ABSTRACT
Turkey reoviruses have been implicated in multiple disease syndromes resulting in significant economic losses to the turkey industry. It has been known for decades that turkey enteric reovirus (TERV) is involved in poult enteritis complex, but turkey arthritis reovirus (TARV), the causative agent of tenosynovitis in turkeys, emerged in 2011. In 2019, we isolated reovirus from several cases of hepatitis in turkeys and tentatively named it turkey hepatitis reovirus (THRV). The comparative pathogenesis of these viruses, and correlation with their genetic make-up (if any), is not known. In this study, we inoculated nine groups of 1-week-old turkey poults with two THRV, five TARV and two TERV via oral route. A tenth group served as a negative control. A subset of birds from each group was euthanised at 3, 5, 7, 14, 21, and 28 days post-inoculation (dpi). Tissues were collected for histology and real-time RT–PCR. All nine viruses were found to be enterotropic; the virus gene copy number in the intestine reached a peak at 5 dpi followed by a sharp decline at 7 dpi. All viruses caused a significant decline in body weight gain of birds as compared to the negative control group. Both TARV and THRV strains replicated in tendons and produced histologic lesions consistent with tenosynovitis. Hepatic lesions were produced by THRV only and the virus was re-isolated from liver and spleen of inoculated birds fulfilling Koch’s postulates. The results of this study should be helpful in facilitating diagnosis and designing future mitigation plans.
Introduction
Turkey reoviruses have been known for decades to be associated with poult enteritis complex, often in association with other enteric viruses (Jindal et al., Citation2009, Citation2010; Spackman et al., Citation2010; Mor, Sharafeldin, Abin et al., Citation2013). In 1980s, two reports described the isolation of turkey reovirus from lame turkeys (Levisohn et al., Citation1980; Page et al., Citation1982), after which no more field cases were observed until 2011, when turkey reovirus was isolated from tendons of 13-week-old turkeys with lameness (Mor, Sharafeldin, Porter et al., Citation2013). The isolated viruses were tentatively called turkey arthritis reovirus (TARV), while those isolated from cases of enteritis were labelled as turkey enteric reovirus (TERV). Inoculation of a TARV isolate into 1-week-old turkey poults produced tenosynovitis and lameness, while TERV did not (Sharafeldin et al., Citation2014, Sharafeldin, Mor, Bekele et al., Citation2015). Lameness was attributed to a decrease in the tensile strength and elasticity modulus of leg tendons (Sharafeldin et al., Citation2016). Both TARV and TERV replicated in the gastrointestinal tract while the former affected tendons and triggered significant innate antiviral (interferons alpha and beta) and T-helper-1 responses (Sharafeldin, Mor, Sobhy et al., Citation2015).
In 2019, we received several cases of turkeys (1-7 weeks old) with mortality approaching 5%. The dead birds exhibited multifocal hepatic and splenic necrosis. Reoviruses isolated from these cases have been tentatively named turkey hepatitis reovirus (THRV). Molecular characterisation of turkey reoviruses in our laboratory indicates that TARV has close homology with TERV, which suggests that TARVs may be a mutant of TERV (Mor et al., Citation2014). We further assume that THRV (reovirus associated with hepatic lesions in turkeys) may be a mutant of either TARV or TERV. This study was undertaken to compare the pathogenesis of turkey reoviruses associated with enteritis, arthritis and hepatitis and to establish Koch’s postulates for THRVs.
Materials and methods
Viruses
Nine different turkey reoviruses (two THRVs, five TARVs and two TERVs) isolated from diseased birds were selected based on the age of the affected birds, year of isolation, geographical location, severity of clinical disease (morbidity/mortality), gross and histologic lesions and molecular characterisation (). In this study, we used TARV-O’Neil strain as a positive control. TARV1 used in the present study was isolated in 2019 from a 16-week-old turkey showing severe clinical signs, gross and histologic lesions of arthritis. TARV2 was isolated from gastrocnemius tendons of a 4-week-old bird showing osteodystrophy and chondrodystrophy but no tenosynovitis. Isolate TARV3 has a unique sequence because it has some insertions and deletions in its S1 gene sequence. The TERV strains used (TERV1 and TERV2) in this study were isolated before 2010. We believe that these two TERV strains may be true enteric pathogens since reovirus-associated lameness was not reported until 2011. All viruses were propagated and titrated on Japanese quail fibrosarcoma (QT-35) cells. The method of Reed and Muench (Citation1938) was used to calculate 50% tissue culture infective dose (TCID50) of each virus.
