ABSTRACT
The pathogenicity of the H5 subtype high pathogenicity avian influenza viruses (HPAIVs) in Ardeidae bird species has not been investigated yet, despite the increasing infections reported. Therefore, the present study aimed to examine the susceptibility of the Ardeidae species, which had already been reported to be susceptible to HPAIVs, to a clade 2.3.2.1 H5N1 HPAIV. Juvenile herons (four grey herons, one intermediate egret, two little egrets, and three black-crowned night herons) were intranasally inoculated with 106 50% egg infectious dose of the virus and observed for 10 days. Two of the four grey herons showed lethargy and conjunctivitis; among them, one died at 6 days post-inoculation (dpi). The viruses were transmitted to the other two cohoused naïve grey herons. Some little egrets and black-crowned night herons showing neurological disorders died at 4–5 dpi; these birds mainly shed the virus via the oral route. The viruses predominantly replicated in the brains of birds that died of infection. Seroconversion was observed in most surviving birds, except some black-crowned night herons. These results demonstrate that most Ardeidae species are susceptible to H5 HPAIVs, sometimes with lethal effects. Herons are mostly colonial and often share habitats with Anseriformes, natural hosts of influenza A viruses; therefore, the risks of cluster infection and contribution to viral dissemination should be continuously evaluated.
Clade 2.3.2.1 H5N1 HPAIV causes lethal infections in Ardeidae sp.
Viruses are transmitted among grey herons.
Some herons with HPAIV showed conjunctivitis or neurological symptoms.
HPAIV systemically replicated in herons tissues.
RESEARCH HIGHLIGHTS
Introduction
Herons, belonging to class Aves, order Pelecaniformes, family Ardeidae, are a group of waterfowl mainly found in the tropics but are spread worldwide (Martínez-Vilalta & Motis, Citation1994). They are essentially non-swimmers that live in the margins of wetlands or aquatic zones. Most Ardeidae members, including herons, perform seasonal migrations, involving the entire population, or part of the population. Thus, some heron species are frequently observed together with other migratory waterfowls, such as ducks and swans, which are considered natural reservoirs of the influenza A virus (Alexander, Citation1982). The influenza A virus is classified by the antigenicity and/or gene sequences of its surface glycoproteins, haemagglutinin (HA) and neuraminidase (NA), into HA subtypes H1–H18 and NA subtypes N1–N11 (Webster et al., Citation1992; Fouchier et al., Citation2005; Yang et al., Citation2021). To date, the H1–H16 and N1–N9 subtypes have been isolated from ducks, swans, and other wild waterfowl. Herons are also considered susceptible to the influenza A virus as antibodies against this virus were detected in 3.1% of grey herons (Ardea cinerea) at the Doñana National Park in Spain (Astorga et al., Citation1994), and low-level antibodies against H2, H9, and H10 subtype influenza viruses were detected in little egrets (Egretta garzetta) and black-crowned night herons (Nycticorax nycticorax) (Chen et al., Citation2006).
The influenza A virus which originally perpetuated in waterfowl populations does not exhibit pathogenicity in chickens (OIE, Citation2021b). However, these viruses can pass through terrestrial poultry, such as quails and turkeys, and infect chicken populations. Among them, H5 and H7 viruses are generally adapted to chickens and acquire pathogenicity (Alexander, Citation1982; Webster et al., Citation1992). The H5 subtype high pathogenicity avian influenza viruses (HPAIVs) have spread to domestic poultry and wildlife in Asia, Europe, the Middle East, Africa, and North America since late 1996, when the virus recognised as the precursor of present circulating viruses (Gs/GD-like viruses) was found in a goose in the Guangdong province, China (Xu et al., Citation1999). The HA genes of these progeny viruses have been phylogenetically organised into 10 clades, numbered 0–9 (WHO/OIE/FAO-Evolution-Working-Group, Citation2008). Initially, high pathogenicity avian influenza (HPAI) outbreaks were observed in gallinaceous birds, and lethal Gs/GD-like virus infection in domestic/wild waterfowl or other wild birds was not reported. However, in 2002, HPAI caused by clade 