non-vaccinated control group (group 1), combined vaccination group containing rOmpH mixed with live attenuated duck plague vaccination (group 2), live attenuated duck plague vaccination group (group 3) and rOmpH vaccination group (group 4). The rOmpH vaccine used in group 4 was administered with Montanide adjuvant (SEPPIC, Paris, France).
The project underwent an ethical review and was given approval by an institutional animal care and use committee. The Animal Welfare Committee of the Faculty of Veterinary Medicine, Chiang Mai University oversaw the use of laboratory birds in accordance with laboratory animal ethics protocols (license number R15/2558). All experiments followed the guidelines that had been designated by the Guide for the Care and Use of Agricultural Animals in Research and Teaching (the Ag Guide, FASS 2010).
Recombinant OmpH
Notably, rOmpH was expressed and purified by the method described in the previous study (Sthitmatee et al., Citation2008; Poolperm et al., Citation2018). E. coli strain PQE-ompH was grown aerobically at 37°C in Luria–Bertani (LB; Difco Laboratories) broth or on LB agar that had been supplemented with 100 µg/ml ampicillin and 25 µg/ml kanamycin (Sigma Aldrich, St. Louis, MO, USA). E. coli strain PQE-ompH glycerol stock was streaked on LB agar containing 100 µg/ml ampicillin and 25 µg/ml kanamycin and incubated at 37°C for 18 h. After the incubation period, a single colony was chosen and inoculated into 20 ml LB broth containing 100 µg/ml ampicillin and 25 µg/ml kanamycin. The culture was grown at 37°C for 18 h with horizontal shaking at 210 rpm. One litre of the LB broth containing 100 µg/ml ampicillin and 25 µg/ml kanamycin was inoculated at a ratio of 1:50 with overnight culturing and allowed to continue growing under the same conditions until an optical density (OD)600 of 0.5–0.7 (mid-log phase) was reached. The recombinant protein expression was subsequently induced by the addition of isopropyl-β-D-thiogalactopyranoside (IPTG; Amresco, Solon, OH, USA) to a final concentration of 1 mM. The culture was then incubated for an additional 4–5 h. Finally, the cells were harvested by centrifugation at 4000 × g for 20 min at 4°C and kept at −20°C for purification.
The purification process of the recombinant protein in this study was conducted by an electroelution method that has been previously described (Thanasarasakulpong et al., Citation2016). Briefly, the cell pellets were lysed in native lysis buffer (100 mM NaH2PO4, 10 mM Tris-HCl, 8 M urea; pH 8.0) with gentle shaking at 4°C for 1 h. The suspension was centrifuged at 10,000 × g at 4°C for 30 min. The supernatant was collected and placed into the chamber of an electroelutor (Nativen; ATTO, Tokyo, Japan). Approximately 1500 µl of the total protein solution was run on a preparative 12.5% sodium dodecyl sulfate polyacrylamide gel column (10 mm stacking gel and 30 mm separating gel) in the sample buffer (4% sodium dodecyl sulfate, 50 mM Tris, 20% glycerol, 0.005% Bromophenol blue). The conditions for protein collection were determined according to the manufacturer’s instructions (delay time: 320 min; eluting time: 6 min; filling time: 100 s; collecting time: 120 s; 15 mA). The rOmpH fractions were obtained from the collection buffer (371 mM Tris, 5% sucrose; pH 8.8) and kept at −20°C for further analysis. The rOmpH concentration was measured using the BCA protein assay kit (Pierce®, Rockford, IL, USA) according to the manufacturer’s instructions.
The eluted rOmpH was passed through a detergent-removing minicolumn (Ampure DT, Amersham Biosciences, Little Chalfont, UK) in order to remove sodium dodecyl sulphate from the protein solution. The rOmpH was adjusted to 100 μg of protein/0.5 ml of Duck Plague Vaccine (see the next section).
Duck plague vaccine
The Duck Plague VaccineTM (Jansen strain) used in this study was manufactured by the Bureau of Veterinary Biologics, Department of Livestock Development, Ministry of Agriculture and Cooperative, Thailand. One dose of the vaccine (0.5 ml) contained 103 TCID50 (median Tissue Culture Infectious Dose) of DEV Jansen strain.