Table 1. Details of virus isolates used in the study.
Experimental design
One-day-old turkey poults (n = 290) were divided into 10 groups of 29 poults each and were placed in 10 different air-filtered isolators. Food and water were supplied ad libitum. At 1 week of age, birds in groups 1–9 were inoculated with 0.3 ml of the respective virus (∼105 TCID50/ml) via the oral route (). Birds in group 10 (sham-inoculated control) were inoculated with virus-free DMEM. Birds were examined daily for overt clinical signs or mortality. Birds displaying signs of severe illness were euthanised according to the Institutional Animal Care and Use Committee (IACUC) protocol and guidelines from Research Animal Resources (RAR) at the University of Minnesota. Four birds from each group were euthanised at 3, 5, 7, 14, 21, and 28 days post-inoculation (dpi). At necropsy, gross lesions were noted followed by sample collection (duodenum, jejunum, ileocaecum, liver, spleen, heart, gastrocnemius tendon and bursa of Fabricius) for virus isolation, real-time RT–PCR, and histopathology. Body weights were noted at euthanasia before removing tissues from 14 dpi onwards.
Sample processing
For virus isolation and real-time RT–PCR (RT-qPCR), a 10% suspension was prepared by homogenising all tissue samples individually in Hank’s balanced salt solution (HBSS) for 1–2 min using a Stomacher (Model 80, Seward Ltd., Worthing, UK). Tissue homogenates were centrifuged at 1800 × g for 10 min at 4°C. The supernatant was decanted and frozen at −80°C until used.
Virus gene copy number
Universal avian reovirus real-time RT–PCR available at the Veterinary Diagnostic Laboratory, University of Minnesota (MVDL; https://www.vdl.umn.edu/node/15341) was used to calculate virus gene copy number in collected tissues. Briefly, viral RNA was extracted by using the MagMAX™ Pathogen RNA/DNA kit (Thermo Fisher Scientific, Waltham, MA, USA). Real-time RT–PCR was performed using AgPath-ID™ One-Step RT–PCR (Thermo Fisher Scientific), following the manufacturer’s instructions. Briefly, 25 µl of reaction mix contained 12.5 µl AgPath master mix, 1 µl of enzyme mix, 1 µl (10 µm/µl) of each primer, 1 µl (5 µm/µl) of each probe, 5 µl each of viral RNA, and nuclease-free water. The reaction conditions were 45°C for 10 min, 95°C for 10 min, and 40 cycles of 95°C for 15 s and 60°C for 45 s. Viral gene copy number was calculated using a standard curve of ten-fold dilutions of TARV-positive RNA included in all 96-well plates. The gene copy numbers of different tissues at different times were calculated and subjected to appropriate statistical analysis.
Virus isolation
Monolayers of QT-35 cells prepared in 24-well tissue culture plates were used for virus isolation. After removing cell culture fluid, the monolayers were washed thrice with sterile PBS, following which 0.5 ml of supernatant from each sample was inoculated in duplicate. The plates were incubated at 37°C under 5% CO2 for 1 h for virus adsorption, followed by the addition of maintenance medium (DMEM with 2% FBS and antibiotics) and incubation at 37°C under 5% CO2. The monolayers were examined daily for a period of 5–7 days for the appearance of virus-induced cytopathic effects (CPE). Two to three blind passages were given before calling a sample negative. Cell culture fluids were collected and subjected to RNA extraction and RT-qPCR as mentioned above.
