Abstract
The field of nanotechnology has allowed for increasing nanoparticle (NP) exposure to the male reproductive system. Certain NPs have been reported to have adverse consequences on male germ and somatic cells. Germ cells are the bridge between generations and are responsible for the transmission of genetic and epigenetic information to future generations. A number of NPs have negative impacts on male germ and somatic cells which could ultimately affect fertility or the ability to produce healthy offspring. These impacts are related to NP composition, modification, concentration, agglomeration, and route of administration. NPs can induce severe toxic effects on the male reproduction system after passing through the blood–testis barrier and ultimately damaging the spermatozoa. Therefore, understanding the impacts of NPs on reproduction is necessary. This review will provide a comprehensive overview on the current state of knowledge derived from the previous in vivo and in vitro research on effects of NPs on the male reproductive system at the genetic, cellular, and molecular levels.
Introduction
The uses of nanoparticles (NPs) and nanodelivery systems in medicine are set to continue spread rapidly and become more important in the medical field for applications in drug delivery and diagnosis. However, the large-scale production and applications of NPs in industry and households leads to the release of such materials into the environment (Khan et al. Citation2019), and pose potential risks to human health (Massarsky et al. Citation2014). NPs are also universal in the environment and can enter the human body by inhalation, ingestion, skin adsorption, and via intravenous injection when used for medical applications (Chen et al. Citation2010). NPs could be released into the environment, enter into cells easily via free penetration or receptor-mediated endocytosis, and interact with cellular components including proteins, lipids, and genomic DNA (Shalviri et al. Citation2012). Studies have shown the male reproductive system is more susceptible to various stresses such as NPs than other organ systems (Ban et al. Citation2018). Evaluating the risk of these NPs in the environment needs an understanding of their mobility, reactivity, ecotoxicity, and persistency (Golobič et al. Citation2012). The active interest in male reproductive health in epidemiological studies, since young males in some regions showed suboptimal sperm quality and sperm number (Huang et al. Citation2017). Previous animal studies have shown a direct causal effect relationship between the decline of sperm quality/quantity and NPs (Li et al. Citation2016). The human impact on environmental studies, particularly those examining the effects of particulate emissions in diesel exhaust showed that constant daily exposure to NPs could result in aggregation of such particles in cells and thus interfere with certain physiological functions (Chou et al. Citation2008).
The male reproductive organ has been identified to be vulnerable to environmental stress such as external toxicants and even NPs (Tang et al. Citation2019). Toxicity studies attempt to track the circulation of NPs throughout the body by investigating the tissue distribution of such particles in various organs. Previous studies reported that various types of NPs are able to pass biological barriers and exert toxic effects on important tissues (Wang et al. Citation2018). NPs could pass through the blood–testis barrier (BTB) due to their small size, and accumulate in reproductive organs (Lan and Yang Citation2012). NPs have been used for drugs and drug delivery systems (DDSs) and for fluorescence imaging in the reproductive system. Nanomaterials (NMs) have been used widely as a drug carrier for targeted therapy directly combining with target organs, providing an opportunity for NMs to enter the reproductive system directly (Lee et al. Citation2007). A study reported that TiO2-NPs were able to cross the BTB inducing inflammation, cytotoxicity, and gene expression alterations that lead to damage of the male reproductive system (Santonastaso et al. Citation2019). The capacity of some NPs to induce reactive oxygen species (ROS) production, DNA damage, and apoptosis displays a promise for their use as antibacterial and cancer therapy. However, their intrinsic toxicity, easy entrance, and accumulation in organisms have raised some concerns regarding the medical use of these NPs. A number of studies have reported that NPs might induce cytotoxic effects on the male reproductive system, compromising male fertility (Pinho, Rebelo, et al. Citation2020).
Male reproductive system and development nanotoxicity
Reproductive medicine has been a developing area aimed to improve the possibility to safe conception and the delivering of healthy children (Lipskind and Gargiulo Citation2013). A number of studies have shown that use of NPs in detection and targeted therapy associated with reproductive cancers (Barkalina et al. Citation2014). For instance, super-paramagnetic iron oxide NPs and aptamer-conjugated NPs, especially gold NPs (Apt-AuNPs) have been used widely for the treatment of prostate cancer patients (Wang et al. Citation2008; Kim et al. Citation2010), and also to determine the expression of genes in offspring (Campos et al. Citation2011). Reproductive toxicity results from exposure to certain NMs with injurious impacts on normal reproductive function and fertility in adult males, including disruption of male germ cells and embryonic–fetal growth during their reproductive years (Adler et al. Citation2011). Different composition and metabolic function among different phases in the male germ cells result in differing vulnerabilities to mutation induction and genotoxicity. There are, however, some types of chemicals which show phase-specific cytotoxicity to spermatogonia, spermatocytes, and spermatids (Habas et al. Citation2020). For example, sperms in the epididymis were found to be more sensitive than both spermatogonia and spermatocytes to silica NP toxicity (Xu et al. Citation2014). NPs may have negative effects on the development and reproductive organ function, physiological structure, germ cells, and fertility in humans and animals (Brohi et al. Citation2017).