Immunization
The ducks were divided into four groups based on the vaccine formulations they were assigned. The non-vaccinated group (group 1) was used as a control. Additionally, the combination rOmpH plus the live attenuated DEV (group 2), the live attenuated DEV (group 3) and the rOmpH – 100 µg (group 4) vaccines were administered intramuscularly twice with a one-month interval. All ducks were then observed for clinical signs and behavioural changes that may have occurred at the pre- and post-immunization stages.
Challenge exposure
Both the vaccinated and non-vaccinated control ducks were challenge-exposed with virulent P. multocida strain X-73 isolate 3.5 × 103 CFU/ml via intranasal administration at 4 weeks after the final vaccination according to the protocol described in the previous study (Poolperm et al., Citation2018). All ducks were observed for clinical signs and mortality until termination of the study at 7 days post challenge. Necropsies were performed on all ducks that died from experimental infections. Gross lesions were recorded, while lungs, livers, spleens, kidneys and hock joints were collected for bacterial isolation. Moreover, nasal discharges and rectal swabs were also collected from the surviving ducks in each group. The bacterial isolation was performed by direct culture using DSA plates (Difco), and each species was identified by biochemical tests as has been previously described (Karaivanov, Citation1984; Marru et al., Citation2013).
ELISA test to evaluate immune response to P. multocida strain X-73
P. multocida serology was performed using ELISA (Poolperm et al., Citation2017). Briefly, 96-well plates were coated with 100 µl of 0.3% formalinized whole cells of P. multocida strain X-73 (approximately 104 CFU/well) or 1 µg of rOmpH. The specimens were then incubated overnight at 4°C. After being washed three times with a washing buffer (PBS containing 0.05% Tween: PBST), the plates were blocked with 1% bovine serum albumin (BSA)-PBS for 1 h at 37°C. The plates were then washed again, and 100 µl of 1:100 dilution of duck sera or 1:10 dilution of tracheal lavage was added to the specimens in duplicate. All wells were incubated for 1 h at 37°C. Horseradish peroxidase (HRP)-conjugated rabbit anti-duck IgG antibody (Sigma-Aldrich) was used as the secondary antibody at 1:4000 or 1:1000 ratios of dilution, respectively. Specimens were then incubated for 1 h at 37°C. After being washed three times, 100 µl tetramethylbenzidine (TMB; KPL) was added to each specimen. After incubation for 15 min, the reaction was terminated by the addition of 50 µl 2 N H2SO4. Optical density was measured in each well at 450 nm (OD450). The standard error of the mean value of each group was calculated according to the method described in the previous report (Poolperm et al., Citation2018).
ELISA to evaluate immune response to duck plague
Indirect ELISA procedure
The indirect ELISA test for antibody against DEV was performed in 96-well microtitre plates (Nunc-Immuno™ MaxiSorp™; Sigma-Aldrich). Each well was coated with 100 µl at 1:10,000 dilution of 103 TCID50 of inactivated DEV Jansen strain, followed by incubation at 4°C overnight. After being washed three times with PBST washing buffer (0.05% Tween 20 in 0.01 M PBS, pH 7.4), 100 μl of blocking buffer (1% BSA-PBS) was added to each well and the plates were kept for 1 h at 37°C. Subsequently, after being washed three times with PBST, 100 µl of 1:800 dilution of duck sera was added to each well in duplicate, and plates were incubated for 1 h at 37°C. After washing the plates three times with PBST, HRP-conjugated rabbit anti-duck IgG (Sigma-Aldrich) was used as the secondary antibody at a 1:20,000 ratio, and the specimens were incubated for 1 h at 37°C. Finally, after being washed an additional three times, 100 μl TMB diluted in substrate buffer was added to each well. This step was followed by a period of incubation in the dark for 30 min at 37°C. Subsequently, the colour reaction was stopped by adding 50 μl of 2 N H2SO4 following a period of incubation in the dark for 20 min at 37°C. The absorbance of each well was read at a wavelength of 450 nm using an automatic ELISA plate reader (AccuReader; Metertech, Taipei, Taiwan R.O.C.). The results were expressed as OD.