Immunofluorescence
To confirm that observed CPE was induced by reovirus, the inoculated QT-35 cells were subjected to immunofluorescence assay. Briefly, the cell culture medium was removed from 24-well plates and the cells were scraped in 0.2 ml of PBS. The scraped cells were placed on a 12-well slide and air-dried. The cells were then fixed in acetone for 2 h followed by the addition of fluorescent tagged anti-ARV (avian reovirus) antibody (ID No. 680 VDL 9501, NVSL, Ames, IA, USA). The slide was placed in a humidified chamber at 37°C for 2 h, rinsed gently thrice with PBS, and counter-stained with Evans blue for 5–7 min. After mounting in 50% glycerol (pH 8.4), a coverslip was applied, and the slide was examined under a fluorescent microscope for the appearance of apple-green colour (positive for reovirus).
Histopathology
Formalin-fixed soft tissues were trimmed, processed, and stained with haematoxylin and eosin (H&E). Formalin-fixed hock joints were decalcified prior to processing for histopathological examination. Lesions in the gastrocnemius tendons were scored using a previously described histologic lesion scoring system (Sharafeldin et al., Citation2014).
Statistics
Two-way ANOVA and t-test were used to test the significance of variation in virus gene copy numbers in different tissues at different time points. A non-parametric Kruskal Wallis test followed by Mann–Whitney U test was used to test the significance of the difference in gastrocnemius tendon lesion scores. Statistical software NCSS2020 (https://www.ncss.com/) was used to conduct statistical analysis. The statistical significance was determined at P value < 0.05.
Results
Body weight
At 14 dpi, there was no statistically significant difference in the body weights of birds in any of the 10 groups. However, at 21 dpi, birds in the sham-inoculated group had statistically significant higher body weights as compared to the nine virus-inoculated groups. At 28 dpi, birds in the sham-inoculated group had statistically significant higher body weights than in groups inoculated with THRV1, THRV2, TARV1, TARV2, TARV3 and TERV2 but not with TARV4, TARV-O’Neil and TERV1 ().
Clinical disease and gross lesions
At all euthanasia time-points, no reovirus-specific clinical signs, mortality or gross lesions were observed in birds from any of the virus-inoculated groups except the THRV2-inoculated group in which one bird died at 9, 13 and 14 dpi each after showing mild weakness and lethargy. Another bird showing signs of severe clinical illness at 13 dpi was euthanised. At necropsy, these birds showed hepatosplenomegaly (n = 2) or splenomegaly (n = 2) with multifocal white necrotic foci in the hepatic parenchyma ((A)). At 21 dpi, some birds in this group showed splenomegaly with mottling (n = 2) and frothy caecal contents (n = 3) ((B)).
Virus gene copy number
All organs of the sham-inoculated group were negative by rRT-PCR. The virus gene copy number in the duodenum, jejunum and ileocaecum of THRV2-inoculated group peaked at 7 dpi, while in the other eight groups, this peak was seen at 5 dpi, and declined remarkably by 7 dpi. There were numerical but statistically non-significant differences among groups at various ages ((A–C)). Viral gene copy numbers in the bursa of Fabricius were not as high as those in intestinal segments. In the bursa, the virus gene copy numbers in THRV2 and TARV3 groups reached a peak at 5 dpi and were significantly higher than the other groups ((D)). In the spleen, significantly higher virus gene copy numbers were seen in the THRV2, TARV4 and TARV-O’Neil groups at 5 dpi than in other groups and at other time points ((A)). In the liver, THRV1, THRV2, TARV4 and TARV-O’Neil showed higher copy numbers than the other groups at 5 dpi. Peak virus gene copy numbers were reached at 7 dpi for THRV2 and at 14 dpi for THRV1 ((B)). In tendons, the THRV1, THRV2, TARV1, TARV4, and TARV-O’Neil groups had higher virus gene copy numbers than the other groups at 14, 21, and 28 dpi. Surprisingly, TERV2 also had a high virus gene copy number in tendons at 21 dpi ((A)).