1 H5N1 viruses occurred for the first time in waterfowl and other captive/wild birds at two parks and a set of mitigation ponds in Hong Kong (Ellis et al., Citation2004). In this report, birds of the family Ardeidae were infected with HPAIV, as well as ducks and swans; about 20 little egrets died at the beginning of the outbreak in Penfold Park, and two dead grey herons were found in ponds, approximately 20 km from Penfold Park. HPAIV infection in herons was subsequently reported in Hong Kong between 2004 and 2009, and clade 2.3.2 and 2.3.4 HPAIVs were isolated from grey herons, great egrets (Ardea alba), little egrets, black-crowned night herons, and Chinese pond herons (Ardeola bacchus) (GISAID, Citation2021; Smith et al., Citation2009). Herons infected with H5N1 HPAIVs were also found in Austria (Fink et al., Citation2010), Cambodia (GISAID, Citation2021), Egypt (Soliman et al., Citation2012), France (Hars et al., Citation2008), Indonesia (GISAID, Citation2021), Japan (Soda, Ito, et al., Citation2013), and Romania (GISAID, Citation2021) between 2005 and 2011. Since the novel clade 2.3.4.4 HPAIV emerged in southern China in late 2013 (Lee, Bertran, et al., Citation2017), H5Nx HPAIVs in this clade have become predominant in herons, as well as other bird species, in Asian and European countries. H5N5, H5N6, and H5N8 HPAIVs have been isolated in Bulgaria (GISAID, Citation2021), Czech Republic (GISAID, Citation2021), Germany (Pohlmann et al., Citation2018), Italy (GISAID, Citation2021), Korea (Woo et al., Citation2017), Mainland China (Luo et al., Citation2018), Vietnam (GISAID, Citation2021), and border areas between Mongolia and Russia (Lee, Sharshov, et al., Citation2017). H5N1 HPAIVs have also been enzootically maintained in Egypt: clade 2.2.1 H5N1 HPAIV was found in cattle egrets (Bubulcus ibis) in 2014 (Ahmed et al., Citation2017). Serological surveillance showed that herons had antibodies against H5 HPAIVs in various clades: some little egrets and black-crowned night herons in the city park in Jiangxi (China) had antibodies against clade 7 H5 HPAIV in egg yolk samples in 2009 (Wang et al., Citation2014), and a pond heron was seropositive against clade 1 HPAIV in central Thailand (Siengsanan-Lamont et al., Citation2011). These reports support the notion that herons are susceptible to H5 HPAIV, suggesting that they might be involved in HPAIV ecology. However, the pathogenicity of HPAIV in herons has not been experimentally demonstrated.
In the present study, we assessed the pathogenicity of H5N1 HPAIV in grey herons, an intermediate egret (Ardea intermedia), little egrets, and black-crowned night herons to estimate their contribution to the dissemination of HPAIVs in field settings. Among our chosen study subjects, East Asia to Far East populations of grey herons, little egrets, and black-crowned night herons entirely or partially migrate to southern China, where various novel H5 HPAIVs have emerged (Xu et al., Citation1999; Lee, Bertran, et al., Citation2017) and HPAIV infection cases have been reported in the field. In addition, the grey heron is among the birds that can be found farthest north, and their breeding sites include Siberia (Martínez-Vilalta & Motis, Citation1994). Similar to ducks and swans, the grey heron may also be involved in HPAIV maintenance and dissemination. To the best of our knowledge, this is the first study to report HPAIV pathogenicity in herons following experimental infection.
Materials and methods
Virus
A clade 2.3.2.1 HPAIV, A/mandarin duck/Miyazaki/22M807-1/2011 (H5N1) (M807), was used for experimental heron infection. The strain was isolated from a mixture of tracheal and cloacal swabs of a Mandarin duck found dead in Miyazaki City, in the Kyusyu region of Japan (Soda, Ito et al., Citation2013), and previously used to evaluate its pathogenicity in other bird species, such as Mandarin duck (Soda, Usui, et al., Citation2013), rook (Soda et al., Citation2020), and American kestrel (Uno et al., Citation2020). The accession numbers for the M807 gene sequences are AB677872–AB677879. The virus was propagated in 10-day-old chicken embryos (Aoki Breeder Farm, Tochigi, Japan) for 48 h at 35°C. After incubation, the eggs were chilled at 4°C for 12 h. The allantoic fluid was then harvested and stored as virus stock at −80°C.
