ABSTRACT
Infectious laryngotracheitis virus (ILTV) DNA has been detected in blood fractions, but the cell phenotype with which the virus is associated is unknown. This study investigated the presence of ILTV antigen in peripheral blood cells of six acutely ILTV-infected chickens (5 or 9 days post ocular inoculation with a virulent isolate) and three sham-inoculated chickens using immunofluorescent staining. Blood fractions were separated by Ficoll-Paque density gradient centrifugation, and smears were prepared from erythrocyte and leukocyte fractions. The smears were stained for ILTV glycoprotein E and the leukocyte markers CD4, CD8, Bu-1 (B cell), KUL01 (monocyte/macrophage), TCRγδ, and TCRαβ/Vβ2 and examined under a confocal microscope. In samples from infected birds, ILTV gE-specific fluorescence was localized in B cells and all evaluated T cell types, but not in monocytes and erythrocytes. The percentage of CD4, CD8, TCRγδ, TCRαβ/Vβ1, TCRαβ/Vβ2 and B cells positive for ILTV antigen ranged from 13.3% to 22.3%. None of the samples from the sham-inoculated chickens exhibited fluorescence for ILTV gE. The results of this pilot study suggest that ILTV has a tropism for peripheral blood T and B cells. Further research is required to investigate whether these cells support ILTV productive replication.
Selective tropism of ILTV for peripheral blood cells was demonstrated in acutely infected birds.
The ILTV antigen gE was detected in blood CD4, CD8, TCRγδ, TCRαβ and B cells but not in monocytes and erythrocytes.
The highest percentage of ILTV antigen was observed in CD4 cells (22.3%) followed by TCRαβ/Vβ1 (20.6%), CD8 (15.4%), TCRαβ/Vβ2 or B cells (14.4%) and TCRγδ cells (13.3%).
RESEARCH HIGHLIGHTS
Introduction
Infectious laryngotracheitis (ILT) is a highly contagious upper respiratory disease of chickens that causes significant economic losses in major poultry producing areas due to immunization costs, increased mortality, and reduced weight and egg production (Perez et al., Citation2019). This disease is caused by Gallid alphaherpesvirus 1, traditionally known as infectious laryngotracheitis virus (ILTV), a member of the genus Iltovirus, subfamily Alphaherpesvirinae (Davison, Citation2010). ILTV enters the host through the respiratory, ocular and oral routes, causing characteristic clinical signs such as respiratory distress and conjunctivitis that commence 6–12 days after natural infection or 2–4 days after experimental inoculation (Hitchner et al., Citation1977; Robertson & Egerton, Citation1981; Bagust, Citation1986; Williams et al., Citation1992; Bagust et al., Citation2000).
Several studies have detected ILTV DNA in a plethora of organs outside of the respiratory tract and conjunctival tissue (Oldoni et al., Citation2009; Wang et al., Citation2013; Zhao et al., Citation2013; Roy et al., Citation2015; Davidson et al., Citation2016; Tran et al., Citation2021). Though Zhao et al. (Citation2013) reported the presence of ILTV antigen in a range of organs including heart, liver, spleen, kidney, larynx, thymus, intestine, bursa of Fabricius, and brain, Tran et al. (Citation2021) failed to detect ILTV antigen in organs other than conjunctiva, trachea and lung. It has been speculated that free ILTV in blood or ILTV associated with immune cells might be responsible for the PCR detection in these tissues (Oldoni et al., Citation2009; Roy et al., Citation2015; Davidson et al., Citation2016) or be involved in the spread of the virus to organs that support replication (Wang et al., Citation2013; Zhao et al., Citation2013). Although ILTV could not be isolated from the blood of infected chickens in early studies (Chang et al., Citation1973; Bagust, Citation1986), in vitro studies have shown that some strains of ILTV can infect leukocytes, more specifically macrophages, with no evidence of lymphocyte infection (Chang et al., Citation1977; Calnek et al., Citation1986). Recently, Tran et al. (Citation2021) reported ILTV DNA in blood fractions of ILTV-infected chickens using quantitative PCR (qPCR), with the leukocyte fraction displaying a higher viral load than the plasma and erythrocyte fractions. This pilot study aimed to phenotype the immune cells which harbour the ILTV surface glycoprotein E (gE) antigen in the peripheral blood of infected chickens using immunofluorescent staining.