Figure 3. Means of log10 of virus gene copy number in (A) duodenum, (B) jejunum, (C) ileocaecum and (D) bursa of Fabricius at 3, 5, and 7 days post-inoculation.
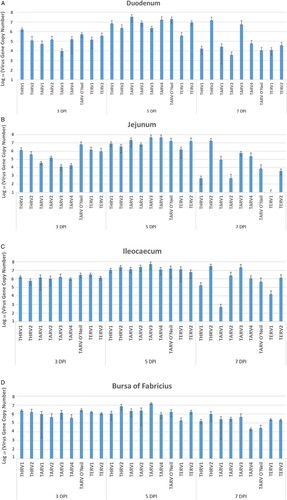
Histopathology of gastrocnemius tendons
Initial tendon lesions consisted of hypertrophy and hyperplasia of synoviocytes progressing to the formation of villous-like structures infiltrated with lymphoplasmacytic cells and later progressing to fibroplasia ((A)). Some sections showed prominent lymphoid aggregates in villous proliferation of synovium (villonodular hyperplasia) ((B)). Histologic lesion scores started at 7 dpi and increased at 14, 21 and 28 dpi in the THRV2 and all TARV groups, whereas THRV1 showed tendon lesions at 14 dpi. Birds inoculated with TARV1, TARV4, or TARV-O’Neil showed consistently high tendon lesion scores at 7, 14, 21, and 28 dpi, while TARV3 caused relatively lower tendon lesion scores at 7, 21, and 28 dpi. TARV2 caused tendon lesion scores only at 7 dpi. Of the two TERVs inoculated, only TERV2 showed tendon lesion scores at 21 and 28 dpi, but these scores were lower than those of most of the TARV and THRV inoculated groups ((B)).
Figure 6. Histologic lesions in tendon, liver and heart. (A) Villous nodular synovial hyperplasia: the villous nodules include hyperplastic synoviocytes, lymphocytic infiltration and fibrosis; (B) Villous nodular hyperplasia with germinal centre-like lymphoid aggregates; (C) Hepatocellular necrosis with mononuclear cell infiltration; (D) Lymphocytic epicarditis; (E) Direct fluorescent antibody test (DFA) of positive liver and spleen samples of group inoculated with THRV2: QT-35 cells are displaying green fluorescence indicating THRV2 replication.
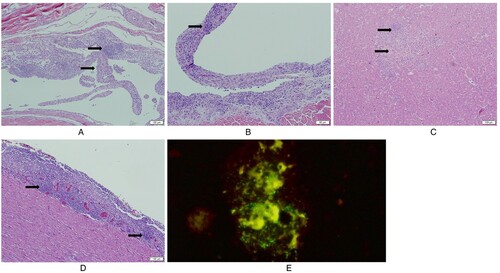
Histopathology of liver
No significant hepatic lesions were seen in any group except the THRV2 group. The birds in this group had extensive hepatic lesions at different time-points. At 5 dpi, three of four dead/euthanised birds had mild to moderate multifocal hepatocellular necrosis with infiltration of mononuclear cells ((C)). At 7 and 14 dpi, three of four and two of four birds, respectively, had moderate to severe hepatocellular necrosis. The virus was re-isolated from the liver and spleen tissue samples when inoculated into QT-35 cells and confirmed by immunofluorescence ((E)) and RT-qPCR.
Histopathology of heart
Only the TARV-O’Neil group showed consistent histologic lesions in the heart at different time-points. Two of the four birds had mild epicarditis (one to two small epicardial lymphoid aggregations) at 5 dpi, while three of four birds showed moderate (three to four small epicardial lymphoid aggregates) to severe (more than four epicardial lymphoid aggregates, focal extensive lymphoid infiltration, or circumferential lymphoid infiltration) epicarditis at 14 dpi. At 21 and 28 dpi, three of four and two of four birds, respectively, had mild epicarditis ((D)).
Histopathology of other organs
No significant microscopic lesions were observed in the intestinal segments and spleen of any of the virus-inoculated groups. All organs of the sham-inoculated control groups showed normal histoarchitecture.