In vitro nanotoxicity studies generally utilize simple tests performed by a practical prescreening method using various cell types and culture conditions. Although they may yield some important data, such studies often fail to demonstrate what really happens within a whole living organism (Lewinski et al. Citation2008). Researchers have recently been relying on both in vivo and in vitro nanotoxicity testing to assess and detect any possible health impacts of NMs (Bahadar et al. Citation2016). This review has considered in vitro and in vivo research into NP-induced toxic impact on the male reproductive system. This type of research involves experimental model organisms like rats and mice (Kong et al. Citation2016). The in vitro studies used a variety of methods which could serve as guidelines for the design of in vivo experiments. In vitro data may help to reduce and/or replace animal testing according to the 3Rs.
Nontoxic effects in male germ cells
Gametogenesis is a complex and highly regulated process which is sensitive to environmental insult, including environmental toxic chemicals (Iona et al. Citation2002). Chemical effects on germ cells and their maturation can affect fertility through a decline in the number of sperm produced which may have negative consequences for the development of the offspring (Anway and Skinner Citation2008). Studies have reported that these particles are toxic to the testicular tissues (Yauk et al. Citation2008). Despite many applications of NMs, there is insufficient information relative to the impact of manufactured NPs on human reproductive health. Following systemic administration, NPs are small enough to penetrate very small capillaries throughout the body, therefore, offer the most effective distribution to certain tissues. NPs can pass through biological membranes and affect the physiology of any cell in an animal body (Kashiwada Citation2006). A study has shown that nSP70 could penetrate the BTB and nuclear membranes of spermatozoa without significant testicular damage (Gromadzka-Ostrowska et al. Citation2012). Moreover, amorphous nanosilica particles (nSPs) can penetrate the BTB and the nuclear membranes of spermatocytes and showed no significant testicular damage (Morishita et al. Citation2012).
A study has also shown that NPs can accumulate in the testis prostate gland and epididymis by direct contact with NPs or blood circulation and causing cytotoxicity (Wang et al. Citation2018). A study has reported that epididymis, testis, and seminiferous tubules are the main targets of NPs in the genital area through different ways (Zhao H et al. Citation2014). In male rats, oral administration of nanosized TiO2 and nickel (Ni) NPs had toxic effects on the reproductive system, causing marked changes in weight of the testis and epididymis (Morgan et al. Citation2017). A histological study has also shown that graphene oxide exposure was associated with structural damage in the testis, which included atrophy of seminiferous tubules with a reduction in germinal epithelium, germ cell loss, and vacuolization (Nirmal et al. Citation2017). It was reported that male rats exposed to silver nanoparticles (AgNPs) induced alterations in the testis seminiferous tubule morphometry (Lopes et al. Citation2019). Cerium oxide nanoparticles (CeO2NPs) revealed congestion and degeneration of seminiferous tubules and decreased sperm motility and count and increased total sperm abnormality in mice (Adebayo et al. Citation2018).
Cytotoxic NPS target male germ cell and supporting cells
Seminiferous tubules and spermatogenesis
The seminiferous tubules are the site of spermatogenesis and within this process the DNA of male germ cells may be injured due to ROS production (Mahfouz et al. Citation2010). Exposure to NPs induces histological changes in seminiferous tubules of testicular tissues, resulting to testicular damage and declined sperm production. The male reproductive system is known to be affected by the exposure to NPs, mainly in the form of a reduction in sperm production (Boisen et al. Citation2012), and impaired spermatogenesis, from primordial germ cells to sperm cells in the seminiferous tubules (). However, this impact has been reported to show variations across individual species (Gao et al. Citation2013). The main cause of male infertility is due to low sperm count, and has been associated with specific molecule-level changes that could affect phase-specific expression of multiple genes regulating the spermatogenesis process (Hong et al. Citation2015). Research into the impact of NPs on spermatogenesis suggests that further precautionary measures should be taken during nanomedical procedures since it is not yet fully understood how NPs penetrate the BTB (Yoshida et al. Citation2010). The testes contain various types of cells, such as Sertoli and Leydig cells, besides the spermatogenic ones, spermatocytes, spermatids, and spermatogonia. Each type of these germ cells can be isolated in laboratory to explain the genetic impacts of NPs (Habas et al. Citation2020).