Optimization of the indirect ELISA method
The indirect ELISA conditions were optimized using a checkerboard titration involving serial dilutions of plate-coating antigen, duck plague-positive duck sera and duck plague-negative duck sera (Crowther, Citation2001). The 103 TCID50 of DEV Jansen strain was used to coat the wells at dilutions of 1:10–1:10,000 by 10-fold dilution. Additionally, the duck sera were diluted with a blocking buffer at dilutions of 1:100–1:3200. HRP-conjugated antibody was used at dilutions of 1:10,000–1:35,000. This checkerboard titration procedure was completed using sera obtained from ducks that had been immunized with duck plague vaccine and these were identified as positive samples, while the non-vaccinated duck sera were considered to be negative samples. The optimal serum dilution with 103 TCID50 of the DEV concentration was defined as the ratio that produced the greatest yield when contrasting the positive and negative sample ODs.
Calculation of the cut-off value
The ELISA cut-off value was obtained by determining the OD at a wavelength of 450 nm, and the value was calculated following the formula previously described (Kich et al., Citation2007).In the interpretation segment, an OD value of the duck sera that was higher than the cut-off value was classified as seropositive and vice versa. This means that the OD value of the lesser cut-off value was also classified as seronegative.
Statistical analyses
Antibody levels were analysed using the R statistical software programme version 3.2.2 (Pinheiro et al., Citation2018) in order to determine the mean detection of the antibody level that exceeded the cut-off value in each month. The antibody level of each bird was correlated, and the data were collected monthly. The generalized least square method was used to test the effect of the vaccine on the antibody level from day 30 to day 180.
In terms of the post hoc analysis, linear contrasts were constructed to analyse the differences in mean values between the vaccine groups at specific time points (days 30, 60, 90, 120, 150 and 180) using Tukey’s test. The significance level was set at α = 0.05.
Results
Immunization and challenge-exposure
Bird experiments were conducted to evaluate the efficacy of the combination vaccine or the monovalent vaccine against a virulent P. multocida strain X-73 challenge-exposure. Clinical signs and behavioural changes were not found at pre-immunization stages in any ducks. All the ducks in groups 1 and 3, the non-vaccinated group and the live attenuated DEV group (n = 10 ducks each group), displayed clinical signs i.e. depression, anorexia, severe diarrhoea and loss of appetite at 6–8 h after being challenged by the intranasal route. One hundred percent mortality rate (20/20) in ducks challenged with P. multocida strain X-73 was reported in both groups within 24 h. Necropsies were performed on all terminated ducks and traditional lesions of fowl cholera were observed in the carcasses of ducks, such as multiple necrotic foci in the liver and/or spleen, splenomegaly, lung congestion and oedema, multiple petechial haemorrhages in the liver and gastrointestinal tract, and fibrinopurulent peritonitis in all carcasses. Notably, no P. multocida colony growth was observed on the agar medium culture obtained from the nasal discharges or rectal swabs of the surviving ducks that displayed no clinical signs. Conversely, all ducks in groups 2 and 4 (n = 10 ducks each group) that received a combination vaccine and fowl cholera vaccine, respectively, showed no clinical signs and no mortality after challenge-exposure.
Immune response to fowl cholera
Serum antibody levels were detected using indirect ELISA test. Mean antibody levels against P. multocida strain X-73 were not different among ducks in group 2 and group 4. Additionally, the levels obtained from both groups were higher than the cut-off level (). However, the levels of serum antibody obtained from groups 2 and 4 were significantly higher than those from groups 1 and 3 (P < 0.05). In addition, the pre- and post-vaccination antibody levels of group 3 and the control group (group 1) were not statistically different and the mean levels obtained from both groups were lower than the cut-off level throughout the experiment.
Figure 1. Alterations in serum antibody levels (measured as optical density by indirect ELISA) against P. multocida strain X-73 in pre- and post-vaccinated ducks monthly until 6 months. Data are expressed as mean ± SE and compared with the OD cut-off for fowl cholera (0.200). Ducks were immunized with combination vaccine (group 2; ▴), duck plague vaccine (group 3; ▪), rOmpH vaccine (group 4; +) or were non-immunized (group 1; •).