Discussion
Turkey reovirus (TRV) has long been known to cause either enteritis or inapparent infections in turkeys. Since 2011, it has emerged as a causative agent of tenosynovitis, leading to lameness in turkeys. Recently, we have isolated TRV from several cases of turkey hepatitis. The reoviruses causing these disease conditions (enteritis, arthritis and hepatitis) are tentatively named as TERV, TARV, and THRV, respectively. This study was undertaken to compare the pathogenesis and tissue tropism of these viruses and to establish Koch’s postulates for THRVs. We selected certain unique reoviral isolates for this pathogenesis study. Previously, we have conducted studies on the pathogenesis of TARV by oral inoculation of the TARV-O’Neil strain in turkey poults (Sharafeldin et al., Citation2014, Sharafeldin, Mor, Bekele et al., Citation2015).
All three types of viruses showed very high replication rates and gene copy numbers in the intestine, which is not surprising since turkey reoviruses are generally enterotropic in nature. Significantly lower body weights in virus-inoculated birds were seen at 21 and 28 dpi but not at 14 dpi (). This is in agreement with Ngunjiri et al. (Citation2019), who observed that the difference in body weight of the virus-inoculated groups was non-significant at a very young age (< 2 weeks). The weight loss due to TERV and TARV has also been reported in several previously published reports (Spackman et al., Citation2005; Pantin-Jackwood et al., Citation2007; Sharafeldin, Mor, Bekele et al., Citation2015; Ngunjiri et al., Citation2019). The depression in the body weight of turkey reoviruses can be due to intrinsic factors of the strains and time post-infection (Spackman et al., Citation2005; Ngunjiri, et al. Citation2019). Although the viruses replicated in the intestinal segments and had an impact on body weight, they did not produce any significant histologic lesions. Previous studies have reported that TARVs and TERVs produce mild to severe enteritis with lymphocytic or heterophilic infiltration but not consistently (Spackman et al., Citation2005; Day et al., Citation2008; Sharafeldin et al., Citation2014). This may suggest that the virus can infect and replicate in the enterocytes but does not cause tissue damage in the absence of other factors such as co-infection with other pathogens, which needs to be explored further.
The gene copy numbers of TARV and TERV in intestinal segments reached a peak at 5 dpi followed by a sharp decline at 7 dpi (). This is in agreement with our previous study in which this decline was attributed to an elevation in antiviral interferon-alpha (IFN-A) and IFN-B at 7 dpi (Sharafeldin et al., 2015b). In contrast, the replication of THRV2 in the intestines reached a peak at 7 dpi, indicating that the predilection and pathogenic potential of THRV2 may be different from those of TARV and TERV. Studies are being planned to investigate the ability of THRV to escape or delay the immune response and cause disease.
In liver and spleen, the replication of THRV1, THRV2, TARV4 and TARV-O’Neil was observed at 5 dpi, reaching a peak at 7 dpi and decreasing thereafter. This observation further confirmed our previous results that these viruses cause viraemia after initial replication in the intestine and then are disseminated in different tissues (Sharafeldin et al., 2015b). The replication of only these four viruses in liver and spleen may be due to intrinsic viral characteristics and molecular or genetic factors that need further investigation.
Intestine and bursa are the primary sites of reovirus entry and replication, and the spleen is the first tissue infected after initial replication and viraemia (Pantin-Jackwood et al., Citation2007). Unlike chicken reovirus, the liver has not been shown to be the site of replication for turkey reoviruses (Pantin-Jackwood et al., Citation2007; Sharafeldin, Mor, Sobhy et al., Citation2015). This is probably the first study reporting the replication of turkey reovirus in the liver, indicating that these viruses are still evolving and changing in terms of their pathogenicity and tissue tropism. Thus, TRVs started out as being enteric pathogens causing mild to severe enteritis but later evolved to be arthrotropic (causing arthritis/tenosynovitis) and most recently to being hepatotropic (causing hepatitis).