Birds
Juvenile herons (six grey herons, one intermediate egret, two little egrets, and three black-crowned night herons) were captured at the bird colony in Kamisu City, Ibaraki, Japan, in agreement with the Ministry of the Environment and the prefectural governor (registration numbers: #24900042–#24900046). The birds were confirmed to be influenza virus-negative by testing oral/cloacal swab samples using an antigen detection kit (ESPLINE INFLUENZA A & B-N, Fujirebio Inc., Tokyo, Japan) and transported to Tottori University. The little egrets and black-crowned night herons were fed with farm-raised weather loaches (Misgurnus anguillicaudatus) (Yasugi Dojou Producer's Association, Shimane, Japan) and reared for 2 weeks before experimental infection. The grey herons and intermediate egret were fed with wild-caught Japanese jack mackerels (Trachurus japonicus), reared for 10 weeks, and shipped by air to Hokkaido University. The birds were confirmed to be seronegative by haemagglutinin inhibition (HI) testing (WHO, Citation2002) using the M807 strain, and then used for experimental infection.
Experimental infection
All birds, except two grey herons (ID: #GH05 and #GH06), were intranasally inoculated with 200 μl of allantoic fluid containing the M807 strain at 106 50% egg infectious dose (EID50). The herons #GH05 and #GH06 were placed in contact with four challenged grey herons on the same day of infection. No negative control animals were used in this study because the number of captured herons was limited. The signs of infection were observed at 24-h intervals for 10 days. Clinical signs were scored according to the method of intravenous pathogenicity index testing regulated by the OIE (OIE, Citation2021a). Oral and cloacal swabs were collected into 2 ml of nutrient broth medium (Nissui Pharmaceutical, Tokyo, Japan) with 10 mg streptomycin sulphate (Meiji Seika Pharma, Tokyo, Japan) and 1×104 units of penicillin G (Meiji Seika Pharma) at 0, 1, 2, 3, 5, 7, and 10 days post-inoculation (dpi). At the end of the 10-day period, the birds were checked for specific antibodies against M807 in serum by HI testing. These sera were also assayed for influenza A subtype viruses using a competitive ELISA (cELISA) kit (IDEXX Influenza A Ab test, IDEXX laboratories, Westbrook, ME, USA). The surviving birds were euthanized with isoflurane (FUJIFILM Wako Pure Chemical Corporation, Tokyo, Japan) after blood collection at 10 dpi. The birds that died of infection were necropsied, and their tissues (brain, trachea, lung, liver, pancreas, spleen, heart, kidney, colon, and breast muscle) were aseptically collected. A tissue sample was collected in 10% neutral buffered formalin (Wako Pure Chemical Industries, Ltd., Osaka, Japan) for histopathological study as described below, and another tissue sample was homogenised to create a 10% (w/v) organ emulsion in nutrient broth medium with antibiotics for virus isolation. The swabs and organ emulsions were serially ten-fold diluted in phosphate buffered saline with antibiotics, and inoculated into embryonated chicken eggs. The EID50 was calculated using the Reed and Muench method (Reed & Muench, Citation1938).
Ethics statement
Experimental infection in the little egrets and black-crowned night herons was performed in self-contained isolator units (CLEA Japan, Tokyo, Japan) at a biosafety level 3 (BSL3) facility at the Avian Zoonosis Research Centre, Tottori University, Japan. The experiment was performed according to the guidelines of the Institutional Animal Care and Use Committee of Tottori University (approval number: h24-T030). Experimental infection in the grey herons and intermediate egret was performed in an open-style BSL3 room at the International Institute for Zoonosis Control, Hokkaido University, Japan. The protocol was approved by the Hokkaido University Animal Care and Use Committee (Permit Number: 12-1160); the floor of the rearing spaces was cleaned every 24 h. All animal experiments were performed according to the Guidelines for Proper Conduct of Animal Experiments of the Science Council of Japan (http://www.scj.go.jp/ja/info/kohyo/pdf/kohyo-20-k16-2e.pdf). Humane endpoints were set as follows: birds unable to eat or drink were euthanized and recorded as dead at the following day's observation time.
Histopathological examination
The tissues fixed in 10% neutral buffered formalin were processed via routine methods, and embedded in paraffin wax. Sections were stained with haematoxylin and eosin for histopathological examination. Immunohistochemical staining was also performed using antigen retrieval solution (0.05% citraconic anhydride, pH 7.4; Immunosaver; Nissin EM, Tokyo, Japan), mouse anti-influenza A virus matrix protein monoclonal antibody (clone GA2B; Serotec Ltd., Oxford, UK), and the Simple Stain MAX-PO (M) kit (Nichirei Bioscience Inc., Tokyo, Japan) following manufacturer's instructions.