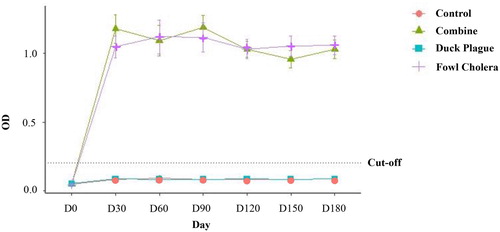
Immune response to duck plague
Standardization of indirect ELISA
The optimization of the ELISA test showed that the signal to noise (S/N) ratio of the positive and negative sample ODs at 450 nm indicated that the 103 TCID50 of the inactivated DEV Jansen strain at a dilution of 1:10,000 was suitable for plate-coating. A dilution of 1:800 was confirmed to be the optimum dilution of duck sera. At this dilution, discrimination between positive and negative sera appeared to be reasonable. Moreover, HRP-conjugated antibody was selected at a dilution of 1:20,000. The cut-off value for the mean OD of the negative control sera plus three standard deviations for DEV Jansen strain was 0.118. The averages of all duplicate means obtained for the positive and negative control sera were recorded at 0.262 and 0.092, respectively, enabling the researchers to effectively define the standard OD values.
Antibody response to DEV
As shown in , the average antibody levels of the ducks in group 2 and group 3 against DEV revealed an increasing post-immunization trend, while the serum antibody levels of those groups were significantly higher than the cut-off level and those of groups 1 and 4 (P < 0.05). However, there were no statistically significant differences found in the antibody levels between the ducks in group 2 and group 3 throughout the course of this study. In addition, the mean levels obtained from both groups were lower than the cut-off level throughout the entire experiment.
Figure 2. Alterations in serum antibody levels (measured as optical density by indirect ELISA) were monitored against DEV in pre- and post-vaccinated ducks monthly until 6 months. Data are expressed as mean ± SE and compared with the OD cut-off for duck plague (0.118). Ducks were immunized with the combined vaccine (group 2; ▴), duck plague vaccine (group 3; ▪), rOmpH vaccine (group 4; +) and non-immunized ducks were also considered (group 1; •).
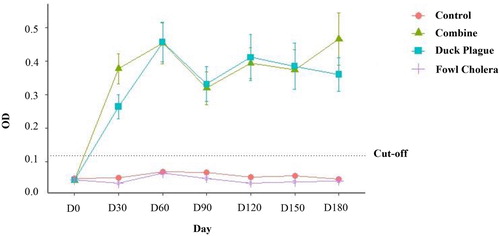
Discussion
Vaccination is one of the most effective tools for preventing diseases and reducing the economic losses of poultry producers (Sharif & Ahmad, Citation2018). Multiple vaccinations with a monovalent vaccine have several disadvantages, including the cost, and the fact that they are time-consuming and can lead to stress that ultimately affects egg or meat production. To save labour, time and costs, and to reduce potential stress levels, some owners of poultry farms have mixed commercial vaccines with partial doses or a single dose, but the vaccination procedures are not uniformly efficacious and have been labelled as “vaccine failures” (Matheis et al., Citation2001; Wu et al., Citation2017). For this reason, we intend to develop a form of vaccination that would minimize the volume of vaccines needed during the process and reduce the amount of time required for administration of the vaccination, which will have minimal effects on egg production among ducks. The present study successfully developed a bivalent vaccine in ducks and demonstrated the protection of ducks against P. multocida challenge. An in-house indirect ELISA for detecting antibody against P. multocida was developed from the previous study (Poolperm et al., Citation2017). This study provided strong support for use of the indirect ELISA to determine the antibody level to P. multocida.