Materials and methods
Blood sample collection and processing
Blood samples were collected from nine commercial meat chickens at 5 or 9 days post ocular inoculation (dpi) with ILTV class 9 field strain NSW/18 B2P3 (n = 5, three chickens at 5 dpi and two chickens at 9 dpi) or sham-inoculated (n = 3, two chickens at 5 dpi and one chicken at 9 dpi) as part of a separate study (unpublished data). Briefly, 10-day-old chickens were inoculated with 60 µl containing 103 TCID50 ILTV (30 µl in each eye) or the same volume of sterile cell culture medium. ILTV-infected birds were selected for blood sampling based on characteristic clinical signs such as moderate to severe conjunctivitis with complete closure of one or both eyes on the day of sample collection. Two of three ILTV-infected birds sampled at 5 dpi presented with respiratory distress including gasping. The University of New England Animal Ethics Committee approved (AEC20-041) this experiment prior to its conduct. Presence or absence of ILTV infection was confirmed using qPCR on choanal cleft swabs collected at 4 dpi as previously described (Nazir, Yegoraw et al., Citation2020). Blood samples were collected from the brachial vein (1 ml per bird) into a microtube containing 0.3 ml of 3.2% sodium citrate anticoagulant. One millilitre of citrated blood was layered on an equal volume of Ficoll-Paque density gradient 1.077 (GE Healthcare Biosciences AB, Sweden) and centrifuged for 20 min at 400 × g. The plasma fraction on the supernatent was discarded, and leukocytes from the buffy coat fraction were transferred to a clean microtube, washed with phosphate-buffered saline pH 7.4 (PBS), centrifuged at 350 × g for 5 min and resuspended in 100 μl of PBS. Erythrocytes collected from the bottom of the tube were transferred to a separate microtube and resuspended in 100 μl of PBS. Nine smears of the leukocyte fraction and two smears of the erythrocyte fraction from each bird were immediately prepared on positively charged Superfrost plus slides (ThermoFisher Scientific, Waltham, MA, USA). After drying for 2 h, the slides were fixed with cold acetone for 8 min. The smears were either immediately processed for immunofluorescence staining or were stored at −80°C until further use.
Immunofluorescence staining
A dual immunofluorescence assay was used to co-label the ILTV antigen and the extracellular leukocyte markers. Prepared smears were washed with PBS three times and an area covering approximately two-thirds of the smear was encircled with a PAP pen (Dako, Glostrup, Denmark). For blocking non-specific binding, smears were washed with PBS three times and incubated with immunofluorescence buffer (PBS with 1% bovine serum albumin, 2% foetal bovine serum, 0.2% Tween 20) in a humidified chamber at room temperature for 30 min. The blocking buffer was tapped off, and the smears were subsequently incubated at room temperature for 1 h in a humid chamber with one of the following antibodies from Southern Biotech (Birmingham, AL, USA) against immune cell markers: mouse anti-chicken-CD4-FITC (clone CT-4) (at 1:50 dilution in immunofluorescence buffer), mouse anti-chicken-CD8-FITC (clone CT-8) (1:50), mouse anti-chicken-Bu1 (B cell)-FITC (clone AV20) (1:100), mouse anti-chicken monocyte/macrophage-FITC (clone KUL01) (1:50), mouse anti-chicken TCRγδ-FITC (clone TCR-1) (1:50), mouse anti-chicken TCRαβ/Vβ1-FITC (clone TCR-2) (1:25), mouse anti-chicken TCRαβ/Vβ2-FITC (clone TCR-3) (1:25). For detection of ILTV antigen, after washing with PBS, smears were stained using rabbit anti-ILTV gE-Alexa Fluor 647 (Bioss, Woburn, MA, USA) (1:200) for 1 h (Nazir, Charlesworth et al., Citation2020). Erythrocyte smears were incubated with the anti-ILTV gE antibody. Antibodies were replaced by immunofluorescence buffer or isotype controls to serve as a control for the non-specific binding. The isotype controls used included mouse IgG1-FITC (Southern Biotech) for cell markers and rabbit IgG-Alexa Fluor 647 (Bioss) for ILTV antigen. Smears prepared from a chicken embryo origin live attenuated ILTV vaccine (Poulvac Laryngo SA2, Zoetis) were used as a positive control for the detection of ILTV antigen. After washing with PBS containing 0.025% Tween 20, slides were incubated with 4′, 6-diamidino-2-phenylindole (DAPI) (Sigma Aldrich, St. Louis, MO, USA) for 10 min for nuclear counterstaining (1:10,000 dilution in PBS). The slides were coverslipped with VECTASHIELD mounting medium (Vector Laboratories, Burlingame, CA, USA).