Table 1. Nanoparticles (NPs) effects on male reproductive system.
Once they have penetrated the uterus, NPs may seriously affect the reproductive cycle beginning from the fetal stage. For instance, Takeda et al. (Citation2009) found that subcutaneous administration of titanium dioxide nanoparticles (NTiO2) (less than 300 nm in diameter) at a dose of 400 µg to pregnant mice could facilitate NP entry into the male offspring's Sertoli cells, spermatids, and Leydig cells. They also observed a reduction in the number of Sertoli cells, alterations in testicular morphology, disruption of seminiferous tubules, and a significant decrease in daily sperm production (DSP) (Takeda et al. Citation2009). Sperm motility was also observed to change in 6-week old male offspring, after exposure to titanium dioxide NPs during the fetal stage was shown to impair the male reproductive capacity at the basic level of spermatogenesis. Another study reported that intratracheal administration of carbon NPs (14 nm) to pregnant ICR mice on the 7th and 14th days of gestation (at a total dose of 400 µg) caused degeneration of germ cells within the seminiferous tubule, and a reduction in DSP in male offspring (Yoshida et al. Citation2009). Curcumin has shown many pharmacological activities in both preclinical and clinical studies. However, curcumin NPs have found to affect the proliferation of testicular cell lines in vitro, causing an acute injury to the testicular functions in male mice (Xia et al. Citation2020).
Exposure to NPs may also cause direct alterations in the seminiferous tubules and development of the sperm cells in adult males. An in vivo study on mice delivering cobalt NPs through intratracheal instillation at a dose of 0.1 mg for 10 times a week observed size-depended changes in the seminiferous tubules, with the smaller particles, though similar in number, causing less damage, thus indicating that the particle size could be exerting a greater impact on the production of spermatozoa (Bai et al. Citation2010). Research conducted with an intravenous injection of multi-walled carbon nanotubes into male mice detected that nanotubes caused some direct damage to the testes, including increased levels of ROS and a thickened epithelium of tubules, which could be repaired in 90 days without any significant impairment in fertility (Kashiwada Citation2006). The tests performed after the 90-day period detected no deterioration in the sperm count and quality or in testosterone levels of the adult mice, which were still fertile and able to produce healthy offspring. Such research findings suggest that the toxicity and mutagenicity of NPs might be highly dependent on the coating, shape, and size of such NM.
Nanotoxicity on the testes
Even though some in vivo research has shown that NPs could accumulate in the testes of animals, they are reported to exhibit rather limited distribution in cells, and there is inconclusive evidence to establish toxic effects induced by accumulation of such particles. For example, experiments on Kunming albino mice exposed to silicon NPs with sodium chloride or sodium iodide coating at a dose of 0–225 mg/kg detected some NP accumulation in the testes after 96 hours, yet no abnormal changes in cells or in animal mortality was observed (Chen et al. Citation2003). A similar finding was reported by Kim et al. (Citation2006), who administrated silica-coated magnetic NPs containing a fluorogenic dye called rhodamine B isothiocyanate (50 nm in diameter) to male IRC mice for 4 weeks and found that the NPs accumulated in the testes had no observable toxic impact on the reproductive system. Another study also revealed that fluorescent NPs measuring 39.4 nm in diameter accumulated in the testes of Medaka fish (Oryzias latipes), without any toxic effects on the cell nuclei or increased mortality even at higher doses in the offspring (Leclerc et al. Citation2015). However, more recent research has failed to find evidence of NPs accumulation in the testes of mice 45 days after the delivery of silica-gold (Au) core–shell NPs (70 nm) through the intramuscular route (Ong et al. Citation2016). The toxic effects of selenium nanoparticles (SeNPs) have shown effects on sperm characteristics, oxidative status, sexual behavior, and the histological alterations of the testes and epididymis in male rats (Hozyen et al. Citation2020).