The outer membrane protein H (OmpH) is a major outer membrane porin that forms a homotrimeric channel in the envelope and capsule of P. multocida. Previous studies on the OMPs of P. multocida identified them as highly protective proteins that were obtained from the crude capsular extract of P. multocida serovars A:1 and A:3. It is believed that this protein could act as an adhesive protein that can induce cross-protective immunity in capsular serotype A strains for chickens by employing the challenge-exposure procedure with the parental strain P-1059 or the heterologous strain X-73 (Chevalier et al., Citation1993; Sthitmatee et al., Citation2008). Moreover, it was suggested that this protein could serve as a candidate vaccine for fowl cholera (Luo et al., Citation1997; Borrathybay et al., Citation2008; Sthitmatee et al., Citation2008). Early studies have successfully administered the intranasal fowl cholera vaccine against rOmpH of P. multocida in ducks to induce an efficient antibody response, and to reduce the degree of adhesion of the P. multocida strain to the duck embryo fibroblast cells, along with successfully inducing high levels of lymphocyte proliferation and producing homologous serum IgY and secretory IgA at the post-vaccination stage (Poolperm et al., Citation2018). Up until now, there have not been any reports on achieving protection by rOmpH proteins, that had been isolated from P. multocida resulting in fowl cholera, when combined with duck plague vaccine to produce a multivalent vaccine that can be administered in a single dose. If successful, this could be considered an alternative strategy that could allow researchers and veterinarians to replace the monovalent vaccine. Additionally, this new vaccine could be administered in the field as a suitable alternative to the vaccines presently being used.
Previous preliminary studies compared the level of immunity in ducks that had been immunized with two vaccine types: combined and single vaccines of fowl cholera and duck plague prepared by the Bureau of Biologics, Department of Livestock Development, Ministry of Agriculture and Cooperative, Thailand (Pongsopida et al., Citation1987). The tube agglutination test and neutralization test were performed in those previous studies. The results demonstrated that the highest antibody levels among ducks vaccinated with fowl cholera monovalent vaccine were detected at one week after the first vaccination using either single or combined vaccines. Meanwhile, the antibody levels against duck plague were the highest after one week following the second vaccination. However, the antibody levels obtained from the monovalent vaccinated ducks were higher than those of the other groups. Complete protection (100% survivors) of ducks against the challenge in experimental fowl cholera and duck plague was conferred by the administration of either single or combined vaccines. This evidence contributed to the selection of an appropriate formulation and is an important step in boosting antigen immunogenicity through the use of a vaccine.
Several indirect ELISA methods have been described for the serological detection of the antibody against DEV (Wu et al., Citation2011). The indirect ELISA test for DEV antibody detection is commercially available (Qi et al., Citation2007; Wen et al., Citation2010; Wu et al., Citation2011). In the present study, the in-house indirect ELISA test employed the whole viral particle as a coating antigen. Each dose of the live Jansen strain against duck plague disease contained at least 103 EID50 of DEV and was used in the tests. It was found to provide suitable conditions for the determination of the antibody against DEV. However, the viral neutralization test has been recognized as a suitable serological test to determine the presence of an antibody against DEV. The present study has identified an alternative serological test for DEV antibody detection. However, virus neutralization tests should have been run in conjunction with the ELISA to establish a procedure of correlation with protection.
According to and , the immune response of the vaccinated ducks to the combination rOmpH-duck plague vaccine revealed that the mean values of the particular duck plague and fowl cholera antibodies were higher than the cut-off level after the first month, and then stabilized at 6 months post vaccination as had been estimated by the indirect ELISA test. These results are in agreement with those of the previous report by Hanan et al. (Citation2014) who revealed that there was no antagonizing effect amongst the increasing antibody titres of the combined vaccine against salmonellosis, duck plague and duck viral hepatitis in ducks. This outcome was also supported by the findings of Nawathe & Ayoola (Citation1981) and Mervat et al. (Citation1999) who mentioned that viral vaccines in poultry did not interfere with the immune response of the birds to bacterial vaccines if both were given in a combined form (Nawathe & Ayoola, Citation1981; Hanan et al., Citation2014). The detection of an antibody by ELISA is not necessarily indicative of protection, but perhaps only exposure. Challenge studies are of paramount importance in establishing the efficacy of any vaccine. Similarly, a previous study reported that ducks that received the duck plague-fowl cholera combined vaccine were completely protected against both infections at the seventh and eighth weeks after challenge with P. multocida and duck plague virus, respectively, after the first vaccination (Pongsopida et al., Citation1987). With regard to the present study, the results of the P. multocida exposure-challenge experiment in the fourth week after the first vaccination revealed that the rOmpH-duck plague combination vaccine provided 100% protection.
