Abstract
Advances in dating and systematics have prompted a revision of monotreme evolution to refine the timing of adaptative trends affecting body size and craniodental morphology. The oldest known monotreme, Teinolophos trusleri, is restricted to uppermost Barremian deposits of the Strzelecki Group in Victoria, Australia. Its body mass is estimated at ∼40 g, making it the smallest known monotreme. Teinolophos trusleri likely possessed an electro-sensitive and/or mechano-sensitive ‘bill’ or ‘beak’, which we suggest evolved for insectivory in seasonally dark Early Cretaceous polar forests. During the early Albian–mid-Cenomanian, monotremes diversified in Australia and evolved body masses greater than 4 kg, becoming amongst the largest Mesozoic mammals. A gap of 35 million years subsequently separates the youngest Mesozoic monotremes from the oldest Cenozoic monotreme, Monotrematum sudamericanum, which is a Paleocene stem ornithorhynchid from southern South America. We also hypothesize that tachyglossids originated in Melanesia, perhaps on the emergent Vogelkop landmass, and then dispersed to Australia during the Pliocene-Pleistocene. Finally, we present a classification of Monotremata to include five families—Teinolophidae fam. nov., Kollikodontidae, Steropodontidae, Ornithorhynchidae, and Tachyglossidae. We also propose a new genus, Murrayglossus gen. nov. for a gigantic Pleistocene echidna from southwestern Western Australia.
http://zoobank.org/urn:lsid:zoobank.org:pub:E39E2644-DADD-49F4-B2B7-47A25C072B07
Timothy F. Flannery [[email protected]], Kristofer M. Helgen [[email protected]], Australian Museum, 1 William St Sydney 2000, Australia; Thomas H. Rich [[email protected]], Tim Ziegler [[email protected]], Museums Victoria, PO Box 666, Melbourne, Victoria 3001, Australia; Patricia Vickers-Rich [[email protected]; [email protected]], School of Earth, Atmosphere and Environment, Monash University, Victoria 3800, Australia; Swinburne University of Science and Technology, Department of Chemistry and Biotechnology, Hawthorn, Victoria 3122, Australia; Elizabeth Grace Veatch [[email protected]], National Museum of Natural History, Smithsonian Institution, Washington, DC 20013, USA.
LIVING monotremes (Monotremata) are the only surviving egg-laying mammals. With the exception of the Patagonian Paleocene Monotrematum sudamericanum Pascual, Archer, Jaureguizar, Prado, Godthelp & Hand, Citation1992 (Pascual et al. Citation1992), all living and fossil monotremes are restricted to Australia and New Guinea (). The five living species are placed in two families: Ornithorhynchidae, with a single living species, Ornithorhynchus anatinus (Shaw, Citation1799); and Tachyglossidae, which includes the short-beaked echidna, Tachyglossus aculeatus (Shaw, Citation1792), and the long-beaked echidnas, Zaglossus Gill, Citation1877. The long-beaked echidnas include three living species: Zaglossus bruijnii (Peters & Doria, Citation1876); Zaglossus bartoni (Thomas, Citation1907); and Zaglossus attenboroughi Flannery & Groves, Citation1998.
Darlington (Citation1957) advanced the earliest published hypothesis concerning monotreme origins and diversification, suggesting that living platypus and echidnas are the remnants of a much wider radiation that might have begun in the Jurassic. However, recent studies have not supported Darlington’s (Citation1957) proposal of a Jurassic origin for onithorhynchids and tachyglossids. For example, a holistic revision by Phillips et al. (Citation2009) concluded that tachyglossids likely developed from platypus-like ancestors ∼19–48 Ma (million years ago), with 95% confidence limits of ∼13.3–73.1 Ma. Phillips (Citation2015) presented another divergence estimate of ∼19.8–33.6 Ma, but included fossil onithorhynchids of the genus Obdurodon Woodburne & Tedford, Citation1975 as crown monotremes. Pian et al. (Citation2016) disputed this claim and argued that placing the species of Obdurodon as crown monotremes affected the minimum bounds given by Phillips (Citation2015) for the tachyglossid–ornithorhynchid split. Other molecular approaches have yielded broadly concordant estimates: a lactalbumin-based study (Messer et al. Citation1998) suggested divergence at ∼50–57 Ma, while a recent genomic analysis at chromosome scale (Zhou et al. Citation2021) suggested a divergence time of ∼55 Ma, with 95% confidence limits of ∼35–85 Ma. In a broad molecular phylogenomic overview of mammalian relationships, Meredith et al. (2011) gave a range of ∼22.4–103.1 Ma. Divergence times derived from earlier studies based on mitochondrial sequences and smaller genomic datasets by Retief et al. (Citation1993) and Belov & Hellman (Citation2003) have alternatively derived ages of ∼22 Ma and ∼21 Ma, respectively. A further constraint on the poorly resolved divergence time of tachyglossids and ornithorhynchids is a molecular estimate for the two extant tachyglossid genera, Tachyglossus Illiger, 1811 and Zaglossus, estimated at ∼1.8–10.6 Ma (Phillips Citation2009). Tarver et al. (Citation2016) found that poor resolution of mammalian phylogenies results from inadequately fitted evolutionary models. Further work is therefore required to resolve this aspect of monotreme evolutionary biology.
Darlington’s (Citation1957) speculation that fossil monotremes would prove to be diverse and speciose has proved more accurate. Discoveries beginning with Archer et al. (Citation1985) have revealed a modestly rich Australian Cretaceous monotreme fauna. These include the unusually bunodont Kollikodon ritchiei Flannery, Archer, Rich & Jones, Citation1995 (Flannery et al. Citation1995), shrew-sized Teinolophos trusleri Rich, Vickers-Rich, Constantine, Flannery, Kool & van Klaveren, Citation1999 (Rich et al. Citation1999), and exceptionally large-toothed Stirtodon elizabethae Rich, Flannery & Vickers-Rich, Citation2020 (Rich, Flannery, & Vickers-Rich Citation2020), none of which survived the Mesozoic.
Darlington’s (Citation1957) Jurassic origin for monotremes was supported by Messer et al. (Citation1998), who placed the split between monotreme and therian mammals (metatherians and eutherians) at ∼163–186 Ma. Zhou et al. (Citation2021) analysed genomes at the chromosome scale to suggest that the divergence of monotremes and eutherians dates to ∼187 Ma. Meredith et al. (Citation2011), however, using a ‘molecular supermatrix’, indicated a Triassic divergence of ∼217.7 Ma with 95% confidence limits of ∼203–238.2 Ma. Most recently, a species-level phylogeny using a Bayesian molecular-clock dating approach estimated a divergence between Monotremata and Therian mammals at ∼200 Ma with 95% credibility intervals of ∼250–165 Ma (Álvarez-Carretero et al. Citation2021).
Most monotremes, including the geologically oldest Early Cretaceous (latest Barremian) taxon, T. trusleri, share several morphological features related to specialization of the anterior region of the jaws, which morphologically equates to the ‘bill’ or ‘beak’ of living monotremes (Rich et al. Citation2016). These include elongation of the region of the dentary anterior to the molars. and development of the dental canal reflecting the presence of an enlarged mandibular nerve associated with mechanoreception and/or electroreception (Rich et al. Citation2016). Most importantly, these key monotreme synapomorphies have not been identified in any other annectant taxa.
Monotremes were long classified within the subclass Prototheria, together with morganucodonts, docodonts, triconodonts and multituberculates (Kemp Citation1983). Prototheria is now recognized as a grade rather than a clade (Kemp Citation1983; although see Sereno Citation2006 and O’Leary et al. Citation2013 for dissenting views), prompting re-classification within a more inclusive Jurassic-Cretaceous Gondwanan infraclass Australosphenida, which also included the endemic Australian Ausktribosphenidae (Luo et al. Citation2001, Citation2002). Rougier et al. (Citation2007, p. 1) later proposed a new family, Henosferidae, for the South American Jurassic mammals Asfaltomylos patagonicus Rauhut, Martin, Ortiz-Jaureguizar & Puerta, Citation2002 and Henosferus molus Rougier, Martinelli, Forasiepi & Novacek, Citation2007, suggesting that: ‘Henosferidae was the sister group to Ambondro [Flynn, Parrish, Rakotosamimanana, Simpson & Wyss, Citation1999] from the Middle Jurassic of Madagascar, which, in agreement with previous phylogenies, was the sister taxon to the remaining australosphenidans’. Woodburne et al. (Citation2003) otherwise disputed the concept of Australosphenida, arguing that Ambondro mahabo Flynn, Parrish, Rakotosamimanana, Simpson & Wyss, Citation1999 was a basal eutherian and unrelated to monotremes. Analyses by Phillips et al. (Citation2009) also questioned this clade, interpreting monotremes as instead deriving from primitive triconodonts, such as the earliest Jurassic Hadrocodium wui Luo, Crompton & Sun, Citation2001.
The monotreme fossil record includes the three extant, and 11 extinct genera ranging from the Early Cretaceous to the Pleistocene and are distributed across Australia, New Guinea, and southern South America (). The phylogenetic position of some taxa is also disputed. In particular, Kryoryctes cadburyi Pridmore, Rich, Vickers-Rich & Gambaryan, Citation2005, which was based on a humerus and associated fragmentary premolar, is distinctly ‘echidna-like’ in morphology but has been questioned by Musser (Citation2013), albeit without supporting arguments. We alternatively follow Pridmore et al. (Citation2005) in recognizing K. cadburyi as a monotreme. However we disagree with the proposal by Rowe et al. (Citation2008) and Camens (Citation2010) that it may be a stem tachyglossid.
Table 1. The monotreme genera and their geological age range in millions of years (Ma).
Musser (Citation2013) also excluded K. ritchiei from Monotremata because it lacks an enlarged dentary canal. However, the discovery of the morphologically similar Sundrius ziegleri Rich, Flannery, Evans, White, Ziegler, Maguire, Poropat, Trusler & Vickers-Rich, Citation2020, led Rich, Flannery, Evans, et al. (Citation2020) to re-assess this relationship. Sundrius ziegleri is known from a single molar (probable M2) that possesses several monotreme synapomorphies. For example, the presence of broad anterior and posterior basal cingulae, as well as two narrowly converging crests on Cusp 4, prompted Rich, Flannery, Evans, et al. (Citation2020, p. 532) to suggest that ‘there are arresting similarities between [S. ziegleri] and the lingual region of the upper molar of Obdurodon sp. Although the morphological similarities are not strong in the buccal region, and there are differences in the development of cingula, no other mammalian molar morphology appears to be any more similar to [S. ziegleri].’ Thus the strongest evidence for affinity between S. ziegleri and K. ritchiei is the arcuate arrangement of the buccal cusps on the upper molars. Pian et al. (Citation2016, p. 102) described this feature as ‘unique [to K. ritchiei] among mammaliaforms known to date’. Its discovery in S. ziegleri (Rich, Flannery, Evans, et al. Citation2020) therefore provides compelling evidence for common ancestry.
Since the last published review of the monotremes (Musser Citation2013), two new fossil taxa, S. elizabethae and S. ziegleri, have been described (Rich, Flannery, Evans, et al. Citation2020, Rich, Flannery, & Vickers-Rich Citation2020). Moreover, the stratigraphical ages of many monotreme-bearing geological formations have been revised, bringing clarity to the timing and nature of monotreme evolutionary change. Here, we synthesize this information while also revising family and generic designations within the group.
Institutional abbreviations
AMNH, American Museum of Natural History, New York, NY, USA; AM (M = mammal collection; F = palaeontology collection), Australian Museum, Sydney, Australia; AOD, Australian Age of Dinosaurs Museum, Winton, Australia; MACN, Museo Argentino de Ciencias Naturales, Buenos Aires, Argentina; NMV, Museum Victoria, Melbourne, Australia; QM, Queensland Museum, Brisbane, Australia; SAM, South Australian Museum, Adelaide, Australia; WAM, Western Australian Museum, Perth, Australia; QVM, Queen Victoria Museum and Art Gallery, Launceston, Tasmania, Australia; MLP, Departamento Paleontología Vertebrados, Museo de La Plata, Argentina; MPEF−PV, Museo Paleontológico Egidio Feruglio, Paleontología Vertebrados, Trelew, Argentina.