Confocal microscopy
The smears were examined with a laser scanning confocal microscope (Nikon C1 with a Ti-E motorized inverted microscope) with a Plan Fluor ELWD 20× C NA 0.45, WD 8 objective lens. A Coherent Cube 405-50 (405 nm, 50 mW) Solid state laser, a Coherent Sapphire 488-20 (488 nm, 20 mW) CW laser and a Coherent Compass 215M-20 (532 nm, 20 mW) CW Laser (Coherent, Inc., Santa Clara, CA, USA) were used to excite the fluorophores. Images were acquired for nuclei (DAPI stained) using a Nikon UV-2A filter block, leukocyte markers (green channel) by a Brightline 512/25 nm bandpass filter and followed by a 605/75 nm bandpass barrier filter for ILTV antigen (red channel). The pinhole and pixel dwell time were set at 33 µm and 30 µs, respectively. The gain of the photomultiplier tube and the laser power were maintained constant throughout the work. The entire smear was examined under low magnification (10×) and five arbitrary regions (each with an area of 0.34 mm2) within each smear were chosen for counting of cells. A positive smear for the ILTV antigen was identified visually by a clear red fluorescence within the cytoplasm of the leukocytes, whereas a clear green fluorescence within a cell indicated the presence of a marker for a specific leukocyte type.
Results
Results of immunofluorescence testing of erythrocytes and leukocyte smears for detection of ILTV antigen are presented in and . All five challenged birds tested positive for ILTV DNA in choanal cleft swabs, with ILTV log10 genomic copies per swab ranging from 5.2 to 9.6 (). The choanal swabs from all three sham-inoculated chickens were negative for ILTV DNA. Dual immunofluorescence staining of leukocyte types and viral antigen demonstrated a clear specific fluorescence signal for viral antigen (gE) in B cells, T cell subtypes TCRγδ, TCRαβ/Vβ1 and TCRαβ/Vβ2, and CD4 and CD8 cells in leukocyte samples from ILTV-inoculated chickens ().
Figure 1. Immunofluorescence staining for ILTV glycoprotein E in representative blood cells. A, B, C, D, E, F and G plots respectively represent an ILTV live attenuated vaccine (positive control), CD4 cell, CD8 cell, γδ T cell (TCR1), TCRαβ/Vβ1 (TCR2), TCRαβ/Vβ2 (TCR3) and B cell positive for ILTV glycoprotein E antigen. The yellow colour or green/red merge demonstrates that the probes are co-localized within the same optical plane. Monocyte (H), and erythrocyte smear (I) with no visible staining for ILTV are also shown. A leukocyte smear (J) from an uninfected bird (stained for CD4) is shown as negative control for ILTV antigen.
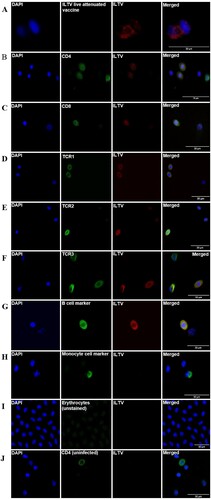
Table 1. Proportion of ILTV antigen-positive blood leukocytes and ILTV log10 genomic copies (GC) per swab in choanal cleft swabs of birds at 5 and 9 days post-inoculation (dpi). nd = not detected.