This present study utilized a preliminary experiment in which ducks were immunized with a live attenuated duck plague vaccine including rOmpH in order to determine the degree of protection that could be achieved against fowl cholera. The formulation used in this study was designed to prove our hypothesis that a combination vaccine could be obtained by excluding a vaccine adjuvant. Subsequently, the further development of a multivalent vaccine requires knowledge of the correlation with immune protection to improve vaccination efficacy against foreign pathogens (Aw-Yong et al., Citation2019). In this present study, no counteracting effects were observed regarding the duck immune response against duck plague-fowl cholera in single synchronized vaccine injections, which would also be of interest in further examinations. Likewise, the vaccine protection against DEV warrants further investigation. It could, therefore, be concluded that a combination vaccine, containing rOmpH and a live attenuated duck plague vaccine, was capable of effectively protecting ducks against artificial P. multocida infection. This finding provides researchers with important information for the future development of a new alternative series of vaccines that can improve upon the efficacy and safety of the vaccines that are presently being used. This can be achieved by presenting recombinant viral vectors that can elicit potent humoral and cellular immune responses.
Disclosure statement
No potential conflict of interest was reported by the authors.
ORCID
Nattawooti Sthitmatee http://orcid.org/0000-0002-2329-8802
Additional information
Funding
References
- Aw-Yong, K.L., NikNadia, N.M.N., Tan, C.W., Sam, I.C. & Chan, Y.F. (2019). Immune responses against enterovirus A71 infection: implications for vaccine success. Reviews in Medical Virology, 29, e2073. doi: 10.1002/rmv.2073
- Belloc, C., Dupuis, L., Deville, S., Aucouturier, J. & Laval, A. (2008). Evaluation of safety and immune response induced by several adjuvants included in Pasteurella multocida vaccines in chickens. Revue de Médecine Vétérinaire, 159, 371–375.
- Borrathybay, E., Sthitmatee, N., Suzuki, K., Shinnakasu, R., Tsuchida, S., Akuzawa, R., Kataoka, Y., Goto, K. & Sawada, T. (2008). Molecular characterization of an adhesive protein of Pasteurella multocida strain P-1059 and its variant strain P-1059B. Bulletin of the Nippon Veterinary and Life Science University, 57, 90–99.
- Chevalier, G., Duclohier, H., Thomas, D., Shechter, E. & Wroblewski, H. (1993). Purification and characterization of protein H, the major porin of Pasteurella multocida. Journal of Bacteriology, 175, 266–276. doi: 10.1128/JB.175.1.266-276.1993
- Crowther, J.R. (2001). The ELISA guidebook. New Jersey: Human Press Inc.
- Dhama, K., Kumar, N., Saminathan, M., Tiwari, R., Karthik, K., Kumar, M.A., Palanivelu, M., Shabbir, M.Z., Malik, Y.S. & Singh, R.K. (2017). Duck virus enteritis (duck plague) – a comprehensive update. Veterinary Quarterly, 37, 57–80. doi: 10.1080/01652176.2017.1298885
- Hanan, A., Ghada, M., Sadek, E., Afan, E.G. Nahed, I.M., Ekram, S.M. & Norhan, N.M. (2014). Preparation and evaluation of combined inactivated duck vaccine against salmonellosis, duck plague and duck hepatitis. Nature and Science, 12, 142–147.
- Huang, J., Jia, R., Wang, M., Shu, B., Yu, X., Zhu, D., Yin, Z., Chen, X. & Cheng, A. (2014). An attenuated duck plague virus (DPV) vaccine induces both systemic and mucosal immune responses to protect ducks against virulent DPV infection. Clinical and Vaccine Immunology, 21, 457–462. doi: 10.1128/CVI.00605-13
- Jansen, J., Kurst, H. & Wemmenhove, R. (1963). The active immunization of duck plaque. Tijdschr. Diergeneskd, 88, 927–932.
- Karaivanov, L. (1984). Biochemical tests for identifying Pasteurella multocida. Veterinarno-Meditsinski Nauki, 21, 38–44.