Systematic palaeontology
MAMMALIA Linnaeus, Citation1758
MONOTREMATA Bonaparte, Citation1837 or 1838
Teinolophidae fam. nov.
()
Figure 2. Teinolophos, and early monotreme premolars. A, Reconstructions from synchrotron X-ray tomography of Teinolophos trusleri (NVM P229408): left dentary in lateral view (above) and a composite reconstruction of the left mandible and dentition (below) modified from Rich et al. (2016). B, Right upper premolars of: (I) Kollikodon ritchiei (AMF140201); (II and II’) occlusal and labial views (NMV P229194) of Teinolophos trusleri, respectively; (III and III’) occlusal and labial views (AM F118621) of Stirtodon elizabethae, respectively; (IV and IV’) occlusal and labial views of the P2 (QM F57736) of Obdurodon dicksoni respectively. Images modified from Rich, Flannery & Vickers-Rich (Citation2020).
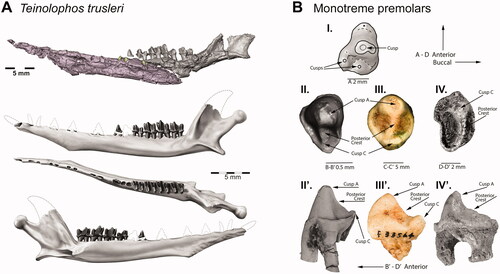
Type species
Teinolophos trusleri (see Rich et al. Citation1999).
Diagnosis
Differing from all other known monotremes in the possession of five molars rather than four or fewer, posterior premolar subequal in size to molars, and in small body-size (estimated at ∼40 g).
Remarks
Teinolophidae represents the oldest recognized family of monotremes followed chronologically by Kollikodontidae, Steropodontidae, Ornithorhynchidae, and Tachyglossidae. The currently only known representative of Teinolophidae, Teinolophos trusleri, is derived from the Flat Rocks locality, a coastal outcrop of the Lower Cretaceous (uppermost Barremian: Wagstaff et al. Citation2020) Strzelecki Group in southeastern Victoria, Australia (). The recently described monotreme Stirtodon elizabethae may also be referable to the Teinolophidae (; see Rich, Flannery, Evans, et al. Citation2020).
TACHYGLOSSIDAE Gill 1872
Murrayglossus gen. nov.
()
Figure 3. Postcrania of Murrayglossus hacketti. A, Left femur in anterior, posterior view. B, Left radius in anterior, posterior views. C, Pelvis in ventral, lateral and anterior views. D, Atlas in dorsal, ventral, anterior and posterior views. E, Clavicle in anterior, posterior views. Photographs courtesy of Geoff Deacon, Western Australian Museum.
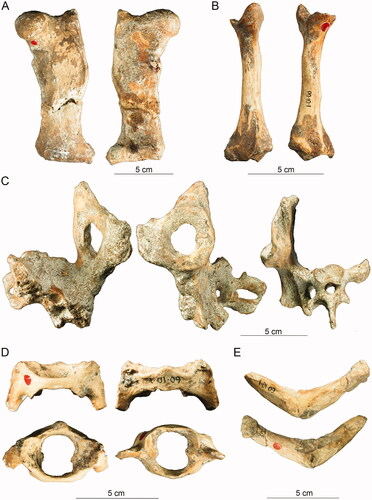
Figure 4. Comparisons of hind limb elements from Murrayglossus hacketti. A, Proportions of illustrated femurs and associated tibias in living and fossil tachyglossid genera modified from Murray (Citation1978b). From left to right; Tachyglossus, Zaglossus attenboroughi (AM 9852), Megalibgwilia oweni (Q.V.M. 13 or 1965:39:5), and Murrayglossus hacketti (WAM 60.10.1). B, Anterior aspect of the right femur (left; WAM 60.10.1), compared with Tachyglossus aculeatus ineptus (right) modified from Glauert (Citation1914) (scale not provided). C, Right tibia (WAM 60.10.1) showing the anterior, lateral, and posterior views modified from Glauert (Citation1914) (scale not provided).
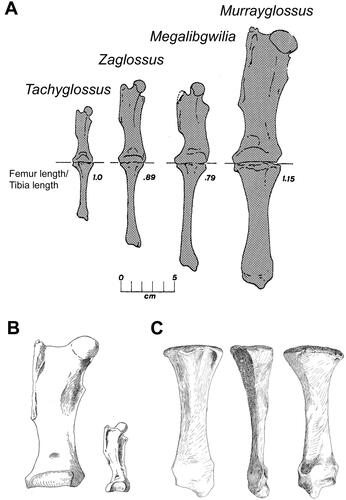
Type species
Murrayglossus hacketti (Glauert, Citation1914).
Diagnosis
Differing from all other tachyglossids in its gigantic size and unique suite of femoral characteristics: femoral head low; lesser trochanter very low relative to head and lying directly below the internal margin of the femur; greater trochanter large and high relative to the head; medial epicondyle flaring; condyles obliquely oriented (). The tibia is also short relative to the femur () with a femur/tibia ratio = 1.15. Comparative ratios: 0.92 (r = 0.89–0.96, n = 4) in Zaglossus; 1.01 (r = 0.98–1.04, n = 10) in Tachyglossus; 0.79 (n = 1) in Megalibgwilia Griffiths, Wells & Barrie, Citation1991.
Etymology
For Dr Peter Murray, who has done so much to elucidate the taxonomic status and morphology of extinct tachyglossids; and ‘glossus’, Greek for tongue.
Holotype
WAM 60.10.1, an associated partial skeleton including the atlas, clavicles and episternum, pelvic girdle, femora, a tibia and radius.
Type locality, unit and age
Strata excavated in 1909 (Glauert Citation1914) at Mammoth Cave, southwestern Western Australia (). The deposits are considered Pleistocene in age.
Remarks
Murray (Citation1978b) concluded that ‘Zaglossus’ hacketti was so distinctive that the species should be designated a separate genus—a proposal that we enact here. Murrayglossus hacketti is the largest known monotreme (Murray Citation1978b). A regionally coeval example of mammalian gigantism is the potoroid marsupial Borungaboodie hatcheri Prideaux, Citation1999 (Prideaux Citation1999), which is represented by a dentary from Tight Entrance Cave, and estimated at twice the body mass of the largest living potoroid (Prideaux Citation1999, Johnson Citation2006, Ayliffe et al. Citation2008). The highly endemic flora and fauna of southwestern Western Australia results from prolonged isolation (Laurie Citation2015), which may have led to ‘insular’ gigantism in B. hatcheri and M. hacketti.
The ecology of M. hacketti is unknown. Yet it is morphologically distinct from the sympatric Tachyglossus and Megalibgwilia, implying some degree of niche partitioning. Murray (Citation1978b, p. 53) commented that ‘one possible postural correlate of the shortened tibia and relatively long femur [in M. hacketti] is an adaptation to shift the centre of gravity of body mass backwards. This may have allowed mobility of the forelimbs for digging or tearing and may have permitted the animal to easily assume an assisted bipedal stance while feeding on ants or termites nests, a posture sometimes used by both living genera’. We would add that a semi-vertical posture might have also facilitated arboreality in M. hacketti. Surprisingly, Zaglossus bartoni is capable of ascending vertical fences (Flannery Citation1998). Furthermore, the vicinity of Mammoth Cave is densely forested. Arboreal-nesting termites (Calaby & Gay Citation1959) could have feasibly provided a viable food source. If correct, then niche separation among tachyglossids potentially involved Tachyglossus as a terrestrial feeder on colonial invertebrates, Megalibgwilia as a terrestrial feeder on large soil invertebrates (e.g., scarab beetle larvae: see Murray Citation1978b), and M. hacketti as a scansorial feeder on arboreal, colonial invertebrates.
Implications for monotreme evolution
Age of Mesozoic monotreme-bearing deposits
The oldest known monotreme, Teinolophos trusleri, is known exclusively from latest Barremian (Pilosisporites notensis Zone, ∼126 Ma: Wagstaff et al. Citation2020) Strzelecki Group deposits of the Flat Rocks locality in eastern Victoria (). The next oldest monotremes are Kryoryctes cadburyi from the Dinosaur Cove locality, and Sundrius ziegleri from Eric the Red West locality, both of which correlate with the lower Albian (Crybelosporites striatus Zone, ∼113–108 Ma: Wagstaff et al. Citation2020) Otway Group of western Victoria (). Kollikodon ritchiei, Steropodon galmani Archer et al. Citation1985, and Stirtodon elizabethae were all recovered from the Finch Clay Facies of the Wallangulla Sandstone (Griman Creek Formation: Rich, Flannery, & Vickers-Rich Citation2020). U-Pb age dating of detrital zircons extracted from a layer of volcanogenic claystone immediately overlying these fossil-bearing horizons have yielded a maximum depositional age-range of 100.2–96.6 Ma (Bell et al. Citation2019), or early to mid-Cenomanian.
Biases affecting the Mesozoic monotreme fossil record
Taphonomic impacts can certainly affect the composition of fossil assemblages. The key question, therefore, is whether taphonomy could be responsible for the absence of large-bodied monotremes in the Strzelecki Group. We consider this unlikely for the following reasons.
To date, 55 fossil mammal specimens have been recovered from the Strzelecki Group at the Flat Rocks locality (THR unpublished data). Of these 17 are dentaries ofTeinolophos trusleri (with or without teeth) attributable to five taxa: Ausktribosphenos nyktos Rich, Vickers-Rich, Constantine, Flannery, Kool & van Klaveren, 1997, Bishops whitmorei Rich et al. Citation2001, Kryoparvus gerriti Rich, Trusler, et al. Citation2020c, Corribaatar marywaltersae Rich, Vickers-Rich, Flannery, Kear, Cantrill, Komarower, Kool, Pickering, Trusler, Morton, van Klaveren & Fitzgerald, Citation2009, and Teinolophos trusleri. All 55 specimens represent small mammals, broadly similar in size to T. trusleri (Rich et al. Citation2009, Rich and Vickers-Rich Citation2004, Rich, Trusler, et al. Citation2020).
Over 2500 tetrapod fossils representing dinosaurs, turtles, temnospondyl amphibians, aquatic reptiles and pterosaurs have also been collected from the Strzelecki Group (Poropat et al. Citation2018), with over 80% being recovered from excavations at the Flat Rocks locality (THR unpublished data). Many of these represent animals with estimated body masses of ∼4–30 kg. By contrast, just six mammalian specimens have been recovered from the stratigraphically younger Otway Group strata (Rich, Flannery, Evans, et al. Citation2020). Of these, ∼50% are identifiable as B. whitmorei, which is similar in size to T. trusleri, while the remainder represent larger mammals (≥ 4 kg), including the large-bodied monotremes Kryoryctes cadburyi and Sundrius ziegleri (see below). The third—a partial premolar (NMV P208383) recovered from ‘The Pillar’ at the Slippery Rock locality—lay within 6 m of the holotype of K. cadburyi and could be referable to this taxon (Rich & Vickers-Rich Citation2004).
Excavations in the Otway Group have collected around 850 tetrapod fossils (THR unpublished data). More recently, the Eric the Red West locality has produced around 300 tetrapod fossils over six field seasons (THR unpublished data). The Otway Group has otherwise yielded around 1300 vertebrate body fossils (THR unpublished data), including various tetrapod specimens that overlap in estimated body mass with T. trusleri. Yet, no ‘Teinolophos-sized’ monotremes have ever been recovered.
The Strzelecki and Otway groups are dominated by stream and over-bank deposits that are rich in pebbly and clay interclast sediments (Poropat et al. Citation2018). It is difficult to imagine a taphonomic process that would exclude large monotremes from such facies in the Strzelecki Group, given that other vertebrates of equivalent size abound. We therefore consider the absence of such monotremes to reflect an original ecological signal.