Although the low number of birds precluded statistical analysis, a numerically higher proportion of ILTV antigen was detected in the immune cell subtypes at 5 dpi compared to 9 dpi (CD4: 32.7% vs 17%, CD8: 17.5% vs 12.5%, TCRγδ: 31.1% vs 7%, TCRαβ/Vβ1: 28.6% vs 9.8%, TCRαβ/Vβ2: 23.1% vs 6.8%, B cell: 17.7% vs 14.4%). Overall comparison of leukocyte types revealed that CD4 was the predominant cell carrying the ILTV antigen (22.3%) followed by TCRαβ/Vβ1 (20.6%), CD8 (15.4%), TCRαβ/Vβ2 or B cell (14.4) and lastly TCRγδ cells (13.3%). In contrast, no signal for ILTV antigen was detected in erythrocytes or monocytes in smears from the same birds. None of the smears from sham-inoculated chickens showed fluorescence for ILTV gE. Nonspecific fluorescence was not observed when staining was performed by replacing antibodies with immunofluorescence buffer or isotype controls.
Discussion
Under the conditions of this study, ILTV gE antigen was detected in peripheral blood B cells and T cell subsets from ocularly ILTV-inoculated chickens during acute stages of infection, while no antigen was detected in monocytes and erythrocytes. The strong ILTV gE signals in B and T cells suggest possible viral replication in these cells, although this could not be demonstrated in this pilot study. Similar to our findings, Marek’s disease virus, another alphaherpesvirus of chickens, is known to infect B and T cells (Calnek et al., Citation1984; Baigent et al., Citation1998). Previous studies failed to isolate ILTV from blood of ILTV-infected chickens using in vitro (Chang et al., Citation1973; Bagust, Citation1986) and in vivo (Yegoraw et al., Citation2021) methods.
This study demonstrated that cells expressing CD4 were the predominant cells carrying ILTV antigen. Higher susceptibility of CD4 cells compared to CD8 cells has been reported for other herpesviruses including Marek’s disease virus (Calnek et al., Citation1984; Baigent et al., Citation1998) and equine herpesvirus 1 (Poelaert et al., Citation2019). Further studies utilizing flow cytometry to examine a larger number of leukocytes in a wider number of samples should be explored to validate these findings. The higher percentage of leukocytes carrying the ILTV antigen at 5 dpi seen in this study correlates with the peak of viral replication in the upper respiratory tract using the same infection model used here (Nazir, Yegoraw et al., Citation2020). This further supports the assumption on the role of immune cells in the dissemination of the virus to visceral organs.
The lack of ILTV gE detection in monocytes in the current study is in contrast to earlier in vitro studies in which ILTV replicated and formed typical multinucleated giant cells in leukocytes, most likely in macrophages, while lymphocytes seemed not to be susceptible to ILTV infection (Chang et al., Citation1977; Calnek et al., Citation1986). Differences between studies may be related to differences in ILTV strains (Calnek et al., Citation1986), differences in the susceptibility of the chicken breeds (Loudovaris et al., Citation1991), or differences in methods of ILTV infection (in vivo versus in vitro). The absence of ILTV antigen detection in erythrocytes also differs from the findings of Tran et al. (Citation2021), who detected viral DNA in the erythrocytes of meat chickens inoculated with the same ILTV strain and method used in the current study. This disparity might be explained by the inherent lower sensitivity of immunostaining techniques compared to molecular assays (Zhao et al., Citation2013; Preis et al., Citation2014).
T cells, particularly CD8 T cells, play an important role in halting ILTV replication (Beltrán et al., Citation2019). On the other hand, a humoral immune response involving B cells and antibodies has no significant protective role against ILTV (Fahey et al., Citation1983; Fahey et al., Citation1984; Fahey & York, Citation1990). Infection of the cellular arm of the immune response may contribute to virus evasion and dissemination within the host, as reported for other herpesviruses, including Marek’s disease virus and equine herpesvirus-1 (Calnek et al., Citation1984; Poelaert et al., Citation2019). Further investigation will be required to determine if the lymphopenia and leukopenia associated with ILTV infection (Chang et al., Citation1977) are due to the direct virus-induced apoptosis or virus lytic replication in these cells. The presence of ILTV antigen in peripheral blood lymphocytes from this study is consistent with the idea of a circulating form of the virus, that may explain detection of ILTV genome in a wide range of organs (including feathers) outside the respiratory tract and conjunctiva (Wang et al., Citation2013; Zhao et al., Citation2013; Roy et al., Citation2015; Davidson et al., Citation2016).