Results
H5N1 HPAIV pathogenicity and transmissibility in the grey herons
One of the challenged grey herons, #GH03, was severely depressed at 6 dpi and died on the same day (). #GH04 presented with lethargy and conjunctivitis at 6 dpi (); conjunctivitis remained until the end of the experiment, but recovery from lethargy was noted the following day (). The remaining two herons (#GH01-02) survived the 10-day observation period and remained asymptomatic (). Two contact herons also survived the experimental period, while #GH06 showed conjunctivitis similar to #GH04 at 10 dpi. All surviving herons seroconverted after 10 days, and higher titres of serum HI antibody were detected in the challenged birds than in the contact birds.
Figure 1. Conjunctival hyperaemia in a grey heron (#GH04) at 7 dpi with the H5N1 HPAIV. Inset indicates the appearance of a grey heron before the virus challenge.
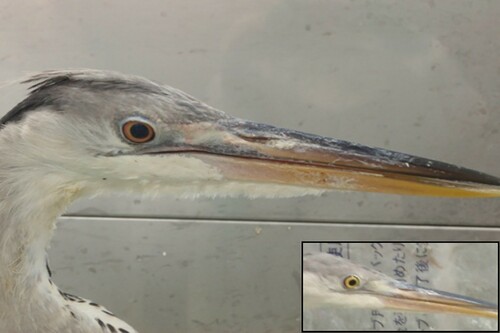
Table 1. Clinical signs and antibody responses of herons intranasally inoculated with H5N1 HPAIV.
The virus titres in the oral and cloacal swabs of the grey herons were determined by egg inoculation (). Herons #GH01-02, which showed subclinical infection, shed no virus via the cloaca, while slight viral shedding was sporadically observed in oral secretions at 2–5 dpi. Heron, #GH03, which died at 6 dpi, initially shed a low virus titre via the cloaca, and subsequently in oral secretions after 5 dpi. #GH04 consecutively excreted ≤103.7 EID50 of the virus via the oral and cloacal routes by 5 dpi before symptom onset ( and ); then onward, no virus shedding was observed. Contact herons (#GH05-06) showed delayed viral shedding (): viruses were detected in oral and cloacal swabs at 5 and 7 days post-contact, respectively. Notably, they shed a higher virus titre via the cloaca than inoculated herons.
Table 2. Viral shedding from herons intranasally inoculated with H5N1 HPAIV.
H5N1 HPAIV pathogenicity in the intermediate egret, little egrets, and black-crowned night herons
One intermediate egret, two little egrets, and three black-crowned night herons were intranasally inoculated with 106 EID50 of the M807 strain. Two little egrets (#LE01-02) and one black-crowned night heron (#NH02) showed severe clinical signs at 4 and 5 dpi, respectively, and died on the following days (); #LE02 intermittently shivered, and repeatedly vomited after eating weather loaches; #NH02 remained motionless with iterations of pupil dilation and constriction. These birds shed virus in oral secretions and via the cloaca before disease manifestation (); #LH01-02 shed ≤103.5 EID50 of virus in oral secretions, starting 1 dpi, and subsequently into the cloaca (≤102.7 EID50); #NH02 shed ≤104.3 EID50 of virus orally and cloacally between 3 and 6 dpi.
The intermediate egret and two black-crowned night herons survived for 10 days without showing any clinical signs (). #IE01 shed ≤104.3 EID50 of virus predominantly in oral secretions within 5 dpi () and showed clear seroconversion after the challenge (). Neither viral shedding nor antibody response was observed in the surviving #NH01 and #NH03 birds ().
Virus replication in herons that died of H5N1 HPAIV infection
Herons that died of infection (#GH03, #LE01-02, and #NH02) were necropsied and their tissue viral titres were determined by egg inoculation (). Viruses were recovered from almost all tested tissues of the dead birds. Relatively higher virus titres were confirmed in the brain, lung, heart, and colon; the brain showed the highest viral titres (106.5–7.7 EID50) in all dead birds. Interestingly, viruses were not recovered from the trachea and breast muscle of #GH03, unlike the other birds.