Body mass estimates in fossil monotremes
The body mass of living and fossil monotremes spans four orders of magnitude. For example, Ornithorhynchus anatinus is the smallest extant monotreme, with body masses of ∼0.7–2.4 kg (Grant Citation1989). Tachyglossus aculeatus ranges from ∼2 to 7 kg (Rismiller & Grutzner Citation2019), while Zaglossus spp. range from ∼4 to 15 kg (Flannery Citation1995, Opiang Citation2009). Amongst the fossil taxa, the dentary of Teinolophos trusleri is ∼25 mm long with individual molar lengths of 1.1–1.7 mm (x̄ = 1.3 mm), and maximum widths of 0.8–1.5 mm (x̄ = 1.0 mm). Comparisons with the proportionately (and potentially ecologically) equivalent insectivorous marsupial, Antechinus adustus (Thomas, 1923), include male individual dentary lengths of ∼21 mm, and average upper molar lengths of ∼7.1 mm (Van Dyck & Crowther Citation2000). The average body mass of male A. adustus is ∼33.3 g (range = ∼30–42 g: Van Dyck & Crowther Citation2000), resulting in a reasonable body mass estimate for T. trusleri at ∼40 g.
Similarly, the humerus of Kryoryctes cadburyi approximates that of Tachyglossus aculeatus (Pridmore et al. Citation2005), which has a body mass of ∼3.5 kg (Rismiller & Grutzner Citation2019). The kollikodontids, Kollikodon ritchiei and Sundrius ziegleri, have upper molars of comparable dimensions (Rich, Flannery, Evans, et al. Citation2020), and might have had body masses of ∼4–8 kg (Weil Citation2005). The body mass of Steropodon galmani has not been estimated; however, its first lower molar is 6.6 mm long, which is comparable to Obdurodon insignis Woodburne & Tedford, Citation1975, with a molar length of 7.2 mm long and an estimated body mass of ∼2 kg (see below).
Stirtodon elizabethae is known from a single very large upper premolar (Rich, Flannery, & Vickers-Rich Citation2020), which is almost twice as long as the posterior upper premolar of Obdurodon dicksoni, but far larger than the premolar of any other documented monotreme (). A cautious estimate suggests that it was somewhat larger than Obdurodon tharalkooschild Pian, Archer & Hand, Citation2013; however, the premolars are smaller than the molars in most monotremes except for T. trusleri where they are of subequal length (Rich, Flannery, & Vickers-Rich Citation2020). If S. elizabethae conformed to this general trend, then its body mass may have been considerably larger.
The stem ornithorhynchid Monotrematum sudamericanum is based on two upper molars, one lower molar, and two partial femora (Pascual et al. Citation1992, Pascual et al. Citation2002, Forasiepi & Martinelli Citation2003). Our comparisons suggest that its body mass was ∼4 kg (). On the other hand, the species of Obdurodon (Woodburne & Tedford Citation1975, Archer et al. Citation1978, Pian et al. Citation2013, Asahara et al. Citation2016) are represented by cranial and dental remains, as well as an ilium with unfused acetabular sutures (SAM F11628) that was attributed to O. insignis by Archer et al. (Citation1978). Comparing this specimen with an adult O. anatinus individual with equivalent ilial dimensions and a recorded body weight of 1.25 kg (AM M48838) derives a body-mass estimate of ∼2 kg (see ).
Table 2. Distal femoral measurements for monotremes.
Table 3. Measurements (mm) of the ilium of Obdurodon insignis.
The m1 of Obdurodon () range from 7.2 mm in O. insignis, to 11.7 mm in O. tharalkooschild. Pian et al. (Citation2013) suggested that O. tharalkooschild was larger than M. sudamericanum, with a maximum body length exceeding 700 mm. The m1 of O. tharalkooschild is ∼50% longer than that of O. insignis, which has an estimated body mass of ∼2 kg. A simple conversion of length measurement to volume thus yields an estimated body-mass of ∼7 kg, which is consistent with Pian et al. (Citation2013).
The tachyglossids Megalibgwilia robusta (Dun, Citation1895) and Megalibgwilia owenii (Krefft, 1868) (formerly Megalibgwilia ramsayi Owen 1884: see Helgen et al. Citation2012), are known from putative Pliocene and Pleistocene deposits, respectively (Long et al. Citation2002). The material attributed to M. robusta includes an associated cranium, atlas and a humerus collected from a cave deposits near Gulgong (Dun Citation1895, Murray Citation1978b), while M. owenii is known from a partial associated skeleton along with referred material from various localities throughout New South Wales, Tasmania, Victoria, South Australia, and Western Australia (Murray Citation1978b, Griffiths et al. Citation1991, Turney et al. Citation2008, Prideaux et al. Citation2010). The published linear limb bone measurements of Megalibgwilia spp. overlap with those of Zaglossus spp., suggesting a similar body mass for both genera (Murray Citation1978b).
The largest recognized monotreme, Murrayglossus hacketti (Glauert Citation1914), probably reached about 1 m in maximum body length, but may have stood higher than other tachyglossids (Murray Citation1978b, Long et al. Citation2002). Skeletal dimensions suggest that it was likely 50% heavier than Zaglossus spp., perhaps with a body mass of ∼20–30 kg (Augee et al. Citation2006).
Monotreme origins
Luo et al. (Citation2001) proposed division of the subclass Holotheria (sensu Wible et al. Citation1995) into two infraclasses: Boreosphenida and Australosphenida. Boreosphenida consisted of the metatherians and eutherians, and supposedly originated in the Northern Hemisphere during the Mesozoic (Luo et al. Citation2001). Conversely, Australosphenida consisted of Southern Hemisphere Mesozoic tribosphenic mammals plus the monotremes (Luo et al. Citation2001).
In putting forth this hypothesis, Luo et al. (Citation2001) carried out a parsimony-based phylogenetic analysis of morphological data. At that time, the available data on Southern Hemisphere tribosphenic Mesozoic mammals was restricted to mandibles and lower dentitions (Rich, Flannery, Evans, et al. Citation2020). Luo et al. (Citation2001) therefore carried out two analyses: one restricted to 55 mandibular and lower dental characters; the other integrating 70 additional cranial and postcranial characters. In response, Sigogneau-Russell et al. (Citation2001) enumerated a number of dental states that were incongruous with the division of Boreosphenida and Australosphenida. Consequently, Woodburne et al. (Citation2003, Appendix C) and Woodburne (Citation2003) re-evaluated tribosphenic molar cusp terminology in monotreme teeth and found a clear separation of monotremes from tribosphenic mammals occurred in both the southern and northern hemispheres. Despite this, other papers have persistently utilized ever-expanding data matrices to support the Australosphenidan hypothesis (Luo et al. Citation2002, Citation2007, Citation2017, Kielan-Jaworwska et al. Citation2004, Rougier et al. Citation2007, Wible et al. Citation2009, Pian et al. Citation2016, Celik & Phillips Citation2020, King & Beck Citation2020). Further assessments of monotreme affinities have therefore not advanced significantly because the fundamental framework of their phylogeny remains essentially unchanged (Helgen Citation2003).
The discovery of Jurassic tribosphenic mammals in Madagascar (e.g., Ambondro mahabo: Flynn et al. Citation1999), South America (e.g., Asfaltomylos patagonicus and Henosferos molus: Rauhut et al. Citation2002, Rougier et al. Citation2007), and possibly Africa (e.g., Tendagurutherium dietrichi Heinrich, Citation1998), indicates a Gondwana-wide distribution of Mesozoic tribosphenic mammals. The Southern Hemisphere tribosphenic mammals subsequently appear to have become extinct (except in Australia) by the end of the Jurassic. No Jurassic mammals are presently known from Australia, but the presence of ausktribosphenids in the Early Cretaceous indicates that the lineage had persisted in eastern Gondwana for some time.
Fossils of Southern Hemisphere Mesozoic tribosphenic mammals consist mainly of lower dentitions and isolated teeth, with the exception of a single specimen comprising two damaged upper molars assigned to Bishops sp. (Rich, Flannery, Evans, et al. Citation2020). Unfortunately, the poor preservation of these teeth adds little to resolving ausktribosphenidan palaeobiogeography, but their evidence of a prominent protocone confirms that ausktribosphenids were truly tribosphenic (Rich, Flannery, Evans, et al. Citation2020).
In closing, we note that no ausktribosphenid possess the key monotreme synapomorphy of an elongated anterior dentary and enlarged dental canal (Rich et al. Citation2001, Rich, Trusler, et al. Citation2020c). Moreover, all known Southern Hemisphere tribosphenic mammals have three molars, whereas the most plesiomorphic monotreme, Teinolophos trusleri, is non-tribosphenic and has five molars (Rich et al. Citation2016). The requisite loss of tribosphenic molars and increase in molar number required to derive monotremes from a shared ancestor with ausktribosphenids is therefore, in our view, highly unlikely. Based on these considerations, we agree with Woodburne et al. (Citation2003) and Woodburne (Citation2003) in concluding that monotremes and ausktribosphenidans are diphyletic.
Monotreme classification
Teinolophos trusleri differs from all other monotremes in being at least an order of magnitude smaller in body mass, having five molars, and postdentary bones (= ear ossicles) that are in contact with Meckel’s cartilage but not the dentary (Rich et al. Citation2016). We subsequently consider this taxon to be deeply divergent within monotreme evolution, and thus designate a new family-level classification—Teinolophidae—to encompass it.
Rich, Flannery, Evans, et al. (Citation2020) regarded Sundrius ziegleri to be a close relative of Kollikodon ritchiei, but lacked some of its more extreme dental specializations having thin enamel, crests descending from cusp 4, and basal cingulae on the upper molars (). We classify both K. ritchiei and S. ziegleri within the family Kollikodontidae based on their shared possession of hypertrophied buccal cusps. Interestingly, Flannery et al. (Citation1995) suggested that the extremely thick enamel with basined dents exposing dentine on the cusp apices indicated that K. ritchiei probably fed on hard-shelled prey, such as molluscs. Sundrius ziegleri lacked this feature and might not have been durophagous (Rich, Flannery, Evans, et al. Citation2020). Rich, Flannery, Evans, et al. (Citation2020) noted that S. ziegleri was stratigraphically equivalent to Kryoryctes cadburyi, which derived from a locality 12 km away. Kryoryctes cadburyi was based on a humerus () that implicated heavily built forelimbs and a fossorial lifestyle similar to modern echidnas (Pridmore et al. Citation2005). Nevertheless, a referred partial premolar is similar in size to the isolated molar of S. ziegleri which is based on a single upper molar, raising the question of whether K. cadburyi and S. ziegleri might be synonymous. Unfortunately, direct comparisons are not possible because of non-overlapping elements. Yet, the premolar of K. cadburyi has an elongate root, while the admittedly broken molar roots of S. ziegleri are shortened. We are therefore reluctant to advocate synonymy until more specimens are found.
Figure 5. Cretaceous monotremes. A, Holotype of Kollikodon ritchiei (AM F96602). Lateral views of the mandible with frontlit (I) and backlit (II) lighting. Occlusal views of the dentary (III) and maxilla (IV). B, Images of Sundrius ziegleri (NMV P252052) modified from Rich, Flannery, & Vickers-Rich (Citation2020) showing the M2 occlusal view in comparison with other genera modified from Musser (Citation2013) and Pian et al. (Citation2016). C, Holotype of Kryoryctes cadburyi (centre; NMV P208094) compared with humeri from Tachyglossus aculeatus and Ornithorhynchus anatinus modified from Pridmore et al. (Citation2005).