In contrast, some in these NPs have been reported to injure male fecundity once they have accumulated in the testes. Research into the toxicity of silver NPs, shown to impair male reproduction, suggests that ingested Ag NPs may accumulate in the testes and damage germline stem cells (Habas et al. Citation2018). Subchronic toxicity experiments involving oral exposure of Ag NPs coated with polyvinyl propylene to rats found that this type of relatively prolonged exposure induced changes in the cellular structure of the testes and abnormal sperm morphology (Hong et al. Citation2016). Exposure to titanium dioxide particles was also shown to cause a significant rise in sperm mutation in male mice exposed to titanium dioxide NPs during their fetal stage in the uterus (Ritz et al. Citation2011). It should be noted that factors like relative solubility of a material and its ability to penetrate tissue barriers can play a key role in the degree of its toxicity. Some stable water-soluble NPs, such as Au (Mohammad Citation2019), and Ag NPs, have little toxicity as they could be excreted from cells through the normal routes; however, insoluble particles with a long biological half-life tend to cause far greater damage to living cells. The impaired male fertility through toxicity, apoptosis, and/or necrosis of germ cells in mice upon exposure to zinc oxide nanoparticles (ZnO NPs), TiO2 NPs, Ni NPs, manganese dioxide (MnO2) NPs, graphene quantum dot, and multiwalled carbon nanotubes (MWCNTs) has been associated with compromised immunity and increased inflammatory responses in the testicular cells (Kong et al. Citation2019; Rafiee et al. Citation2019; Zhang et al. Citation2019). Furthermore, intratracheal administration of relatively smaller carbon NPs (14 nm) at a dose of 1 mg has been reported to cause low sperm count in ICR mice (Bai et al. Citation2010).
Given the importance of reproduction across species including humans and the widespread use of NPs in foods, personal hygiene products, and electronics, it appears that more research is clearly warranted to elucidate possible consequences of NP accumulation in the testes by using different doses, NMs, and administration routes.
Nanotoxicity in germline and/or spermatozoa
Testicular weight relies on the germ cell mass and reduce in testicular weight could be due to the death of germ cells and defects in spermatogenesis (Karimi et al. Citation2019). These changes could be due to NPs that, after accumulating in testes, may induce their toxicity via altering sperm morphology, number and viability. However, studying effects in male germ cells could allow us to determine the potential hazards of NPs to animal reproduction based on the material, size, and shape of the particles. Designed to examine the impact of NPs on sperm cells, one human study, directly introduced Au NPs (9 nm) to fresh semen from a healthy male (Wiwanitkit et al. Citation2009); exposure to NPs at a dose of 44 µg/mL resulted in a loss of motility in 25% of the sperm, because the particles accumulated in the sperm tails and head. NPs can easily cross the BTB, reached the testes and then accumulated, after accumulation in the testis, induce adverse effects on spermatogenesis, resulting in poor quality of sperm, changes in hormone level and testicular lesions (Gao et al. Citation2013). However, it has been reported that NPs primarily affect the maturation process of spermatozoa in the epididymis. This was found to be more sensitive to NPs toxicity compared to spermatogenic cells, mainly spermatogonia and spermatids. The NPs have damaging effects on the quantity and quality of sperm in the epididymis by inducing oxidative stress and damaging the structure of mitochondria, resulting in energy metabolism dysfunction (Xu et al. Citation2014). Considering that several imaging techniques like CT scans and X-ray imaging currently utilize high doses of NPs (e.g. Au NPs) as contrast agents, they may be distributed to all organ systems throughout the body, including the reproductive system and impair fertility by damaging sperm cells. To that end, researchers directly exposed bovine sperm to iron oxide NPs coated with polyvinyl alcohol, observing that NPs accumulated on mitochondria in the sperm tail and on the acrosomal membrane in the head, with no detectable effects on sperm motility (Ben-David Makhluf et al. Citation2006). Recently, it has been reported that Mn3O4 NPs with the size of 20 nm significantly decrease the sperm quality of rats, resulting to the decline in fertility after repeated intravenous injection for 120 days (Zhang et al. Citation2020). Regarded as the bedrock of spermatogenesis and male fertility, spermatogonial stem cells (SSCs) have been proven to be of great value for in vitro animal testing conducted to determine the toxicity of different NPs in male reproduction (Braydich-Stolle et al. Citation2005). A controlled in vitro study comparing the effects of different types of NPs on germ cells derived from bovine and mice testes assessed certain variables such as cell morphology, LDH leakage, apoptosis status, and mitochondrial function upon sustained exposure to such particles (Braydich-Stolle et al. Citation2005). The study found no significant change in cell morphology, concentration-dependent cytotoxicity to mitochondrial function, dose-dependent increase in LDH leakage, and apoptosis induced by certain types of NPs. Among all NPs tested (Ag, molybdenum (Mo), and aluminum (Al)), Ag NPs (15 nm) were found to have the greatest toxic impact on the C18-4 germline stem cells. This type of research design in particular enables a healthy comparison of NP cytotoxicity under the same experimental conditions, helping the elimination the current uncertainty about the impact of NMs on the male fertility and reproductive capacity. A recent study has reported that nano-TiO2 can penetrate through the BTB and enter the testicular tissue, leading to damage to the testis and epididymis and reduces sperm concentration via disrupting meiosis and related signaling pathways (Hong et al. Citation2020).