The heart lesions (epicarditis) caused by TARV-O’Neil strain in this study is in contrast to previous results from our laboratory (Sharafeldin et al., Citation2014, Sharafeldin, Mor, Bekele et al., Citation2015). However, reovirus-induced heart lesions in turkeys have been reported previously (Spackman et al., Citation2005; Pantin-Jackwood et al., Citation2007; Shivaprasad et al., Citation2009; Davis et al., Citation2012). Our recent diagnostic findings indicate an increased lymphocytic myocarditis/epicarditis in TARV-positive turkey flocks (unpublished). It is worth mentioning that none of the other turkey reoviruses used in this study induced myocarditis/epicarditis. These findings indicate that the ability to induce myocarditis/epicarditis varies among different turkey reoviruses. Virus replication and gene copies were low in the heart of birds from all the virus-inoculated groups (data not shown).
Virus replication in tendons started at 7 dpi, reached a peak at 21 dpi, and declined at 28 dpi. Previous studies have also reported high rates of reovirus detection as early as 7 dpi in tendons of commercial turkeys (Sharafeldin, Mor, Sobhy et al., Citation2015; Ngunjiri et al., Citation2019). In tendons, appreciable viral gene copy numbers were observed in the THRV1, THRV2, TARV1, TARV4 and TARV-O’Neil groups. It is interesting to note that both strains of THRV migrated to and replicated in tendons. This finding correlates with the field situation where some flocks show hepatitis and mortality at a young age (2–5 weeks of age) followed by clinical lameness at 8–10 weeks of age. We believe that TARVs have mutated from TERVs that are ubiquitously present in turkeys, and the same progression may hold true for THRVs. Rapid genetic and antigenic divergence, vaccination pressure, and the inherent ability of reovirus to undergo reassortment and recombination might have resulted in the evolution of a different pathotype of TARV in the form of THRV. Further studies on genotyping and pathotyping are indicated.
This study, in conjunction with previous studies, confirmed that there are two main groups of avian reoviruses: highly pathogenic and low pathogenic in chickens and turkeys (Jones et al., Citation1984; Spackman et al., Citation2005; Sharafeldin et al., Citation2014; Ngunjiri et al., Citation2019). Reassortment may cause variations in the virulence and pathogenicity as reported by Ni and Kemp (Citation1995) for reovirus infection in broilers where a reassortant from mildly virulent and highly virulent strains behaved like mild strains in chickens. Hence, there is a possibility to determine pathotypes of reoviruses based on genomic characterisation if a complete genomic database of old and new strains in a particular farm is available.
Recently isolated TARV1 (in 2019) showed high replication in the intestine but low replication in tendons as compared to TARV-O’Neil (positive control). However, the histologic lesion score in tendons was as high as that of TARV-O’Neil, which suggests that TARV1 is also highly pathogenic. On the other hand, TARV2 isolated from a case of chondrodysplasia in 4-week-old birds showed ample replication in the intestine and bursa, but no replication and histologic lesion score in tendons (except at 7 dpi). This may suggest that this isolate either does not cause tenosynovitis or may do so at a higher dose or under conditions of stress or co-infection.
Two isolates (TARV3 and TERV1) had insertion and deletions in their S1 gene sequence and were divergent from previously reported turkey reoviruses. However, based on S1 gene sequence, they were similar to an enteric strain (NC/SEP-R44/03) which is known to cause moderate to severe bursal atrophy (Day et al., Citation2008). Although TARV3 showed higher replication and gene copy numbers in the intestine and bursa, it showed low replication in tendons and caused minimal to mild tenosynovitis as compared to other TARV strains. Although the S1 gene sequence of TERV1 was similar to that of TARV3, it showed different replication and tissue tropism. This is in contrast with duck reovirus strain HN5d, which has an 18 amino acid deletion in the sigma C (S1 gene) but was highly pathogenic in ducklings causing severe haemorrhage and necrosis of the liver with 20-30% mortality (Zheng et al., Citation2016).