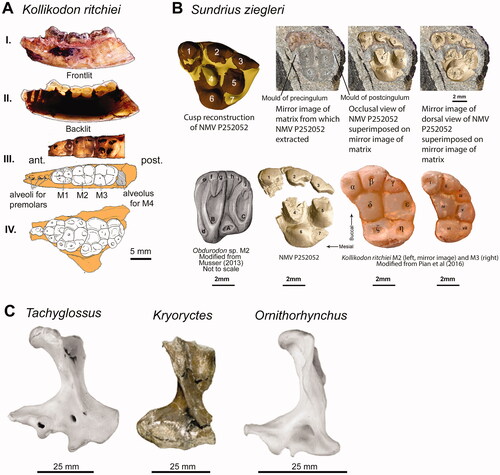
Multiple interpretations of kollikodontid relationships are possible. Musser (Citation2013) argued that the small mandibular canal of K. ritchiei evidenced non-monotreme affinities, but we consider this a product of its hypertrophied dentition, requiring deep molar roots and a robust dentary to resist the forces incurred by durophagous feeding. Alternatively, if Musser (Citation2013) is correct then the small mandibular canal of K. ritchiei could evince Kollikodontidae as the plesiomorphic sister lineage of Teinolophidae, Steropodontidae, Ornithorhynchidae, and Tachyglossidae. This arrangement would necessitate convergence in molar loss, with kollikodontids and all other monotremes except for teinolophids reducing their molar numbers from five to four, or fewer independently. An as-yet undetected ‘ghost lineage’ of kollikodontids would also necessarily pre-date the latest Barremian occurrence of teinolophids.
Whichever hypothesis is correct, it is clear that a reduction in molar number is a characteristic of monotreme evolution. For example, the stratigraphically oldest monotreme T. trusleri had five molars (Rich et al. Citation2016), while K. ritchiei had four (Flannery et al. 1993), Steropodon galmani three (Archer et al. Citation1985), and Obdurodon spp. and probably Monotrematum sudamericanum (see below) two upper and three lower molars (the posterior-most lower being rudimentary and single-rooted: Musser & Archer Citation1998). Ornithorhynchus anatinus has teeth only as a juvenile (one premolar and two upper molars, and three lower molars: see Latimer Citation2014), but these are replaced in later ontogeny by horny pads (). Conversely, all tachyglossids are edentulous (Musser Citation2013), with comparative genomic analyses further indicating that four of the eight key genes involved in mammalian tooth development have been lost in ornithorhynchids, tachyglossids, and probably their common ancestor (Zhou et al. Citation2021). The loss of functional teeth in O. anatinus and tachyglossids is nevertheless unlikely to be a synapomorphy, as O. anatinus retains a plesiomorphic vestigial dentition in early ontogeny.
The steropodontid S. galmani is ‘platypus-like’ in its reduction of lower molar number, but differs from all other monotremes in its superficially ‘tribosphenic-like’ molar morphology (Kielan-Jaworwska et al. Citation1987). Musser (Citation2013) argued that a flattened area of the dentary in S. galmani served as a facet for accessory jaw bones. Nevertheless, the only known specimen of this taxon is preserved as a solid opaline replacement with no discernible internal structure, which makes it difficult to determine if the flattened area is actually a plesiomorphic retained facet, or the result of diagenetic modification and/or post-mortem damage ().
Figure 6. Platypus-like monotremes. A, Dentary of Steropodon galmani (AM F66763) modified from Archer et al. (Citation1985). B, Stereopair occlusal view of the right M2 (above; MLP 91−I − 1 − 1 [holotype]) and right m1 (below; MPEF–PV 1635) of Monotrematum sudamericanum modified from Pascual et al. (Citation2002) (scale not provided). C, Lm1 (QM F56252, holotype) of Obdurodon tharalkooschild. 1–2, Stereopair, occlusal view; 3, anterior view; 4, buccal view; 5, lingual view. Image modified from Pian et al. (Citation2013, ). D, Distal end of the left femur of Monotrematum sudamericanum (MACN-Pv CH 1888) in lateral, dorsal, ventral, medial, and distal views modified from Forasiepi & Martinelli (Citation2003). E, Upper and lower premolars and molars of Obdurodon dicksoni and Obdurodon insignis (SAM P18087 [holotype] and AMNH 97228 [paratype]) modified from Archer et al. (Citation1992). F, Occlusal surfaces of the lower right toothrow of Ornithorhynchus anatinus. Image modified from Green (1937) (Scale not provided). G, Occlusal views of Lm1 (holotype cast, SAM P18087) and Lm2 (paratype, AMNH 97228) of Obdurodon insignis.
![Figure 6. Platypus-like monotremes. A, Dentary of Steropodon galmani (AM F66763) modified from Archer et al. (Citation1985). B, Stereopair occlusal view of the right M2 (above; MLP 91−I − 1 − 1 [holotype]) and right m1 (below; MPEF–PV 1635) of Monotrematum sudamericanum modified from Pascual et al. (Citation2002) (scale not provided). C, Lm1 (QM F56252, holotype) of Obdurodon tharalkooschild. 1–2, Stereopair, occlusal view; 3, anterior view; 4, buccal view; 5, lingual view. Image modified from Pian et al. (Citation2013, fig. 2). D, Distal end of the left femur of Monotrematum sudamericanum (MACN-Pv CH 1888) in lateral, dorsal, ventral, medial, and distal views modified from Forasiepi & Martinelli (Citation2003). E, Upper and lower premolars and molars of Obdurodon dicksoni and Obdurodon insignis (SAM P18087 [holotype] and AMNH 97228 [paratype]) modified from Archer et al. (Citation1992). F, Occlusal surfaces of the lower right toothrow of Ornithorhynchus anatinus. Image modified from Green (1937) (Scale not provided). G, Occlusal views of Lm1 (holotype cast, SAM P18087) and Lm2 (paratype, AMNH 97228) of Obdurodon insignis.](/cms/asset/b885206f-523d-4b1d-a6dd-4ed45c4117dd/talc_a_2025900_f0006_b.jpg)
Teinolophids and ornithorhynchids retain the plesiomorphic condition of parallel transverse crests on the molars (Rich et al. Citation2016). This state is unknown amongst other Mesozoic monotremes, suggesting that no presently defined lineage is ancestral to ornithorynchids. However, Musser (Citation2013) identified an edentulous partial mandible from the Finch Clay facies of the Griman Creek Formation, which is attributed to an undescribed steropodontid. This dentary is twisted laterally, as in O. anatinus, and could evidence a splayed bill (Musser Citation2013). We concur with this interpretation, but interpret the fossil as a probable new genus and species of stem ornithorhynchid.
Camens (Citation2010) and Musser (Citation2013) recognized M. sudamericanum as a stem ornithorhynchid. While the molar number of M. sudamericanum is unknown, there is no obvious wear facet from the third molar on M2 (: Pascual et al. Citation2002). Moreover, the molar morphology of M. sudamericanum is almost identical to that of Obdurodon spp., adding to the probability that M. sudamericanum had only two upper molars and possibly three in the lower jaw.
The toothed ornithorhynchid Obdurodon insignis from the upper Oligocene Etadunna Formation (), has been dated at ∼24–26 Ma using magnetostratigraphy and bio-correlation (Woodburne et al. Citation1994). In addition, Obdurodon dicksoni is known from a complete skull recovered at the lower Miocene Ringtail Site in the Riversleigh World Heritage Area, which has been radiometrically dated at ∼13.6 Ma from speleothems (Woodhead et al. Citation2016). Isolated ornithorhynchid teeth are also recorded from various lower Miocene (Faunal Zone B) and middle Miocene (Faunal Zone C) sites at Riversleigh (Pian et al. Citation2013). Of these, Obdurodon tharalskooschild () was named from a single tooth found at Two Trees Site. This cave deposit is of uncertain age (Archer et al. Citation1997) but has faunal similarities to the middle Miocene Kutjumarpu Local Fauna of Central Australia (Pian et al. Citation2013, Archer et al. Citation2018). Fossil elapid snakes and the potoroid marsupial Bettongia moyesi Flannery, 1987 have been cited to support an alternative Pliocene age (Archer et al. Citation1997, Scanlon et al. Citation2003); however, in our view, Two Trees Site cannot be dated with any certainty and contains an admixture of middle Miocene and Pliocene fossils that might be the result of redeposition. More robust stratigraphical and/or dating analyses are clearly required before the age of O. tharalkooschild can be determined.
The geologically oldest toothless ornithorhynchid is the Pliocene Ornithorhynchus agilis de Vis, 1886, which Archer et al. (Citation1978) and Bino et al. (Citation2019) regard as a junior synonym of O. anatinus. Speculatively, therefore, if O. tharalkooschild eventually proves to be Pliocene in age, then both toothed and toothless ornithorhynchids might have overlapped temporally. If this is the case, the genus Obdurodon might not be ancestral to modern Ornithorhynchus, as sometimes regarded, but alternatively represent a longer-lived lineage that is either closely related, or even paraphyletic relative to Ornithorhynchus. More precise molecular and geological dating is required to resolve this question.
Monotreme palaeozoogeography
The earliest known fossil monotremes are latest Barremian in age (), and coincide with the onset of rifting between Africa, India/Madagascar, and Australia/West Antarctica, as well as the establishment of a marked climatic gradient restricting dispersal from South America to Australia/East Antarctica across the West Antarctica archipelago, which persisted until the Turonian (∼93.9–89.8 Ma: Cantrill & Poole Citation2002). Tectonics and climate might have also delimited the distribution of other mammals, promoting the development of a unique Australian mammalian fauna that included endemic ausktribosphenids and one of the earliest cimolodontan multituberculates (Rich et al. Citation2009). Although monotremes subsequently migrated to southern South America (where they eventually became extinct), they were the most geographically restricted element of this distinctive Australian assemblage, as well as its only survivors.
Figure 7. Reconstruction of known temporal ranges for monotreme genera. Molar number and estimated maximum body weight are shown for each genus. Genetic divergence estimates for Monotremata and crown monotremes are also included. Family assignments for Ornithorhynchidae include both crown and stem taxa (e.g., Monotrematum); descendants of the earliest stem ornithorhynchids may also include the Tachyglossidae.
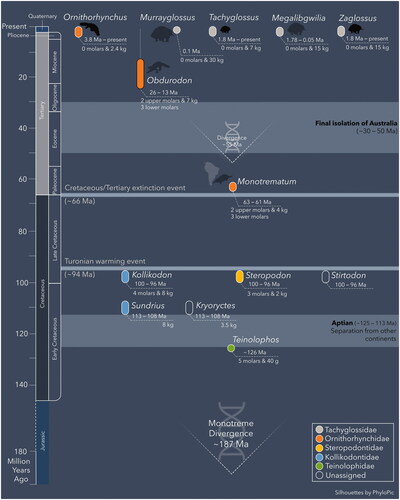
All extant monotremes possess a highly specialized ‘bill’ or ‘beak’ that plays a mechanoreceptive or electroreceptive role in feeding (Pettigrew Citation1999). A similar structure was apparently present in the earliest monotremes based on the mandibular morphology of Teinolophos trusleri (Rich et al. Citation2016). Southern Australia also lay within the polar circle throughout the Early Cretaceous (Poropat et al. Citation2018). Environmental reconstructions indicate a densely forested setting that experienced months of darkness each year and periodic snow cover during winter (Rich & Vickers-Rich Citation2000). Under such extreme conditions, probe feeding with a mechanoreceptive and electroreceptive rostrum would have been highly effective for obtaining food. We envisage that this adaptation evolved in direct conjunction with a polar environment, and accounts for the constrained distribution of early monotremes on the Australian landmass during the Early Cretaceous ().
A mechanoreceptive and/or electroreceptive ‘bill’ or ‘beak’ is also demonstrably useful for aquatic lifestyles, and may have appeared in the Cenomanian based on the undescribed ornithorychid-like dentary from the Finch Clay facies of the Griman Creek Formation (Musser Citation2013). Only aquatic stem monotremes seem to have survived the end-Cretaceous (K-Pg) mass extinction (), which may have in part been due to their freshwater habitats where bacterial decomposition of plant remains supported residual food chains, unlike oceanic and terrestrial photosynthesis which were profoundly interrupted (Robertson et al. Citation2013).