Table 3. Viral titres/antigen-detection in the tissues of herons that died after H5N1 HPAIV infection.
Histopathology and viral antigen distribution in herons
Histopathological findings and virus antigen distribution in birds that died of viral infection were assessed (). Histopathological changes were mainly observed in the brains: non-purulent encephalitis, consisting of multifocal to coalescing neuronal degeneration and necrosis with minimal glial nodules and perivascular mononuclear cell cuffing was observed in all dead birds. Little egrets (#LE01 and #LE02) and the black-crowned night heron (#NH02) had mild multifocal myocardial degeneration with mononuclear cell infiltration in the heart. Necrotic foci in the renal tubules and splenic follicles were sometimes observed in #LE02, suspected to be associated with bacterial sepsis. Viral antigens were distributed in the brains of all dead birds ( and ) in the nucleus of meningeal and glial cells, and nucleus/cytoplasm of affected neurons. #LE01-02 and #NH02, in contrast to #GH03, had viral antigens in their hearts (nucleus/cytoplasm of affected cardiomyocytes) and lungs (nucleus/cytoplasm of a few alveolar epithelial cells). Viral antigen was also observed in intestinal tissues in the nucleus/cytoplasm of pancreatic duct and nucleus/cytoplasm of colonic gland cells of #LE01-02 and #NH02.
Figure 2. Representative histopathological findings of the cerebrum in #LE02 (A) and heart in #LE01 (B); arrow heads indicate areas that degenerated to necrotic foci with mild inflammatory reactions. Immunohistochemical demonstration of type A influenza virus antigens in the cerebrum in #LE02 (C) and heart of #LE01 (D) [which is almost consistent with the lesions in #LE02 (A) and #LE01 (B), respectively], lung in #NH02 (E), and colon in #LE02 (F). Bars indicate 100 µm.
![Figure 2. Representative histopathological findings of the cerebrum in #LE02 (A) and heart in #LE01 (B); arrow heads indicate areas that degenerated to necrotic foci with mild inflammatory reactions. Immunohistochemical demonstration of type A influenza virus antigens in the cerebrum in #LE02 (C) and heart of #LE01 (D) [which is almost consistent with the lesions in #LE02 (A) and #LE01 (B), respectively], lung in #NH02 (E), and colon in #LE02 (F). Bars indicate 100 µm.](/cms/asset/9673eafd-3658-4152-a119-2ead5cd8be42/cavp_a_2022599_f0002_oc.jpg)
Discussion
The present study revealed that herons are not only susceptible to clade 2.3.2.1 H5N1 HPAIV but can also suffer from lethal infections. In particular, viruses propagating in the brains of dead herons were probably involved in this lethal effect. Two grey herons with HPAIV showed lethargy and conjunctivitis. As conjunctival hyperaemia could be easily recognised, ocular symptoms could be a valid indicator of infected wild/captive herons with HPAIVs. All surviving grey herons seroconverted, while two of the three black-crowned night herons were not infected with HPAIV. Susceptibility of herons to HPAIVs is likely to vary depending on the heron species. Several infection cases have been reported in grey herons in the field, suggesting that this species is highly susceptible to HPAIVs. Viruses were isolated from the brain and lungs of the dead grey heron in this study, in accordance with previous cases in Hong Kong (Ellis et al., Citation2004). Seroconversion was also observed in the contact grey herons. The low HI titres observed at 10 dpi (2–4 HI) suggest that they acquired the infection after the challenged grey herons. Oral shedding of virus was probably involved in the viral transmission because the challenged grey herons shed limited amounts of viruses via the cloaca. We speculate that regurgitating behaviour contributed to the viral transmission; during the infection experiment, some herons occasionally ate the fed jack mackerel that other individuals regurgitated. In this way, viruses were mechanically transmitted from herons, such as #GH04, with viruses in the oral cavity and oesophagus via feed. This hypothesis is likely supported by the finding that higher virus titres were found in the digestive tracts (cloacal swabs) of the contact birds. In HPAI-affected geographic locations, virus-susceptible birds, such as Anseriformes, are likely to shed virus into environmental water via the oral and cloacal routes, and HPAIV was detected in some water samples (Usui et al., Citation2020), suggesting that herons may become infected with HPAIV by preying on fish in water. Subclinical infection was observed in grey herons, intermediate egret, and black-crowned night herons, indicating that serological surveillance could be effective in investigating HPAIV prevalence in heron flocks.