Interestingly, both THRV strains produced severe histologic lesions in tendons but had consistently high viral gene copy numbers in the liver, indicating their predilection for the liver. It is important to note that THRV1 did not produce clinical disease and hepatic lesions while THRV2 did, although both viruses were originally isolated from cases of hepatitis. Factors responsible for this variation between THRV1 and THRV2 (and others) are not known at present.
Even TERV2 replicated in tendons at 21 dpi and showed histological lesions of tenosynovitis. However, the tendon lesion scores produced by TERV2 were significantly lower than those produced by TARVs. Histologic lesion scores above zero were observed in birds inoculated with enteric reovirus strain MN1 by Ngunjiri et al. (Citation2019) but were lower than the TARV-inoculated birds. High tenosynovitis lesion scores generally do not manifest into clinical lameness or gross pathological lesions, but we do not know if this virus would have produced frank lameness in older turkeys if the inoculated birds were kept for a longer duration. One enteric virus-inoculated bird developed severe valgus and lameness, suggesting enteric reovirus can cause arthritis in turkeys under stress conditions (Ngunjiri et al., Citation2019). Intrinsic characteristics of reovirus strains are responsible for different pathogenesis (Gouvea & Schnitzer, Citation1982) and the infectious dose may contribute to the pathotypic differences (Sharafeldin et al., Citation2014; Ngunjiri et al., Citation2019). Differential pathogenicity due to titre of turkey reoviruses used in this study was neutralised by inoculating a uniform amount of all the virus strains. This strongly proves that the observed pathogenicity is due to inherent and intrinsic viral characters. Additionally, it is possible that this 2008 TERV isolate constituted an evolutionary linkage with TARV that emerged in 2010.
In summary, this study demonstrated the pathogenicity of various turkey reoviruses when inoculated via the oral route. We conclude that all turkey reoviruses are enterotropic since they showed a very high replication rate in the intestines. We further believe that turkey reoviruses follow a generalised pathogenicity affecting different organs according to their predilection, the basis for which is not currently known. Only THRVs produced hepatic lesions and the virus was re-isolated from the liver and spleen of inoculated birds fulfilling Koch’s postulates.
Ethical statement
The experimental protocol was approved by the Institutional Animal Care and Use Committee (IACUC) of College of Veterinary Medicine, University of Minnesota, USA.
Supplemental Material
Download MS Excel (13.6 KB)Acknowledgements
The authors thank Drs Asif Idrees and Zumara Younas for technical help in the experimental study.
Disclosure statement
No potential conflict of interest was reported by the authors.
Additional information
Funding
References
- Davis, J.F., Kulkarni, A. & Fletcher, O. (2012). Myocarditis in 9-and 11-day-old broiler breeder chicks associated with a reovirus infection. Avian Diseases, 56, 786–790.
- Day, J.M., Spackman, E. & Pantin-Jackwood, M.J. (2008). Turkey origin reovirus-induced immune dysfunction in specific pathogen free and commercial turkey poults. Avian Diseases, 52, 387–391.
- Gouvea, V. & Schnitzer, T.J. (1982). Pathogenicity of avian reoviruses: examination of six isolates and a vaccine strain. Infection and Immunity, 38, 731–738.
- Jindal, N., Patnayak, D.P., Chander, Y., Ziegler, A.F. & Goyal, S.M. (2010). Detection and molecular characterization of enteric viruses from poult enteritis syndrome in turkeys. Poultry Science, 89, 217–226.
- Jindal, N., Patnayak, D.P., Ziegler, A.F., Lago, A. & Goyal, S.M. (2009). Duration of growth depression and pathogen shedding in experimentally reproduced poult enteritis syndrome. Avian Diseases, 53, 517–522.
- Jones, R.C. & Guneratne, J.R.M. (1984). The pathogenicity of some avian reoviruses with particular reference to tenosynovitis. Avian Pathology, 13, 173–189.
- Levisohn, S., Gur-Lavie, A. & Weisman, J. (1980). Infectious synovitis in turkeys: isolation of tenosynovitis virus-like agent. Avian Pathology, 9, 1–4.