Several lines of evidence indicate that tachyglossids evolved from ‘platypus-like’ ancestors. Indeed, embryonic echidnas possess a marginal rostral cartilage, suggesting that their characteristic ‘beak’ originally developed from a ‘platypus-like’ ‘bill’ (Wilson Citation1901). Teinolophos trusleri likewise exhibited specializations indicative of an electroreceptive snout, implying that this feature could be a monotreme synapomorphy that was secondarily reduced in tachyglossids, and possibly kollikodontids (Rowe et al. Citation2008).
The earliest well-dated tachyglossid fossils derive from the early Pleistocene (between 1.77 Ma and 0.78 Ma: ) Nelson Bay Local Fauna in southwestern Victoria, which has yielded an ulna and some associated pedal elements of a Megalibgwilia-sized tachyglossid (Piper Citation2007). Other possible mid-Pleistocene or older tachyglossid fossils derive from the Canadian Deep Lead mine southeast of Gulgong, where the partial cranium (AM F51451), atlas (AM F51452) and humerus (AM F51453; originally described as the platypus, Ornithorhynchus maximus Dun, Citation1895) of Megalibgwilia robusta were recovered from gold-bearing gravel in a limestone cave (Dun Citation1895). Dun (Citation1895) also reported meiolaniid turtle, macropodid and bird remains from these deposits, which Murray (Citation1978b, p. 47) ‘believed to be Pliocene [in age] on the basis of the estimated age of a basalt flow overlying beds similar to those from which the fossils were recovered’. Nonetheless, some of these flows date from at least the Pleistocene (McAndrew Citation1965). Meiolaniids are thought to have been climatically delimited from southern Australia by the late Pleistocene (see Roberts et al. Citation2001); placing an upper limit on the age of the Canadian Deep Lead mine sediments during a warmer, wetter interval.
Three other eastern Australian localities have yielded remains of large tachyglossids: an ulna (AOD F0912) and radius (AOD F0913) from Glenrock, north of the Barrington Tops in eastern New South Wales; a humerus, scapula, interclavicle, partial femur and a claw from Wellington Caves in New South Wales; and a partial humerus and a claw from the Darling Downs in Queensland (Dun Citation1895). Both the Wellington Caves and Darling Downs have complex lithostratigraphical histories, incorporating both Pliocene and Pleistocene depositional phases (Osborne Citation1983, Wilkinson et al. Citation2021). Existing field exploration notes also record little beyond basic locality information. Hopefully more recent systematic excavations at Cathedral Cave in the Wellington Caves system (Fusco et al. Citation2019) can clarify at least some of these determinations in the near future.
Pleistocene strata in southern Australia have yielded a richer record of tachyglossids, (), including Murrayglossus hacketti whose unusual large size and postcranial morphology suggest that Quaternary monotremes occupied a broader range of ecologies than is currently suspected. Murray (Citation1978b) tentatively identified several gracile femora from Henschke’s Quarry Cave in South Australia, and Wellington Caves to the extant species Zaglossus bruijnii. Price & Webb (Citation2006) documented additional remains of Zaglossus sp. from the eastern Darling Downs (dated at ∼120–83 ka: Price et al. Citation2011, Price et al. Citation2019), and a tachyglossid ungual has been excavated from middle Pleistocene (∼330 ka) sediments at Mt Etna in Queensland (Hocknull Citation2009).
The extinct tachyglossid Megalibgwilia owenii had a wide distribution from near-coastal localities at Mammoth Cave in southwestern Western Australia (Glauert Citation1910), Henschke’s Quarry in the Naracoorte Caves system of South Australia (Murray Citation1978b, Pledge Citation1990), Strathdownie and other caves in southwestern Victoria (Gill Citation1957, Murray Citation1978b), and King Island (Scott & Lord Citation1921) and Montagu Cave in Tasmania (Murray Citation1978a).
Tachyglossus aculeatus is recognized from Pleistocene sediments in Mammoth Cave (Rismiller & Grutzner Citation2019), the Naracoorte Caves complex (Reed & Bourne Citation2009), McEachern’s Cave in Victoria (e.g., NMV P256461, NMV P256532-5, NMV P256568-9, NMV P256572-5), and the eastern Darling Downs (Price & Webb Citation2006). Tachyglossus aculeatus often co-occurs with M. owenii in the Bassian region (Griffiths et al. Citation1991).
The bones of tachyglossids are typically robust and frequently occur in Pleistocene caves, fluviatile deposits on the Darling Downs (Wilkinson Citation2021) and at Gulgong (Dun Citation1895), as well as paleosols at Nelson Bay (Piper Citation2016). The notable absence of tachyglossids in well-dated Oligocene, Miocene and Pliocene deposits in northern and central Australia (e.g., the Riversleigh World Heritage Area, Lake Eyre Basin, Frome Basin, Bullock Creek, and Alcoota: Rich Citation1991) thus requires explanation. One possibility is that tachyglossids arose during the mid-Miocene, but remained ‘platypus-like’ in morphology until the Pleistocene when they evolved into ‘echidna-like’ forms. We consider this scenario highly unlikely since it infers excessively rapid evolutionary rates. Alternatively, tachyglossids could have been restricted to environments not represented in the pre-Pleistocene Australian fossil record; yet, this is likewise difficult to envisage given the documented habitat diversity (e.g., rainforest in the Riversleigh World Heritage Area, to savannah at Alcoota: Archer et al. Citation1994, Woodburne Citation1967). Geographical isolation, however, offers a variant on this possibility. As a working hypothesis, we suggest that tachyglossids may have originated in Melanesia, and therefore remained absent from Australia prior to the later Neogene. The Vogelkop Peninsula or ‘Bird’s Head’ region of western New Guinea could provide possible ancestral areas within which stem ornithorhynchids inhabiting a tropical, rainforest-covered island would have had extensive areas of humid forest within which to forage. In the absence of competitors, specialization for a more terrestrial lifestyle could thus have feasibly evolved.
We find support for this scenario in the strong biogeographical links between Australia and the Vogelkop. While now endemic to the Vogelkop (Flannery Citation1995), the western long-beaked echidna (Zaglossus bruijnii) has been reported from Quaternary fossil deposits in southern Australia (Murray, Citation1978b), depicted in Pleistocene rock art from the Northern Territory (Helgen et al. Citation2012), and also persisted in the Kimberley region until the early twentieth century (Helgen et al. Citation2012). Moreover, the complex geological history of the Vogelkop (Troussaint et al. Citation2021) as an insular Cenozoic ‘micro-continent’ palaeogeographically proximal to northern Australia (Dam Citation1998, Hall Citation1996, Citation1998, Ratman Citation1998, Polhemus Citation2007) is broadly consistent with our proposal. The Vogelkop ‘central massif’ formed by the Arfak Mountains is composed of granitoids and highly metamorphosed rocks (Kleiber Citation1959); however, marine sediments deposited along its southern, eastern, and western margins indicate water depths exceeding 100 m during the Late Cretaceous. Foraminiferal assemblages otherwise demonstrate very shallow conditions (<10 m water depths) by the Paleocene, which may have exposed small islands. At least one substantial landmass was present during the early Oligocene, with most of what is now western New Guinea becoming fully subaerial from the mid-Oligocene to early Miocene (Gold et al. Citation2017). Some minor marine transgressions did occur during the mid-Pliocene (4–3.4 Ma), but substantial uplift has otherwise maintained terrestrial environments across New Guinea from the latest Miocene to Pleistocene (Gold et al. Citation2017).
The timing for establishment of land-bridge connections between the Vogelkop and Australian mainland remains uncertain (Hall Citation1996, Citation1998); although the presence of deeply divergent reptile (e.g., the crocodile skink, Tribolonotus Duméril & Bibron, 1839, pig-nosed turtle Carettochelys insculpta Ramsay, 1886) and endemic bird lineages (Cnemophilidae, Melanocharitidae, Paramythidae, Rhagologidae, Eulacestomidae, Ifritidae, Melampittidae: see Schodde & Christidis Citation2014, Aggerbeck et al. Citation2014) suggests that Melanesia maintained a separate diversification centre within the Sahul region. Subsequent faunal interchange from the late Miocene to Pleistocene coincides with the abrupt appearance of tachyglossids in the Australian fossil record, as well as the development of a distinctive rainforest-dwelling marsupial fauna that became widespread across eastern and northern Australia before extending to New Guinea (Flannery Citation1996). Key elements include ‘long-footed’ members of the genus Dendrolagus Müller, 1840, which are unique to Australia and the Vogelkop-North Coast Ranges (Flannery et al. Citation1996), as well as the pseudocheirid possum Petauroides Thomas, 1888, which is represented by the subfossil ‘Petauroides’ ayamaruensis Aplin, Citation1999 in the Vogelkop and is distributed in eastern Australia today (Eldridge et al. Citation2018). Tachyglossids could therefore have conceivably migrated to Australia from the Vogelkop at the same time as this northward interchange. Once in Australia, the group then radiated into diverse woodland, grassland and arid environments while simultaneously increasing emphasis on olfaction as a primary sensory adaptation for prey detection and capture (Ashwell Citation2013). Tachyglossus aculeatus has since become one of the most successful Australian-New Guinean mammals, achieving a wider distribution than any other endemic species (Augee Citation2008). Ashwell (Citation2013) suggested that the dispersal of T. aculeatus was augmented by the extinction of Pleistocene megafauna, which promoted its principal food source—termites—as a major ‘grazing’ influence on the Australian grasslands. By contrast, the Australian tachyglossid Megalibgwilia might have fed primarily upon insect larvae as suggested by its shorter, broader skull (Nicol Citation2021). Contraction of mesic habitats during the Late Pleistocene-Holocene could have depleted such soft-bodied prey, and together with human hunting, ultimately contributed to the extinction of the Megalibgwilia and Zaglossus species in Australia.
Conclusions
Monotreme evolution is marked by major adaptational and morphological trends affecting body size, mandibular morphology, and the number of cheek teeth.
A new family-level clade, Teinolophidae, is established for the oldest known monotreme, Teinolophos trusleri, which had an estimated body mass of ∼40 g, and likely possessed an electro-sensitive or mechano-sensitive ‘bill’ or ‘beak’ for feeding on insects in seasonally dark Early Cretaceous (latest Barremian) polar forests.
Monotreme diversifications during the early Albian to mid-Cenomanian coincided with marked body mass increase to >4 kg, and the emergence of the specialized kollikodontid and steropodontid lineages ().
By the Paleocene, stem ornithorhynchids had dispersed to southern South America via Antarctica, and subsequently speciated on the Australian landmass ().
The fossil record of tachyglossids extends only to the Pliocene or early Pleistocene, with the group potentially originating in Melanesia and subsequently dispersing to Australia.
Finally, we recognize a new tachyglossid genus, Murrayglossus, for a gigantic extinct Pleistocene echidna from southwestern Western Australia, and highlight the urgent need for protection of extant monotreme species, which are in decline because of human-induced habitat degradation (Helgen et al. Citation2012, Bino et al. Citation2019).
Acknowledgements
We thank the various curators and collection managers who provided access to specimens for our research. Sandy Ingleby (AM) provided access to extant monotreme material. Jared Diamond provided valuable insights into the zoogeography of Melanesia. Finally, the Chief Editor and Editorial Board of Alcheringa assisted with the compilation, submission and processing of our manuscript.
Disclosure statement
No potential conflict of interest was reported by the authors.
References
- Aggerbeck, M., Fjeldså, J., Christidis, L., Fabre, P.-H. & Jønsson, K.A., 2014. Resolving deep lineage divergences in core corvoid passerine birds supports a proto-Papuan island origin. Molecular Phylogenetics and Evolution 70, 272–285.
- Álvarez-Carretero, S., Tamuri, A., Battini, M., Nascimento, F., Carlisle, E., Asher, R., Yang, Z., Donoghue, P. & Dos Reis, M., 2021. A species-level timeline of mammal evolution integrating phylogenomic data. Nature. https://doi.org/https://doi.org/10.1038/s41586-021-04341-1
- Aplin, K.P., Pasveer, J.M. & Boles, W.E., 1999. Late Quaternary vertebrates from the Bird’s Head Peninsula, Irian Jaya, Indonesia, including descriptions of two previously unknown marsupial species. Records of the Western Australian Museum Supplement 57, 351–387.