Two little egrets showed apparent symptoms, such as lethargy at 4 dpi, and died the next day. Sudden death of little egrets was also reported in Penfold Park, Hong Kong in 2002; the birds showed physical inactivity and depression in the morning and died by evening (Ellis et al., Citation2004). Viruses were detected in the lungs and oral/cloacal swabs of infected little egrets in previous field cases (Ellis et al., Citation2004) and the present study. Continuous virus shedding via the oral route was observed in #LE01 and #LE02, starting 1 dpi. Efficient virus replication is likely to have contributed to dissemination of the virus from the lower respiratory tract to the oral cavity. The large number of deaths in little egret flocks in Hong Kong might have been caused by dissemination of the rapidly shed viruses to their roost. Interestingly, viruses were isolated from the breast muscles of little egrets and black-crowned night herons that died from HPAIV infections, in contrast to the dead grey heron. These results support the notion that HPAIV can be transmitted via consumption of the carcasses of infected smaller heron species. To prevent HPAIV dissemination to scavengers, wild/captive herons suspected to be infected should be immediately incinerated or transported to appropriate biosecure facilities for definitive diagnosis.
In the present study, the herons were inoculated with the clade 2.3.2.1 H5N1 subtype M807 strain. A similar virus was isolated from a grey heron during the same season in Japan [A/grey heron/Oita/4402B069/2011 (H5N1)]. Clade 2.3.4.4 HPAIVs have recently circulated worldwide (Lee, Bertran, et al., Citation2017) and have been isolated from heron species, as described previously; they are likely to be susceptible to clade 2.3.4.4 HPAIVs as well as clade 2.3.2.1 virus, and further cases of infection and their involvement in the virus maintenance and dissemination are concerning. Although the present study had some limitations, such as the low number and unknown profiles (age and sex) of herons examined, we expect that the present findings will provide a basis for further pathological studies in herons and contribute to reducing the risk of future HPAI outbreaks in these species and other species sharing their habitat.
Acknowledgements
The capture and transportation of the herons was facilitated by the Yamashina Institute for Ornithology and Rhino LLC. We thank H. Takeuchi (Rhino LLC) for providing technical suggestions regarding the aviculture. We also thank S. Murakami (Hokkaido University) for technical support in the use of the BSL3 facility at the International Institute for Zoonosis Control, Hokkaido University. Rearing the captured herons at Tottori University was kindly supported by Y. Hashimoto, N. Murakami, T. Nishi, Y. Tanaka, and S. Toyoumi.
Disclosure statement
No potential conflict of interest was reported by the authors.
Additional information
Funding
References
- Ahmed, M.B., El-Shazly, M.M., El-Sanousi, A.A. & Youssef, I.Y. (2017). Isolation of H5 highly pathogenic avian influenza virus from cattle egret (Bubulcus ibis) near affected broiler chicken flocks in Egypt. Journal of Virological Sciences, 2, 62–70.
- Alexander, D.J. (1982). Ecological aspects of influenza A viruses in animals and their relationship to human influenza: a review. Journal of the Royal Society of Medicine, 75, 799–811.
- Astorga, R.J., Leon, L., Cubero, M.J., Arenas, A., Maldonado, A., Tarradas, M.C. & Perea, A. (1994). Avian influenza in wild waterfowl and shorebirds in the Donana National Park: serological survey using the enzyme-linked immunosorbent assay. Avian Pathology, 23, 339–344.
- Chen, H.X., Shen, H.G., Li, X.L., Zhou, J.Y., Hou, Y.Q., Guo, J.Q. & Hu, J.Q. (2006). Seroprevalance and identification of influenza A virus infection from migratory wild waterfowl in China (2004–2005). Journal of Veterinary Medicine. B: Infectious Diseases and Veterinary Public Health, 53, 166–170.
- Ellis, T.M., Bousfield, R.B., Bissett, L.A., Dyrting, K.C., Luk, G.S.M., Tsim, S.T., Sturm-Ramirez, K., Webster, R.G., Guan, Y. & Malik Peiris, J.S. (2004). Investigation of outbreaks of highly pathogenic H5N1 avian influenza in waterfowl and wild birds in Hong Kong in late 2002. Avian Pathology, 33, 492–505.