Conclusions
The tropism of ILTV for peripheral blood lymphocytes may have implications for this disease’s pathogenesis, including dissemination of the virus within the host and possible compromise of T leukocyte function. Further studies should be conducted to better understand the virus-cell interactions and confirm the ILTV replication in these cells and the proportion of cell subtypes infected with ILTV in a larger number of samples from naturally infected birds.
Acknowledgements
The authors would like to acknowledge Jon Clay for providing technical assistance during laboratory work. SN is the recipient of the Australian Government Research Training Programme scholarship.
Disclosure statement
No potential conflict of interest was reported by the authors.
Additional information
Funding
References
- Bagust, T. (1986). Laryngotracheitis (gallid-1) herpesvirus infection in the chicken 4. Latency establishment by wild and vaccine strains of ILT virus. Avian Pathology, 15, 581–595.
- Bagust, T., Jones, R. & Guy, J. (2000). Avian infectious laryngotracheitis. Revue Scientifique et Technique-Office International des Epizooties, 19, 483–488.
- Baigent, S.J., Ross, L. & Davison, T. (1998). Differential susceptibility to Marek's disease is associated with differences in number, but not phenotype or location, of pp38+ lymphocytes. Journal of General Virology, 79, 2795–2802.
- Beltrán, G., Hurley, D.J., Gogal, R.M., Sharif, S., Read, L.R., Williams, S.M., Jerry, C.F., Maekawa, D.A. & García, M. (2019). Immune responses in the eye-associated lymphoid tissues of chickens after ocular inoculation with vaccine and virulent strains of the respiratory infectious laryngotracheitis virus (ILTV). Viruses, 11, 635.
- Calnek, B., Fahey, K. & Bagust, T. (1986). In vitro infection studies with infectious laryngotracheitis virus. Avian Diseases, 30, 327–336.
- Calnek, B., Schat, K., Ross, L. & Chen, C.L. (1984). Further characterization of Marek's disease virus-infected lymphocytes. II. In vitro infection. International Journal of Cancer, 33, 399–406.
- Chang, P., Jasty, V., Fry, D. & Yates, V. (1973). Replication of a cell-culture-modified infectious laryngotracheitis virus in experimentally infected chickens. Avian Diseases, 17, 683–689.
- Chang, P., Sculco, F. & Yates, V. (1977). An in vivo and in vitro study of infectious laryngotracheitis virus in chicken leukocytes. Avian Diseases, 21, 492–500.
- Davidson, I., Raibshtein, I., Altori, A. & Elkin, N. (2016). Infectious laryngotracheitis virus (ILTV) vaccine intake evaluation by detection of virus amplification in feather pulps of vaccinated chickens. Vaccine, 34, 1630–1633.
- Davison, A.J. (2010). Herpesvirus systematics. Veterinary Microbiology, 143, 52–69.
- Fahey, K., Bagust, T. & York, J. (1983). Laryngotracheitis herpesvirus infection in the chicken: the role of humoral antibody in immunity to a graded challenge infection. Avian Pathology, 12, 505–514.
- Fahey, K., York, J. & Bagust, T. (1984). Laryngotracheitis herpesvirus infection in the chicken. II. The adoptive transfer of resistance with immune spleen cells. Avian Pathology, 13, 265–275.
- Fahey, K.J. & York, J.J. (1990). The role of mucosal antibody in immunity to infectious laryngotracheitis virus in chickens. Journal of General Virology, 71, 2401–2405.
- Hitchner, S., Fabricant, J. & Bagust, T. (1977). A fluorescent-antibody study of the pathogenesis of infectious laryngotracheitis. Avian Diseases, 21, 185–194.