- Archer, M., Binfield, P., Hand, S.J., Black, K.H., Creaser, P., Myers, T.J., Gillespie, A.K., Arena, D.A., Scanlon, J.D., Pledge, N.S. & Thurmer, J., 2018. Minimipossum notioplanetes, a Miocene forest-dwelling phalangeridan (Marsupialia: Diprotodontia) from northern and central Australia. Palaeontologica Electronica 21, 1–11.
- Archer, M., Flannery, T.F., Ritchie, A. & Molnar, R.E., 1985. First Mesozoic mammal from Australia — an early Cretaceous monotreme. Nature 318, 363–366.
- Archer, M., Godthelp, H. & Hand, S., 1994. Riversleigh: Story of Animals in Ancient Rainforest of Inland Australia. Australia: New Holland.
- Archer, M., Hand, S.J., Godthelp, H. & Creaser, P., 1997. Correlation of the Cainozoic sediments of the Riversleigh World Heritage fossil property, Queensland, Australia. In Actes du congrès BiochroM’97, Mémoires et Travaux de l’Ecole Pratique des Hautes Etudes. Aguilar, J.-P., Legendre S. & Michaux, J. eds., 21. Institut de Montpellier, Montpellier, 131–152.
- Archer, M., Jenkins, F.A., Jr, Hand, S.J., Murray, P. & Godthelp, H., 1992. Description of the skull and non-vestigial dentition of a Miocene platypus (Obdurodon dicksoni n. sp.) from Riversleigh, Australia, and the problem of monotreme origins. Platypus and echidnas. Royal Society of New South Wales, location is Mosman, NSW, Australia, 15–27.
- Archer, M., Plane, M. & Pledge, N., 1978. Additional evidence for interpreting the Miocene Obdurodon insignis Woodburne and Tedford, 1975, to be a fossil platypus (Ornithorhynchidae: Monotremata) and a reconsideration of the status of Ornithorhynchus agilis De Vis, 1885. Australian Zoologist 20, 9–27.
- Asahara, M., Koizumi, M., Macrini, T.E., Hand, S.J. & Archer, M., 2016. Comparative cranial morphology in living and extinct platypuses: feeding behavior, electroreception, and loss of teeth. Science Advances 2, e1601329.
- Ashwell, K.W.S., 2013. Reflections: monotreme neurobiology in context. In Neurobiology of Monotremes. Brain Evolution in Our Distant Mammalian Cousins. Ashwell, K., ed., CSIRO Publishing, Melbourne, 285–298.
- Augee, M., 2008. Short-beaked echidna. In The Mammals of Australia. Van Dyck, S. & Strahan, R., eds. Reed New Holland, Sydney, 37–39.
- Augee, M.L., Gooden, B. & Musser, A., 2006. Echidna: Extraordinary Egg-Laying Mammal. CSIRO Publishing, Sydney, 136 pp.
- Ayliffe, L.K., Prideaux, G.J., Bird, M.I., Grün, R., Roberts, R.G., Gully, G.A., Jones, R., Fifield, L.K. & Cresswell, R.G., 2008. Age constraints on Pleistocene megafauna at Tight Entrance Cave in southwestern Australia. Quaternary Science Reviews 27, 1784–1788.
- Bell, P.R., Fanti, F., Hart, L.J., Milan, L.A., Craven, S.J., Brougham, T. & Smith, E., 2019. Revised geology, age, and vertebrate diversity of the dinosaur-bearing Griman Creek Formation (Cenomanian), Lightning Ridge, New South Wales, Australia. Palaeogeography, Palaeoclimatology, Palaeoecology 514, 655–671.
- Belov, K. & Hellman, L., 2003. Platypus immunoglobin M and the divergence of the two extant monotreme lineages. Australian Mammalogy 25, 87–94.
- Bino, G., Kingsford, R.T., Archer, M., Connolly, J.H., Day, J., Dias, K., Goldney, D., Gongora, J., Grant, T., Griffiths, J., Hawke, T., Klamt, M., Lunney, D., Mijangos, L., Munks, S., Sherwin, W., Serena, M., Temple-Smith, P., Thomas, J., Williams, G. & Whittington, C., 2019. The platypus: evolutionary history, biology, and an uncertain future. Journal of Mammalogy 100, 308–327.
- Bonaparte, C.L.J.L., 1837 or 1838. Synopsis vertebratorums systematis. Nuovi annali delle scienze naturali. Bologna, 2, 105–133.
- Calaby, J.H. & Gay, F.J., 1959. Aspects of the distribution and ecology of Australian termites. In Biogeography and Ecology in Australia. Keast, A.,ed., Springer, Dordrecht, Netherlands.
- Camens, A.B., 2010. Were early Tertiary monotremes really all aquatic? Inferring paleobiology and phylogeny from a depauperate fossil record. Proceedings of the National Academy of Sciences USA 107, E12–E12.
- Cantrill, D.J. & Poole, I.P., 2002. Cretaceous patterns of floristic change in the Antarctic Peninsula. Geological Society of London Special Publication 194, 141–152.
- Celik, M.A. & Phillips, M.J., 2020. Conflict resolution for Mesozoic mammals: reconciling phylogenetic incongruence among anatomical regions. Frontiers in Genetics 11, 0651.
- Clarke, J.A., Tambussi, C.P., Noriega, J.I., Erickson, G.M. & Ketcham, R.A., 2005. Definitive fossil evidence for the extant avian radiation in the Cretaceous. Nature 433, 305–308.
- Dam, R.A.C., 1998. Cenozoic geological development and environmental settings of the Bird’s Head of Irian Jaya. In Perspectives on the Bird’s Head of Irian Jaya. Miedema, J., Odé, C. & Dam, R.A.C., eds, Rodopi, Amsterdam, 757–782.
- Dam, R.A.C., 1998. Yapen Island: a right-lateral paradox in the left-lateral ‘North New Guinea Megashear’. Implications for the biogeography and geological development of the Birds Head, Irian Jaya. In Perspectives on the Birds Head of Irian Jaya, Indonesia. Miedema, J., Odé, C. & Dam, R.A.C., eds, Rodopi, Amsterdam, 757–782, 982pp.
- Darlington, P.J., 1957. Zoogeography: The Geographical Distribution of Animals. John Wiley, London.
- Dun, W.S., 1895. Notes on the occurrence of monotreme remains in the Pliocene of New South Wales. Records of the Geological Survey of New South Wales 4, 118–126.
- Eldridge, M.D.B., Potter, S., Helgen, K.M., Sinaga, M.H., Aplin, K.P., Flannery, T.F. & Johnson, R., N., 2018. Phylogenetic analysis of the tree-kangaroos (Dendrolagus) reveals multiple divergent lineages within New Guinea. Molecular Phylogenetics and Evolution 127, 589–599.
- Flannery, T.F., 1995. Mammals of New Guinea. Reed Publishing, Sydney.
- Flannery, T.F., 1996. Mammalian zoogeography of New Guinea and the southwestern Pacific. In The Origin and Evolution of Pacific Island Biotas, New Guinea to Eastern Polynesia: Patterns and Processes. Keast, A. & Miller, S., eds, Academic Publishing, Amsterdam, 399–406.
- Flannery, T.F., 1998. Throwim Way Leg. Text Publishing, Melbourne.
- Flannery, T.F., Archer, M., Rich, T.H. & Jones, R., 1995. A new family of monotremes from the Cretaceous of Australia. Nature 377, 418–420.
- Flannery, T.F. & Groves, C.P., 1998. A revision of the genus Zaglossus (Monotremata, Tachyglossidae), with description of new species and subspecies. Mammalia 62, 367–396.
- Flannery, T.F., Martin, R. & Szalay, A., 1996. Tree Kangaroos: A Curious Natural History. Reed Publishing, Sydney.
- Flynn, J.J., Parrish, J.M., Rakotosamimanana, B., Simpson, W.F. & Wyss, A.R., 1999. A Middle Jurassic mammal from Madagascar. Nature 401, 57–60.
- Forasiepi, A.M. & Martinelli, A.G., 2003. Femur of a monotreme (Mammalia, Monotremata) from the Early Paleocene Salamanca Formation of Patagonia, Argentina. Ameghiana 40, 625–630.
- Fusco, D.A., Thorn, K.M., Shute, E.R., Tyler, M.J., Gibbs, N.R., Arnold, L.J., Sniderman, J., Worthy, T.H. & Prideaux, G.J., 2019. Mammalian responses to Late Quaternary environmental change in eastern Australia. Journal of Vertebrate Paleontology Program and Abstracts, 105.
- Gill, E.D., 1957. The ancestors of our marsupials. The Australian Amateur Mineralogist 3, 80–81.
- Gill, T., 1877. Vertebrate zoology. In: Baird, S.F. (Ed.), Annual Record of Science and Industry for 1876. Harper and Brothers, New York, pp. 171–172.
- Glauert, L., 1910. The Mammoth Cave. Records of the West Australian Museum 1, 9–30.
- Glauert, L., 1914. The Mammoth Cave (continued). Records of the Western Australian Museum and Art Gallery 1, 244–251.
- Gold, D.P., White, L.T., Gunawan, I., & Dagher-Fadel, B.O.U. & K, M., 2017. Relative sea level change in western New Guinea recorded by regional biostratigraphic data. Marine and Petroleum Geology 86, 1133–1158.
- Grant, T.R., 1989. Ornithorhynchidae. In Fauna of Australia Volume 1B. Walton, D.W. & Richardson, B.J., eds, Australian Government Publishing Service, Canberra, 1–28.
- Griffiths, M., Wells, R.T. & Barrie, D.J., 1991. Observations on the skulls of fossil and extant echidnas (Monotremata: Tachyglossidae). Australian Mammalogy 14, 87–101.
- Hall, R., 1996. Reconstructing Cenozoic SE Asia. In Tectonic Evolution of Southeast Asia. Hall, R. & Blundell, D.J., eds, The Geological Society, London, 153–184.
- Hall, R., 1998. The plate tectonics of Cenozoic SE Asia and the distribution of land and sea. In Biogeography and Geological Evolution of SE Asia. Hall, R. & Holloway, J. D., eds, Backhuys Publishers, Leiden, 99–132.
- Heinrich, W.D., 1998. Late Jurassic mammals from Tendaguru, Tanzania, East Africa. Journal of Mammalian Evolution 5, 269–290.
- Helgen, K.M., 2003. Major mammalian clades: a review under consideration of molecular and palaeontological evidence. Mammalian Biology 68, 1–15.
- Helgen, K.M., Portela Miguez, R., Kohen, J.L. & Helgen, L.E., 2012. Twentieth century occurrence of the long-beaked echidna Zaglossus bruijnii in the Kimberley region of Australia. ZooKeys 255, 103–132.
- Hocknull, S.A., 2009. Late Cainozoic Rainforest Vertebrates from Australopapua: Evolution, Biogeography and extinction. PhD thesis. University of New South Whales.
- Johnson, C., 2006. Australia’s Mammal Extinctions: A 50,000 Year History. Cambridge University Press, Melbourne, Australia, 310 pp.
- Kemp, T.S., 1983. The relationships of mammals. Zoological Journal of the Linnean Society 77, 353–384.
- Kielan-Jaworowska, Z., Cifelli, R.L. & Luo, Z.-X., 2004. Mammals from the Age of Dinosaurs: Origins, Evolution, and Structure. Columbia University Press, New York, 700 pp.
- Kielan-Jaworowska, Z., Crompton, A.W. & Jenkins, F.A., Jr., 1987. The origin of egg-laying mammals. Nature 326, 871–873.
- King, B. & Beck, R.M.D., 2020. Tip dating supports novel resolutions of controversial relationships among early mammals. Proceedings of the Royal Society B 287, 20200943.
- Kleiber, A.V., 1959. Geology of the Vogelkop Peninsula, Netherlands New Guinea. The 5th World Petroleum Congress, New York, USA, May 1959. Paper Number: WPC-8049.