- Mor, S.K., Sharafeldin, T.A., Abin, M., Kromm, M., Porter, R.E., Goyal, S.M. & Patnayak, D.P. (2013). The occurrence of enteric viruses in light turkey syndrome. Avian Pathology, 42, 497–501.
- Mor, S.K., Sharafeldin, T.A., Porter, R.E., Ziegler, A., Patnayak, D.P. & Goyal, S.M. (2013). Isolation and characterization of a turkey arthritis reovirus. Avian Diseases, 57, 97–103.
- Mor, S.K., Verma, H., Sharafeldin, T.A., Porter, R.E., Jindal, N., Ziegler, A. & Goyal, S.M. (2014). Characterization of S class gene segments of a newly isolated turkey arthritis reovirus. Virology, 464, 33–44.
- Ngunjiri, J.M., Ghorbani, A., Jang, H., Waliullah, S., Elaish, M., Abundo, M.C., Mahesh, K.C., Taylor, K.J., Porter, R. & Lee, C.W. (2019). Specific-pathogen-free turkey model for reoviral arthritis. Veterinary Microbiology, 235, 170–179.
- Ni, Y. & Kemp, M.C. (1995). A comparative study of avian reovirus pathogenicity: virus spread and replication and induction of lesions. Avian Diseases, 39, 554–566.
- Page, R.K., Fletcher Jr, O.J. & Villegas, P. (1982). Infectious tenosynovitis in young turkeys. Avian Diseases, 26, 924–927.
- Pantin-Jackwood, M.J., Spackman, E. & Day, J.M. (2007). Pathology and virus tissue distribution of turkey origin reoviruses in experimentally infected turkey poults. Veterinary Pathology, 44, 185–195.
- Reed, L.J. & Muench, H. (1938). A simple method of estimating fifty per cent endpoints. American Journal of Epidemiology, 27, 493–497.
- Sharafeldin, T.A., Chen, Q., Mor, S.K., Goyal, S.M. & Porter, R.E. (2016). Altered biomechanical properties of gastrocnemius tendons of turkeys infected with turkey arthritis reovirus. Veterinary Medicine International, 2016, 7829138. https://doi.org/10.1155/2016/7829138
- Sharafeldin, T.A., Mor, S.K., Bekele, A.Z., Verma, H., Goyal, S.M. & Porter, R.E. (2014). The role of avian reoviruses in turkey tenosynovitis/arthritis. Avian Pathology, 43, 371–378.
- Sharafeldin, T.A., Mor, S.K., Bekele, A.Z., Verma, H., Noll, S.L., Goyal, S.M. & Porter, R.E. (2015). Experimentally induced lameness in turkeys inoculated with a newly emergent turkey reovirus. Veterinary Research, 46, 1–7.
- Sharafeldin, T.A., Mor, S.K., Sobhy, N.M., Xing, Z., Reed, K.M., Goyal, S.M. Porter, R.E., & Roques P. (2015). A newly emergent turkey arthritis reovirus shows dominant enteric tropism and induces significantly elevated innate antiviral and T helper-1 cytokine responses. PLoS One, 10, e0144085.
- Shivaprasad, H.L., Franca, M., Woolcock, P.R., Nordhausen, R., Day, J.M. & Pantin-Jackwood, M. (2009). Myocarditis associated with reovirus in turkey poults. Avian Diseases, 53, 523–532.
- Spackman, E., Day, J.M. & Pantin-Jackwood, M.J. (2010). Astrovirus, reovirus, and rotavirus concomitant infection causes decreased weight gain in broad-breasted white poults. Avian Diseases, 54, 16–21.
- Spackman, E., Pantin-Jackwood, M., Michael Day, J. & Sellers, H. (2005). The pathogenesis of turkey origin reoviruses in turkeys and chickens. Avian Pathology, 34, 291–296.
- Zheng, X., Wang, D., Ning, K., Liang, T., Wang, M., Jiang, M. & Zhang, D. (2016). A duck reovirus variant with a unique deletion in the sigma C gene exhibiting high pathogenicity in Pekin ducklings. Virus Research, 215, 37–41.