- Fink, M., Fernandez, S.R., Schobesberger, H. & Koefer, J. (2010). Geographical spread of highly pathogenic avian influenza virus H5N1 during the 2006 outbreak in Austria. Journal of Virology, 84, 5815–5823.
- Fouchier, R.A., Munster, V., Wallensten, A., Bestebroer, T.M., Herfst, S., Smith, D., Rimmelzwaan, G.F., Olsen, B. & Osterhaus, A.D. (2005). Characterization of a novel influenza A virus hemagglutinin subtype (H16) obtained from black-headed gulls. Journal of Virology, 79, 2814–2822.
- GISAID. (2021). Global Initiative on Sharing Avian Influenza Data database Retrieved 05-08-2021, from https://www.gisaid.org/.
- Hars, J., Ruette, S., Benmergui, M., Fouque, C., Fournier, J.Y., Legouge, A., Cherbonnel, M., Daniel, B., Dupuy, C. & Jestin, V. (2008). The epidemiology of the highly pathogenic H5N1 avian influenza in mute swan (Cygnus olor) and other anatidae in the Dombes region (France), 2006. Journal of Wildlife Diseases, 44, 811–823.
- Lee, D.H., Bertran, K., Kwon, J.H. & Swayne, D.E. (2017). Evolution, global spread, and pathogenicity of highly pathogenic avian influenza H5Nx clade 2.3.4.4. Journal of Veterinary Science, 18, 269–280.
- Lee, D.H., Sharshov, K., Swayne, D.E., Kurskaya, O., Sobolev, I., Kabilov, M., Alekseev, A., Irza, V. & Shestopalov, A. (2017). Novel reassortant clade 2.3.4.4 avian influenza A(H5N8) virus in wild aquatic birds, Russia, 2016. Emerging Infectious Diseases, 23, 359–360.
- Luo, K., Zhang, K., Liu, L., Shen, X., Jiao, P., Song, Y., Lv, J., Wang, M., Liu, Y., Qi, W., Ren, T., Irwin, D.M., Liao, M. & Shen, Y. (2018). The genetic and phylogenetic analysis of a highly pathogenic influenza A H5N6 virus from a heron, southern China, 2013. Infection, Genetics and Evolution, 59, 72–74.
- Martínez-Vilalta, A. & Motis, A. (1994). Family ARDEIDAE (HERONS). In D. Hoyo, A. Elliott & J. Sargatal (Eds.), Handbook of the Birds of the World Volume 1: Ostrich to Ducks (pp. 376–403). Barcelona: Lynx Edicions.
- OIE. (2021a). OIE Terrestrial Manual 2021: Chapter 3.3.4. Avian influenza (including infection with high pathogenicity avian influenza viruses). Retrieved 06-09-2021, from https://www.oie.int/fileadmin/Home/eng/Health_standards/tahm/3.03.04_AI.pdf.
- OIE. (2021b). Terrestrial Animal Health Code: Chapter 10.4. Infection with high pathogenicity avian influenza viruses. Retrieved 05-08-2021, from https://www.oie.int/fileadmin/Home/eng/Health_standards/tahc/current/chapitre_avian_influenza_viruses.pdf.
- Pohlmann, A., Starick, E., Grund, C., Hoper, D., Strebelow, G., Globig, A., Staubach, C., Conraths, F.J., Mettenleiter, T.C., Harder, T. & Beer, M. (2018). Swarm incursions of reassortants of highly pathogenic avian influenza virus strains H5N8 and H5N5, clade 2.3.4.4b, Germany, winter 2016/17. Scientific Reports, 8, 15.
- Reed, L.J. & Muench, H. (1938). A simple method of estimating fifty percent endpoints. American Journal of Epidemiology, 27, 493–497.
- Siengsanan-Lamont, J., Robertson, I., Blacksell, S.D., Ellis, T., Fenwick, S., Saengchoowong, S., Suwanpukdee, S., Yongyuttawichai, P., Sariya, L., Prompiram, P., Chaichoun, K., Wiriyarat, W., Pothieng, D. & Ratanakorn, P. (2011). Virological and molecular epidemiological investigations into the role of wild birds in the epidemiology of influenza A/H5N1 in central Thailand. Veterinary Microbiology, 148, 213–218.