- Kathryn, A., Converse, A. & Gregory, A.K. (2001). Duck plague epizootics in the United States. Journal of Wildlife Diseases, 37, 347–357. doi: 10.7589/0090-3558-37.2.347
- Kich, J.D., Schwarz, P., Silva, L.E., Coldebella, A., Piffer, I.A., Vizzoto, R. & Marisa Ribeiro de, I.C. (2007). Development and application of an enzyme-linked immunosorbent assay to detect antibodies against prevalent Salmonella serovars in swine in southern Brazil. Journal of Veterinary Diagnostic Investigation, 19, 510–517. doi: 10.1177/104063870701900508
- Leibovitz, L. & Hwang, J. (1968). Duck plague on the American continent. Avian Diseases, 12, 361–378. doi: 10.2307/1588237
- Liu, J., Chen, P., Jiang, Y., Wu, L., Zeng, X., Tian, G., Ge, J., Kawaoka, Y., Bu, Z. & Chen, H. (2011). A duck enteritis virus-vectored bivalent live vaccine provides fast and complete protection against H5N1 avian influenza virus infection in ducks. Journal of Virology, 85, 10989–10998. doi: 10.1128/JVI.05420-11
- Luo, Y., Glisson, J.R., Jackwood, M.W., Hancock, R.E., Bains, M., Cheng, I. & Wang, C. (1997). Cloning and characterization of the major outer membrane protein gene (ompH) of Pasteurella multocida X-73. Journal of Bacteriology, 179, 7856–7864. doi: 10.1128/JB.179.24.7856-7864.1997
- Marru, H.D., Anijajo, T.T. & Hassen, A.A. (2013). A study on Ovine pneumonic pasteurellosis: isolation and identification of Pasteurellae and their antibiogram susceptibility pattern in Haramaya District, Eastern Hararghe, Ethiopia. BMC Veterinary Research, 9, 239. doi: 10.1186/1746-6148-9-239
- Matheis, W., Zott, A. & Schwanig, M. (2001). The role of the adsorption process for production and control combined adsorbed vaccines. Vaccine, 20, 67–73. doi: 10.1016/S0264-410X(01)00317-6
- Mervat, A.E.-K., Khodeir, M.H., Abd-El-Khaleeck, M.A. & Abou El-Khair, M.A. (1999). Preparation of combined inactivated vaccine against duck virus hepatitis and duck virus enteritis. Alexandria Journal of Veterinary Sciences, 15, 627–637.
- Nawathe, D.R. & Ayoola, S.O. (1981). Experiments with a combined vaccine for poultry. Bulletin of Animal Health and Production in Africa, 29, 65.
- OIE Terrestrial Manual. (2015). Chapter 3.3.9 – fowl cholera.
- Pedersen, K., Dietz, H.H., Jørgensen, J.C., Christensen, T.K., Bregnballe, T. & Andersen, T.H. (2003). Pasteurella multocida from outbreaks of avian cholera in wild and captive birds in Denmark. Journal of Wildlife Diseases, 39, 808–816. doi: 10.7589/0090-3558-39.4.808
- Pinheiro, J., Bates, D., DebRoy, S., Sarkar, D. & R Core Team (2018). nlme: linear and nonlinear mixed effects models. R package version 3.1-137.
- Pongsopida, A., Taweechotipatr, P. & Srimahasongcram, S. (1987). Fowl cholera and duck plague vaccine 1. III. The immunity of combined vaccines, formalinized bacterin plus attenuated duck plague vaccines: a preliminary report. Wetchasan Sattawaphaet, 15, 7–9.
- Poolperm, P., Apinda, N., Kataoka, Y., Suriyasathaporn, W., Tragoolpua, K., Sawada, T. & Sthitmatee, N. (2018). Protection against Pasteurella multocida conferred by an intranasal fowl cholera vaccine in Khaki Campbell ducks. Japanese Journal of Veterinary Research, 66, 239–250.
- Poolperm, P., Varinrak, T., Kataoka, Y., Tragoolpua, K., Sawada, T. & Sthitmatee, N. (2017). Development and standardization of an in-house indirect ELISA for detection of duck antibody to fowl cholera. Journal of Microbiological Methods, 142, 10–14. doi: 10.1016/j.mimet.2017.08.018
- Qi, X.F., Cheng, A.C., Wang, M.S., Yang, X.Y., Jia, R.Y. & Chen, X.Y. (2007). Development of an indirect-ELISA kit for detection of antibodies against duck plague virus. Veterinary Science in China, 37, 690–694.