- Latimer, A.E., 2014. Redescription of Teeth and Epithelial Plates from the Platypus Ornithorhynchus anatinus: Morphological and Evolutionary Implications. PhD thesis. University of Texas at Austin.
- Laurie, V., 2015. The Southwest: Australia’s Biodiversity Hotspot. UWA Publishing, Nedlands, WA, Australia. 240 pp.
- Linnaeus, C., 1758. Systema naturae per regia troa matirae. Secundum classes, ordines, genera, species cum characteribus, differentiis synonymis, locis. Editio decima, reformata. Stockholm, Laurenti Salvii 1, + 824 pp.
- Long, J., Archer, M., Flannery, T.F. & Hand, S., 2002. Prehistoric Mammals of Australia and New Guinea: One Hundred Million Years of Evolution. Johns Hopkins University Press, Baltimore, 45–47.
- Luo, Z.-X., 2007. Transformation and diversification in early mammalian evolution. Nature 450, 1011–1019.
- Luo, Z.-X., Chen, P., Li, G. & Chen, M., 2007. A new eutriconodont mammal and evolutionary development in early mammals. Nature 446, 288–293.
- Luo, Z.-X., Cifelli, R.L. & Kielan-Jaworowska, Z., 2001. Dual origin of tribosphenic mammals. Nature 409, 53–57.
- Luo, Z.-X., Kielan-Jaworowska, Z. & Cifelli, R.L., 2002. In quest for a phylogeny of Mesozoic mammals. Acta Palaeontologica Polonica 47, 1–78.
- Luo, Z.-X., Meng, Q.-J., Grossnickle, D.M., Liu, D., Neander, A.I., Zhang, Y.-G. & Ji, Q., 2017. New evidence for mammaliaform ear evolution and feeding adaptation in a Jurassic ecosystem. Nature 548, 326–329.
- McAndrew, J. (ed.), 1965. Geology of Australian Ore Deposits. Vol. I of the Eighth Commonwealth Mining and Metallurgical Congress, Australia and New Zealand. Melbourne.
- Meredith, R.W., Janecka, J.E., Gatesy, J., Ryder, O.A., Fisher, C.A., Teeling, E.C., Goodbla, A., Eizirik, E., Simao, T.L.L., Stadler, T., Rabosky, D.L., Honeycutt, R.L., Flynn, J.J., Ingram, C.M., Steiner, C., Williams, T.L., Robinson, T.J., Burk-Herrick, A., Westerman, M., Ayoub, N.A., Springer, M.S. & Murphy, M.J., 2011. Impacts of the Cretaceous Terrestrial Revolution and KPg extinction on mammal diversification. Science 334, 521–524.
- Messer, M., Weiss, A.S., Shaw, D.C. & Westerman, M., 1998. Evolution of the monotremes: phylogenetic relationship to marsupials and eutherians, and estimation of divergence dates based on α-lactalbumin amino acid sequences. Journal of Mammalian Evolution 5, 95–105.
- Murray, P.F., 1978a. A Pleistocene spiny anteater from Tasmania (Monotremata: Tachyglossidae, Zaglossus). Papers and Proceedings of the Royal Society of Tasmania 112, 39–68.
- Murray, P.F., 1978b. Late Cenozoic monotreme anteaters. Australian Zoologist 20, 29–55.
- Musser, A.M., 2003. Review of the monotreme fossil record and comparison of palaeontological and molecular data. Comparative Biochemistry and Physiology Part A: Molecular & Integrative Physiology 136, 927–942.
- Musser, A.M., 2013. Classification and evolution of the monotremes. In Neurobiology of Monotremes. Brain Evolution in Our Distant Mammalian Cousins. Ashwell, K., ed., CSIRO Publishing, Melbourne, 1–17.
- Musser, A.M. & Archer, M., 1998. New information about the skull and dentary of the Miocene ornithorhynchid relationships platypus Obdurodon dicksoni, and a discussion of ornithorhynchid relationships. Philosophical Transactions of the Royal Society of London. Series B: Biological Sciences 353, 1063–1079.
- Nicol, S.C., 2021. Diet, feeding behaviour and echidna beaks: a review of functional relationships within the tachyglossids. Australian Mammalogy 43, 39–50.
- O’Leary, M.A., Bloch, J.I., Flynn, J.J., Gaudin, T.J., Giallombardo, A., Giannini, N.P., Goldberg, S.L., Kraatz, B.P., Luo, Z.-X., Meng, J., Ni, X., Novacek, M.J., Perini, F.A., Randall, Z., Rougier, G.W., Sargis, E.J., Silcox, M.T., Simmons, N.B., Spaulding, M., Velazco, P.M., Weksler , Wible, M.J.R. & Cirranello, A.L., 2013. The placental mammal ancestor and the post-K-Pg radiation of placentals. Science 332, 662–667.
- Opiang, M.D., 2009. Home ranges, movement, and den use in long-beaked echidnas, Zaglossus bartoni, from Papua New Guinea. Journal of Mammalogy 90, 340–346.
- Osborne, R.A.L., 1983. Cainozoic stratigraphy at Wellington Caves, New South Wales. Proceedings of the Linnean Society of N.S.W. 107, 131–147.
- Pascual, R., Archer, M., Jaureguizar, E.O., Prado, J.L., Godthelp, H. & Hand, S.J., 1992. First discovery of monotremes in South America. Nature 356, 704–706.
- Pascual, R., Goin, F.J., Balarino, L. & Udriazar Sauthier, D.E., 2002. New data on the Paleocene monotreme Monotrematum sudamericanum, and the convergent evolution of triangulate molars. Acta Palaeontologica Polonica 47, 487–492.
- Peters, W. & Doria, G., 1876. Descrizione di una nuova specie di Tachyglossus proveniente dalla Nuova Guinea settentrionale. Annali del Museo Civico Storia Naturale di Genova 9, 183–185.
- Pettigrew, J.D., 1999. Electroreception in monotremes. Journal of Experimental Biology 202, 1447–1454.
- Phillips, M.J., 2015. Four mammal fossil calibrations: balancing competing palaeontological and molecular considerations. Palaeontologia Electronica 18, 1–16.
- Phillips, M.J., Bennett, T.H. & Lee, M.S.Y., 2009. Molecules, morphology, and ecology indicate a recent, amphibious ancestry for echidnas. Proceedings of the National Academy of Sciences USA 106, 17089–17094.
- Pian, R., Archer, M. & Hand, S.J., 2013. A new, giant platypus, Obdurodon tharalkooschild, sp. nov. (Monotremata, Ornithorhynchidae), from the Riversleigh World Heritage Area. Australia. Journal of Vertebrate Palaeontology 33, 1255–1259.
- Pian, R., Archer, M., Hand, S.J., Beck, R.M. & Cody, A., 2016. The upper dentition and relationships of the enigmatic Australian Cretaceous mammal Kollikodon ritchiei. Memoirs of Museum Victoria 74, 97–105.
- Piper, K.J., 2007. Early Pleistocene mammals from the Nelson Bay Local Fauna, Portland, Victoria, Australia. Journal of Vertebrate Paleontology 27, 492–503.
- Piper, K.J., 2016. The Macropodidae (Marsupialia) of the early Pleistocene Nelson Bay Local Fauna, Victoria, Australia. Memoirs of Museum Victoria 74, 233–253.
- Pledge, N.S., 1990. The upper fossil fauna of the Henschke fossil cave, Naracoorte, South Australia. Memoirs of the Queensland Museum 28, 247–262.
- Polhemus, D.A., 2007. Tectonic geology of Papua. In The Ecology of Papua, Part One. Marshall, A.J., & Beehler, B.M., eds, Periplus, Singapore, 137–164.
- Poropat, S.F., Martin, S.K., Tosolini, A.M.P., Wagstaff, B.E., Kear, B.P., Vickers-Rich, P. & Rich, T.H., 2018. Early Cretaceous polar biotas of Victoria, southeastern Australia—an overview of research to date. Alcheringa 42, 158–230.
- Price, G.J., Louys, J., Smith G, K. & Kramb, J., 2019. Shifting faunal baselines through the Quaternary revealed by cave fossils of eastern Australia. PeerJ 6, e6099.
- Price, G.J. & Webb, G.E., 2006. Late Pleistocene sedimentology, taphonomy and megafauna extinction on the Darling Downs, southeastern Queensland. Australian Journal of Earth Sciences 53, 947–970.
- Price, G.J., Webb, G.E., Zhao, J., Feng, Y., Murray, A.S., Cooke, B.N., Hocknull, S.A. & Sobbe, I.H., 2011. Dating megafaunal extinction on the Pleistocene Darling Downs, eastern Australia: the promise and pitfalls of dating as a test of extinction hypotheses. Quaternary Science Reviews 30, 899–914.
- Prideaux, G.J., 1999. Borungaboodie hatcheri gen. et sp. nov., a very large bettong (Marsupialia: Macropodoidea) from the Pleistocene of southwestern Australia. Records of the Western Australian Museum Supplement 57, 317–329.
- Prideaux, G.J., Gully, G.A., Couzens, A.M.C., Ayliffe, L.K., Jankowski, N.R., Jacobs, Z., Roberts, R.G., Hellstrom, J.C., Gagan, M.K. & Hatcher, L.M., 2010. Timing and dynamics of Late Pleistocene mammal extinctions in southwestern Australia. Proceedings of the National Academy of Sciences USA 107, 22157–22162.
- Pridmore, P.A., Rich, T.H., Vickers-Rich, P. & Gambaryan, P.P., 2005. A tachyglossid-like humerus from the Early Cretaceous of south-eastern Australia. Journal of Mammalian Evolution 12, 359–378.
- Ratman, N., 1998. Geology of the Bird’s Head, Irian Jaya, Indonesia. In Perspectives on the Bird’s Head of Irian Jaya. Miedema, J., Odé, C, & Dam, R.A.C., eds, Rodopi, Amsterdam, 719–755.
- Rauhut, O.W., Martin, T., Ortiz-Jaureguizar, E., & Puerta, P., 2002. A Jurassic mammal from South America. Nature 416, 165–168.
- Reed, E.H. & Bourne, S.J., 2009. Pleistocene fossil vertebrate sites of the southeast region of South Australia II. Transactions of the Royal Society of South Australia 133, 30–40.
- Retief, J.D., Winkfein R, J. & Dixon G, H., 1993. Evolution of the monotremes: the sequences of the protamine P1 genes of platypus and echidna. European Journal of Biochemistry 218, 457–461.
- Rich, T.H., 1991. Appendix 1, Australian Mesozoic and Tertiary terrestrial mammal localities. In Vertebrate Palaeontology of Australasia. Vickers-Rich, P. ed., Pioneer Design Studio, Clayton, Victoria, Australia 1005–1058.
- Rich, T.H., Flannery, T.F., Evans, A.R., White, M., Ziegler, T., Maguire, A., Poropat, S., Trusler, P. & Vickers-Rich, P., 2020. Multiple hypotheses about two mammalian upper dentitions from the Early Cretaceous of Australia. Alcheringa 44, 528–536.
- Rich, T.H., Flannery, T.F., Trusler, P., Kool, L., VAN Klaveren, N.A. & Vickers-Rich, P., 2001. A second tribosphenic mammal from the Mesozoic of Australia. Records of the Queen Victoria Museum 110, 1–9.
- Rich, T.H., Flannery, T.F., Trusler, P., Kool, L., VAN Klaveren, N.A. & Vickers-Rich, P., 2002. Evidence that monotremes and ausktribosphenids are not sistergroups. Journal of Vertebrate Paleontology 22, 466–469.
- Rich, T.H., Flannery, T.F. & Vickers-Rich, P., 2020. Evidence for a remarkably large toothed monotreme from the Early Cretaceous of Lightning Ridge, NSW, Australia. In Biological Consequences of Plate Tectonics: New Perspectives on Post-Gondwana and Break-up—a Tribute to Ashok Sahni, Vertebrate Paleobiology and Paleoanthropology. Prasad, G.V. & Patnaik, R., eds., Springer International, Cham, Switzerland.