Despite the widespread adoption of NMs by diverse fields and industries, we still have limited knowledge on the impact of engineered NMs on the environment and human health. Also, a broad range of industrial settings may be releasing small particulate matter that mimic NPs. For instance, environmental risks like air pollution are known to contain concentrations of ultrafine silver particles that may slip into lungs. After chronic exposure, NPs can penetrate even the tiniest capillaries throughout the body and distribute to a variety of tissues. Previous research examining the impact of the particulate matter suspended in air on mice exposed to ambient air in a polluted industrial area in the vicinity of steel mills detected mutations in germ line cells (Yauk et al. Citation2008). Such findings, among other observations, point to the urgent need for further research geared toward better health assessment of humans residing around industrial areas that may be exposed to NPs contained in environmental pollutants.
Mechanisms involved in male germ cell and somatic cell toxicity
Nanoparticles can enter into the reproductive system in different paths. In drug delivery, penetration of NPs into target cells is very important. AuNPs has been commonly used in many medicine applications and has been known to cause detrimental effects on sperm morphology, motility, and DNA damage (Taylor et al. Citation2012). NPs have been commonly used for dermatological therapy, for skin care and in diagnostic imaging of skin diseases (Niska et al. Citation2018). Sub-dermal exposure of Ag NPs for 7 and 28 days showed decline in sperm number and motility in addition to in testosterone, luteinizing hormone (LH), and follicle stimulating hormone levels along with histological abnormalities in the testis in male rats (Olugbodi et al. Citation2020). NPs entering the human body through inhalation can deposit in the respiratory tract, particularly in the lungs, and their high surface reactivity increases their capacity to induce inflammation, generation of ROS, necrosis, and pulmonary injury (Del Vento et al. Citation2019). NPs can also be absorbed in the gastrointestinal tract and then enter into the blood, therefore, easily target other organs and accumulating there (Hansson Citation2012). Oral administration of Ag NPs has been found to be induced reduction in sperm number in male rats (Sleiman et al. Citation2013). A study has reported that the adverse effects of NPs on male reproductive function were mainly due to modification of the testicular structure, damage of spermatogenesis, and alteration in the biosynthetic and catabolic pathways of testosterone (Iavicoli et al. Citation2013). NPs entered into the reproductive system, could induce different toxic effects at the reproductive organs, cell, and hormone levels (). Several in vitro studies have evaluated the potential adverse health effects of NPs, pointing out their ability to produce ROS which is generally considered the main pathway in the toxicity of NPs. These reactive molecules and free radicals are unstable forms of oxygen that often tend to remain close to their site of production (Wells et al. Citation2005). An imbalance between oxidant and antioxidant mechanisms, however, heightens oxidative stress, thus inducing or exacerbating NP-related inflammatory response. Under such circumstances, both NPs and the inflammation may travel beyond the respiratory tract or the lungs. With the translocation of NPs, all types of inflammatory mediators might leak into the systemic circulation to promote further inflammation (Erdely et al. Citation2011). The particles traveling throughout the body can ultimately end up in the reproductive system and infiltrate placental cells, complicating the development of the existing embryo or fetus. Toll-like receptors (TLRs) in the placenta are key components of the immune system, and activation of these receptors plays a role in the inflammatory response caused by airway exposure to NPs (Koga et al. Citation2014). Although recent research has yielded compelling evidence that inflammatory response and generation of ROS contribute to toxicity of NPs in male reproductive capacity, more studies are warranted to gain better insight into the mechanisms of nanotoxicity.
Figure 1. Various ways that nanoparticles (NPs) can enter into the testicular tissues through the circulatory system.