- Loudovaris, T., Yoo, B. & Fahey, K. (1991). Genetic resistance to infectious laryngotracheitis in inbred lines of White Leghorn chickens. Avian Pathology, 20, 357–361.
- Nazir, S., Charlesworth, R.P., Walkden-Brown, S.W. & Gerber, P.F. (2020). A Melanin bleaching method to prevent non-specific immunostaining of chicken feathers. MethodsX, 7, 100957.
- Nazir, S., Yegoraw, A.A., Charlesworth, R.P., Williamson, S., Sharpe, S., Walkden-Brown, S.W. & Gerber, P.F. (2020). Marked differences in virulence of three Australian field isolates of infectious laryngotracheitis virus in meat and layer chickens. Avian Pathology, 49, 1–27.
- Oldoni, I., Rodriguez-Avila, A., Riblet, S.M., Zavala, G. & Garcia, M. (2009). Pathogenicity and growth characteristics of selected infectious laryngotracheitis virus strains from the United States. Avian Pathology, 38, 47–53.
- Perez, D., Carnaccini, S., Cardenas-Garsia, S., Ferreri, L., Santos, J. & Rajao, D. (2019). Infectious Laryngotracheitis Virus. In Avian virology: current research and future trends (ed. Samal SK) pp. 317-344. Caister Academic Press, Virginia-Maryland College of Veterinary Medicine, University of Maryland.
- Poelaert, K.C., Van Cleemput, J., Laval, K., Favoreel, H.W., Couck, L., Van den Broeck, W., Azab, W. & Nauwynck, H.J. (2019). Equine herpesvirus 1 bridles T lymphocytes to reach its target organs. Journal of Virology, 93, e02098-18.
- Preis, I., Fiúza, A., Silva, C., Braga, J., Couto, R., Martins, N.D.S. & Ecco, R. (2014). Pathological, immunohistochemical, and molecular findings in commercial laying hens and in backyard chickens naturally infected with the infectious laryngotracheitis virus. Revista Brasileira de Ciência Avícola, 16, 359–366.
- Robertson, G. & Egerton, J. (1981). Replication of infectious laryngotracheitis virus in chickens following vaccination. Australian Veterinary Journal, 57, 119–123.
- Roy, P., Islam, A.F., Burgess, S.K., Hunt, P.W., McNally, J. & Walkden-Brown, S.W. (2015). Real-time PCR quantification of infectious laryngotracheitis virus in chicken tissues, faeces, isolator-dust and bedding material over 28 days following infection reveals high levels in faeces and dust. Journal of General Virology, 96, 3338–3347.
- Tran, T.T., Nazir, S., Yegoraw, A.A., Assen, A.M., Walkden-Brown, S.W. & Gerber, P.F. (2021). Detection of infectious laryngotracheitis virus (ILTV) in tissues and blood fractions from experimentally infected chickens using PCR and immunostaining analyses. Research in Veterinary Science, 134, 64–68.
- Wang, L.-G., Ma, J., Xue, C.-Y., Wang, W., Guo, C., Chen, F., Qin, J.P., Huang, N.H., Bi, Y.Z. & Cao, Y.C. (2013). Dynamic distribution and tissue tropism of infectious laryngotracheitis virus in experimentally infected chickens. Archives of Virology, 158, 659–666.
- Williams, R., Bennett, M., Bradbury, J., Gaskell, R., Jones, R. & Jordan, F. (1992). Demonstration of sites of latency of infectious laryngotracheitis virus using the polymerase chain reaction. Journal of General Virology, 73, 2415–2420.
- Yegoraw, A.A., Assen, A.M., Gerber, P.F. & Walkden-Brown, S.W. (2021). Transmission of infectious laryngotracheitis virus vaccine and field strains: the role of degree of contact and transmission by whole blood, plasma and poultry dust. Veterinary Research, 52, 91.
- Zhao, Y., Kong, C., Cui, X., Cui, H., Shi, X., Zhang, X., Hu, S., Hao, L. & Wang, Y. (2013). Detection of infectious laryngotracheitis virus by real-time PCR in naturally and experimentally infected chickens. PLoS One, 8, e67598.