- Smith, G.J., Vijaykrishna, D., Ellis, T.M., Dyrting, K.C., Leung, Y.H., Bahl, J., Wong, C.W., Kai, H., Chow, M.K., Duan, L., Chan, A.S., Zhang, L.J., Chen, H., Luk, G.S., Peiris, J.S. & Guan, Y. (2009). Characterization of avian influenza viruses A (H5N1) from wild birds, Hong Kong, 2004–2008. Emerging Infectious Diseases, 15, 402–407.
- Soda, K., Ito, H., Usui, T., Nagai, Y., Ozaki, H., Yamaguchi, T. & Ito, T. (2013). Incursion and spread of H5N1 highly pathogenic avian influenza viruses among wild birds in 2010-11 winter in Japan. Journal of Veterinary Medical Science, 75, 605–612.
- Soda, K., Usui, T., Uno, Y., Yoneda, K., Yamaguchi, T. & Ito, T. (2013). Pathogenicity of an H5N1 highly pathogenic avian influenza virus isolated in the 2010-2011 winter in Japan to mandarin ducks. Journal of Veterinary Medical Science, 75, 619–624.
- Soda, K., Tomioka, Y., Usui, T., Ozaki, H., Yamaguchi, T. & Ito, T. (2020). Pathogenicity of H5 highly pathogenic avian influenza virus in rooks (Corvus frugilegus). Avian Pathology, 49, 261–267.
- Soliman, A., Saad, M., Elassal, E., Amir, E., Plathonoff, C., Bahgat, V., El-Badry, M., Ahmed, L.S., Fouda, M., Gamaleldin, M., Mohamed, N.A., Salyer, S., Cornelius, C. & Barthel, R. (2012). Surveillance of avian influenza viruses in migratory birds in Egypt, 2003–09. Journal of Wildlife Diseases, 48, 669–675.
- Uno, Y., Soda, K., Tomioka, Y., Ito, T., Usui, T. & Yamaguchi, T. (2020). Pathogenicity of clade 2.3.2.1 H5N1 highly pathogenic avian influenza virus in American kestrel (Falco sparverius). Avian Pathology, 49, 515–525.
- Usui, T., Soda, K., Sumi, K., Ozaki, H., Tomioka, Y., Ito, H., Murase, T., Kawamoto, T., Miura, M., Komatsu, M., Imanishi, T., Kurobe, M., Ito, T. & Yamaguchi, T. (2020). Outbreaks of highly pathogenic avian influenza in zoo birds caused by HA clade 2.3.4.4 H5N6 subtype viruses in Japan in winter 2016. Transboundary and Emerging Diseases, 67, 686–697.
- Wang, G., Zhang, T., Li, X., Jiang, Z., Jiang, Q., Chen, Q., Tu, X., Chen, Z., Chang, J., Li, L. & Xu, B. (2014). Serological evidence of H7, H5 and H9 avian influenza virus co-infection among herons in a city park in Jiangxi, China. Scientific Reports, 4, 6345.
- Webster, R.G., Bean, W.J., Gorman, O.T., Chambers, T.M. & Kawaoka, Y. (1992). Evolution and ecology of influenza A viruses. Microbiological Reviews, 56, 152–179.
- WHO. (2002). WHO manual on animal influenza diagnosis and surveillance, from https://apps.who.int/iris/handle/10665/68026.
- WHO/OIE/FAO-Evolution-Working-Group. (2008). Toward a unified nomenclature system for highly pathogenic avian influenza virus (H5N1). Emerging Infectious Diseases, 14, e1.
- Woo, C., Kwon, J.H., Lee, D.H., Kim, Y., Lee, K., Jo, S.D., Son, K.D., Oem, J.K., Wang, S.J., Kim, Y., Shin, J., Song, C.S., Jheong, W. & Jeong, J. (2017). Novel reassortant clade 2.3.4.4 avian influenza A (H5N8) virus in a grey heron in South Korea in 2017. Archives of Virology, 162, 3887–3891.
- Xu, X., Subbarao, K., Cox, N.J. & Guo, Y. (1999). Genetic characterization of the pathogenic influenza A/Goose/Guangdong/1/96 (H5N1) virus: similarity of its hemagglutinin gene to those of H5N1 viruses from the 1997 outbreaks in Hong Kong. Virology, 261, 15–19.
- Yang, W., Schountz, T. & Ma, W. (2021). Bat influenza viruses: current status and perspective. Viruses, 13, 547.