- Samuel, M.D., Botzler, R.G. & Wobeser, G.A. (2007). Avian cholera. In N.J. Thomas, D. B. Hunter, & C.T. Atkinson (Eds.), Infectious diseases of wild birds (pp. 239–269). Ames: Blackwell Publishing Ltd.
- Sharif, A. & Ahmad, T. (2018). Preventing vaccine failure in poultry flocks. IntechOpen, chapter 6.
- Skibinski, D.A., Baudner, B.C., Singh, M. & T O’Hagan, D. (2011). Combination vaccines. Journal of Global Infectious Diseases, 3, 63. doi: 10.4103/0974-777X.77298
- Sthitmatee, N., Kataoka, Y. & Sawada, T. (2011). Inhibition of capsular protein synthesis of Pasteurella multocida strain P-1059. Journal of Veterinary Medical Science, 73, 1445–1451. doi: 10.1292/jvms.11-0282
- Sthitmatee, N., Numee, S., Kawamoto, E., Sasaki, H., Yamashita, K., Takahashi, N., Kataoka, Y. & Sawada, T. (2008). Protection of chickens from fowl cholera by vaccination with recombinant adhesive protein of Pasteurella multocida. Vaccine, 26, 2398–2407. doi: 10.1016/j.vaccine.2008.02.051
- Sthitmatee, N., Yano, T., Lampang, K.N., Suphavilai, C., Kataoka, Y. & Sawada, T. (2013). A 39-kDa capsular protein is a major cross-protection factor as demonstrated by protection of chickens with a live attenuated Pasteurella multocida strain of P-1059. Journal of Veterinary Medical Science, 75, 923–928. doi: 10.1292/jvms.12-0475
- Thanasarasakulpong, A., Poolperm, P., Tangjitjaroen, W., Varinrak, T., Sawada, T., Pfeiffer, D. & Sthitmatee, N. (2016). Comparison of the effect of two purification methods on the immunogenicity of recombinant outer membrane protein H of Pasteurella multocida serovar A:1. Veterinary Medicine International. Article ID 2579345, 7 pages.
- Thanasarasakulpong, A., Poolperm, P., Tankaew, P., Sawada, T. & Sthitmatee, N. (2015). Protectivity conferred by immunization with intranasal recombinant outer membrane protein H from Pasteurella multocida serovar A: 1 in chickens. Journal of Veterinary Medical Science, 77, 321–326. doi: 10.1292/jvms.14-0532
- Varinrak, T., Muenthaisong, A., Apinda, N., Sawada, T. & Sthitmatee, N. (2019). Construction and characterization of an OmpH deficient mutant of Pasteurella multocida strain X-73. Avian Pathology, 48, 4–11. doi: 10.1080/03079457.2018.1533239
- Varinrak, T., Poolperm, P., Sawada, T. & Sthitmatee, N. (2017). Cross-protection conferred by immunization with an rOmpH-based intranasal fowl cholera vaccine. Avian Pathology, 46, 515–525. doi: 10.1080/03079457.2017.1321105
- Wen, Y., Cheng, A., Wang, M., Ge, H., Shen, C., Liu, S., Xiang, J., Jia, R., Zhu, D., Chen, X., Lian, B., Chang, H. & Zhou, Y. (2010). A thymidine kinase recombinant protein-based ELISA for detecting antibodies to duck plague virus. Virology Journal, 7, 77. doi: 10.1186/1743-422X-7-77
- Wu, P., Lu, J., Feng, L., Wu, H., Zhang, X., Mei, M., Hou, J., Liu, X. & Tang, Y. (2017). Antigen-sparing and enhanced efficacy of multivalent vaccines adjuvanted with immunopotentiators in chickens. Frontiers in Microbiology, 8, 927. doi: 10.3389/fmicb.2017.00927
- Wu, Y., Cheng, A., Wang, M., Zhang, S., Zhu, D., Jia, R., Luo, Q., Chen, Z. & Chen, X. (2011). Serologic detection of duck enteritis virus using an indirect ELISA based on recombinant UL55 protein. Avian Diseases, 55, 626–632. doi: 10.1637/9766-042111-Reg.1