- Rich, T.H., Hopson, J.A., Gill, P.G., Trusler, P., Rogers-Davidson, S., Morton, S., Cifelli, R.L., Pickering, D., Kool, L., Siu, K., Burgmann, F.A., Senden, T., Evans, A.R., Wagstaff, B.E., Seegets-Villiers, D., Corfe, I.J., Flannery, T.F., Walker, K., Musser, A.M., Archer, M., Pian, R. & Vickers-Rich, P., 2016. The mandible and dentition of the Early Cretaceous monotreme Teinolophos trusleri. Alcheringa 40, 475–501.
- Rich, T.H., Trusler, P., Kool, L., Pickering, D., Evans, A., Siu, K., Maksimenko, A., Kundrat, M., Gostling, N.J., Morton, S. & Vickers-Rich, P., 2020. A third, remarkably small, tribosphenic mammal from the Mesozoic of Australia. In Biological Consequences of Plate Tectonics: New Perspectives on Post-Gondwana and Break-up—a Tribute to Ashok Sahni, Vertebrate Paleobiology and Paleoanthropology. In Prasad, G.V. & Patnaik, R., eds, Springer International, Cham, Switzerland.
- Rich, T.H. & Vickers-Rich, P., 2000. Dinosaurs of Darkness. In Search of the Lost Polar World. Indiana University Press, Bloomington, Indiana, USA.
- Rich, T.H. & Vickers-Rich, P., 2004. Diversity of early Cretaceous mammals from Victoria, Australia. Bulletin of the American Museum of Natural History 285, 36–53.
- Rich, T.H., Vickers-Rich, P., Constantine, A., Flannery, T.F., Kool, L. & VAN Klaveren, N., 1999. Early Cretaceous mammals from Flat Rocks, Victoria, Australia. Records of the Queen Victoria Museum 106, 1–34.
- Rich, T.H., Vickers-Rich, P., Flannery, T.F., Kear, B.P., Cantrill, D.J., Komarower, P., Kool, L., Pickering, D., Trusler, P., Morton, S., VAN Klaveren, N. & Fitzgerald E, M.G., 2009. An Australian multituberculate and its palaeobiogeographic implications. Acta Palaeontologica Polonica 54, 1–6.
- Rismiller, P.D. & Grutzner, F., 2019. Tachyglossus aculeatus (Monotremata: Tachyglossidae). Mammalian Species 51, 75–91.
- Roberts, R.G., Flannery, T.F., Ayliffe L, K., Yoshida, H., Olley, J.M., Prideaux, G.J., Laslett, G.M., Baynes, A., Smith, M.A., Jones, R. & Smith, B.L., 2001. New ages for the last Australian megafauna: continent-wide extinction about 46,000 years ago. Science 292, 1888–1892.
- Robertson, D.S., Lewis, W.M., Sheehan, P.M. & Toon, O.B., 2013. K-Pg extinction patterns in marine and freshwater environments: the impact winter model. Journal of Geophysical Research: Biogeosciences 18, 1006–1014.
- Rougier, G.W., Martinelli, A.G., Forasiepi, A.M. & Novacek, M.J., 2007. New Jurassic mammals from Patagonia, Argentina: a reappraisal of australosphenidan morphology and interrelationships. American Museum Novitates 3566, 1–54.
- Rowe, T., Rich, T.H., Vickers-Rich, P., Springer, M. & Woodburne, M.O., 2008. The oldest platypus and its bearing on divergence timing of the platypus and echidna clades. Proceedings of the National Academy of Sciences USA 105, 1238–1242.
- Scanlon, J.D., 2005. Australia’s oldest known snakes: Patagoniophis, Alamitophis, and cf. Madtsoia (Squamata: Madtsoiidae) from the Eocene of Queensland. Memoirs of the Queensland Museum. Proceedings of the Conference of Australasian Vertebrate Evolution, Palaeontology and Systematics 51, 215–223.
- Scanlon, J.D., Lee, M.S.Y. & Archer, M., 2003. Mid-Tertiary elapid snakes (Squamata, Colubroidea) from Riversleigh, northern Australia: early steps in a continent-wide adaptive radiation. Geobios 36, 573–601.
- Schneider, E.R., Anderson, E.O., Mastrotto, M., Matson, J.D., Schulz, V.P., Gallagher, P.G., Lamotte, R.H., Gracheva, E.O. & Bagriantsev, S.N., 2017. Molecular basis of tactile specialization in the duck bill. Proceedings of the National Academy of Sciences 114, 13036–13041.
- Schneider, E.R., Gracheva, E.O. & Bagriantsev, S.N., 2016. Evolutionary specialization of tactile perception in vertebrates. Physiology (Bethesda, Md.) 31, 193–200.
- Schodde, R. & Christidis, L., 2014. Relicts from Tertiary Australasia: undescribed families and subfamilies of songbirds (Passeriformes) and their zoogeographic signal. Zootaxa 3786, 501–522.
- Scott, H.H. & Lord, C.E., 1921. Studies in Tasmanian mammals, living and extinct. Number V. Zaglossus harrissoni, sp. nov. Papers and Proceedings of the Royal Society of Tasmania 1921, 13–15.
- Sereno, P.C., 2006. Shoulder girdle and forelimb in a Cretaceous multituberculate: form, functional evolution, and a proposal for basal mammalian taxonomy. In Amniote Paleobiology: Perspectives on the Evolution of Mammals, Birds, and Reptiles. M.T. Carrano, T.J. Gaudin, R.W. Blob, and J.R. Wible, eds, University of Chicago Press, Chicago, 315–370.
- Shaw, G., 1792. The Naturalist’s Miscellany 3 (36), London: F.P. Nodder & Co.
- Shaw, G., 1799. The Naturalist’s Miscellany 10 (118), London: F.P. Nodder & Co.
- Sigé, B., Archer, M., Crochet, J.-E., Godthelp, H., Hand, S. & Beck, R., 2009. Chulpasia et Thylacotinga, marsupiaux bunodontes gondwaniens transantarctiques d’âge paléocène supérieur-éocène inférieur: nouvelles données d’Australie. Geobios 42, 813–823.
- Sigogneau-Russell, D., Hooker, J.J. & Ensom, P.C., 2001. The oldest tribosphenic mammal from Laurasia (Purbeck Limestone Group, Berriasian, Cretaceous, UK) and its bearing on the ‘dual origin’ of Tribosphenida. Comptes Rendus de l'Académie des Sciences-Series IIA-Earth and Planetary Science 333, 141–147.
- Tarver, J.E., Dos Reis, M., Mirarab, S., Moran, R.J., Parker, S., O’Reilly, J.E., King, B.L., O’Connell, M.J., Asher, R.J., Warnow, T., Peterson, K.J., Donoghue, P.C.J. & Pisani, D., 2016. The interrelationships of placental mammals and the limits of phylogenetic inference. Genome Biology and Evolution 8, 330–344.
- Thomas, M.O., 1907. On the occurrence of Acanthoglossus in British New Guinea. Annals and Magazine of Natural History (series 7) 20, 293–294.
- Toussaint, E.F.A., White, L.T., Shaverdo, H., Lam, A., Surbakti, S., Panjaitan, R., Sumoked, B., von Rintelen, T., Sagata, K. & Balke, M., 2021. New Guinean orogenic dynamics and biota evolution revealed using a custom geospatial analysis pipeline. BMC Ecology and Evolution 21, 51.
- Turney, C.S.M., Flannery, T.F., Roberts, R.G., Reid, C., Fifield, L.K., Higham, T.F.G., Jacobs, Z., Kemp, N., Colhoun, E.A., Kalin, R.M. & Ogle, N., 2008. Late-surviving megafauna in Tasmania, Australia, implicate human involvement in their extinction. Proceedings of the National Academy of Sciences USA 105, 12150–12153.
- VAN Dyck, S. & Crowther, M.S., 2000. Reassessment of representatives of the northern Antechnius stuartii complex (Marsupialia: Dasyuridae): A. subtropicus sp. nov. and A. adustus new status. Memoirs of the Queensland Museum 45, 611–635.
- Wagstaff, B.E., Gallagher, S.J., Hall, W.M., Korasidis, V.A., Rich, T.H., Seegets-Villiers, D.E. & Vickers-Rich, P.A., 2020. Palynological-age determination of Early Cretaceous vertebrate-bearing beds along the south Victorian coast of Australia, with implications for the spore-pollen biostratigraphy of the region. Alcheringa 44, 460–474.
- Weil, A., 2005. Mammalian palaeobiology: living large in the Cretaceous. Nature 433, 116–117.
- Whitelaw, M., 1991. Magnetic polarity stratigraphy of Pliocene and Pleistocene fossil vertebrate localities in southeastern Australia. Geological Society of America Bulletin 103, 1493–1503.
- Wible, J.R., Rougier, G.W., Novacek, M.J. & Asher, R.J., 2009. The eutherian mammal Maelestes gobiensis from the Late Cretaceous of Mongolia and the phylogeny of Cretaceous Eutheria. Bulletin of the American Museum of Natural History 327, 1–123.
- Wible, J.R., Rougier, G.W., Novacek, M.J., Mckenna, M.C. & Dashzeveg, D.D., 1995. A mammalian petrosal from the Early Cretaceous of Mongolia: implications for the evolution of the ear region and mammaliamorph relationships. American Museum Novitates 3149, 1–19.
- Wilkinson, J.E., Spring, K.A., Dunn, T.L., Price, G.J. & Louys, J., 2021. The vertebrate fossil collection record from the Chinchilla Sand, South-East, Queensland, Memoirs of the Queensland Museum 63, 1844–2021.
- Wilson, J.T., 1901. On the skeleton of the snout of the mammary foetus of monotremes. Proceedings of the Linnean Society of New South Wales 26, 717–737.
- Woodburne, M.O., 1967. The Alcoota Fauna, Central Australia. Bureau of Mineral Resources Geology and Geophysics, Australia Bulletin 87, 1–187.
- Woodburne, M.O., 2003. Monotremes as pretribosphenic mammals. Journal of Mammalian Evolution 10, 195–248.
- Woodburne, M.O., Macfadden, B.J., Case, J.A., Springer, M.S., Pledge, N.S., Power, J.D., Woodburne, J.M. & Springer, K.B., 1994. Land mammal biostratigraphy and magnetostratigraphy of the Etadunna Formation (Late Oligocene) of South Australia. Journal of Vertebrate Paleontology 13, 483–515.
- Woodburne, M.O., Rich, T.H. & Springer, M.S., 2003. The evolution of tribospheny and the antiquity of mammalian clades. Molecular Phylogenetics and Evolution 28, 360–385.
- Woodburne, M.O. & Tedford, R., 1975. The first Tertiary monotreme from Australia. American Museum Novitates 2588, 1–11.
- Woodhead, J., Hand, S.J., Archer, M., Raham, I., Sniderman, K., Arena, D.A., Black, K.H., Godthelp, H., Creaser, P. & Price, E., 2016. Developing a radiometrically-dated chronologic sequence for Neogene biotic change in Australia, from the Riversleigh World Heritage Area of Queensland. Gondwana Research 29, 153–167.
- Zhou, Y., Shearwin-Whyatt, L., Li, J., Song, Z., Hayakawa, T., Stevens, D., Fenelon, J.C., Peel, E., Cheng, Y., Pajpach, F., Bradley, N., Suzuki, H., Nikaido, M., Damas, J., Daish, T., Perry, T., Zhu, Z., Geng, Y., Rhie, A., Sims, Y., Wood, J., Haase, B., Mountcastle, J., Fedrigo, O., Li, Q., Yang, H., Wang, J., Johnston, S.D., Phillippy, A.M., Howe, K., Jarvis, E.D., Ryder, O.A., Kaessmann, H., Donnelly, P., Korlach, J., Lewin, H.A., Graves, J., Belov, K., Renfree, M.B., Grutzner, F., Zhou, Q. & Zhang, G., 2021. Platypus and echidna genomes reveal mammalian biology and evolution. Nature 592, 756–762.