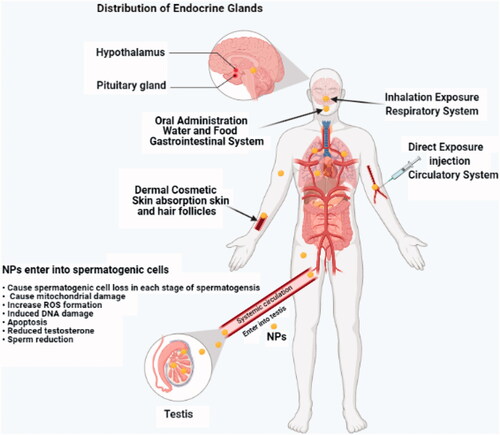
Different types of NPs are reported to induce significant cytotoxic effects on male germ cells. These effects depended on concentration and time of exposure, showing that a high concentration and time of exposure induce more toxicity. NP’s toxicity is generally induced via increased ROS generation and via inhibition of antioxidant defense systems, inducing DNA damage, and cell cycle arrest resulting in complex mechanisms with apoptosis of male germ cells (Yan et al. Citation2016) (). NPs pass through the outer membranes of gonads and generate ROS in the same way as Sertoli and Leydig cells, whose role is to support and regulation of male germ cells (Pinho, Rebelo, et al. Citation2020). The NPs toxicity mechanisms are complicated and can stimulate the generation of free radicals via causing damage of the antioxidant system and disrupting cellular metabolism (Pérez-Labrada et al. Citation2019).
Figure 2. The cytotoxic effects of NPs on male germ cells and supporting cells. Schematic summary of potential biological effects and mechanism of NP impact on the male reproductive system.
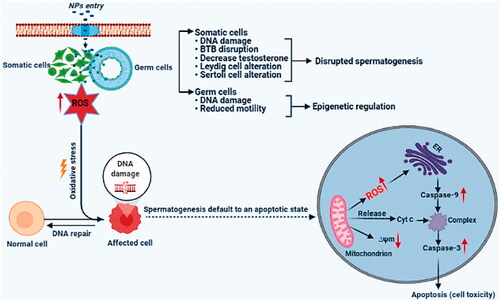
NPS induced DNA damage
NPs have been shown to induce oxidative damage to DNA, both on DNA bases and on the 2-deoxyribose moiety of the DNA backbone, thus resulting in the formation of oxidized DNA bases, single-strand breaks (SSBs) and abasic (AP) sites (Carriere et al. Citation2017). ZnO NPs average size is 40–70 nm inducing genotoxic effects on human sperm (Gopalan et al. Citation2009). Previous studies have also shown that ZnO NPs are a dose- and time-dependent cytotoxic inducer in testis and in male germ cells, resulting in increased ROS production, causing DNA damage in spermatocytes (Liu et al. Citation2016). γ-H2AX is a biomarker of cellular response to DNA double-strand breaks (DSBs) and its potential for monitoring DNA damage (Solier and Pommier Citation2014). GC-1 cell line derived from mouse testes exposed to different doses of ZnO NPs showed an increase of γ-H2AX (S139) levels (Pinho, Martins, et al. Citation2020). Titanium dioxide NPs have cytotoxic effect on buffalo spermatozoa by affecting sperm functionality and causing a DNA fragmentations (Pawar and Kaul Citation2014).
NPs induced oxidative stress
The human male reproductive system is recognized to be susceptible to many exogenous materials (Knez Citation2013). Furthermore, oxidative stress is known as one of the most vital causes of male infertility (Hussein et al. Citation2016), and is one of the main mechanisms of this deterioration (Tremellen Citation2008). Oxidative stress induced by NP exposure comprises mitochondrial respiration, mitochondrial apoptosis, alteration of calcium homeostasis, activation of the NADPH oxidase system, and depletion of antioxidant enzymes (Manke et al. Citation2013). Testis and epididymis are the most susceptible sites for NPs damage, and studies have shown that most NPs can reach the reproductive organs or tissues of male rats in different ways, such as testis and epididymis (Zhao H et al. Citation2014). NPs entering into male mice have different effects on testis, and will affect the quantity and quality of sperm after they reach target. For example, water-soluble carbon nanotubes can cause oxidative stress in the testes of male mice, thus reducing the thickness of spermatogenic epithelium (Bai et al. Citation2010). Exposure to AgNP can cause male reproductive toxicity in mammals. For example, AgNPs have been shown to affect the proliferation of SSCs by disrupting GDNF/Fyn kinase signaling in mouse (Braydich-Stolle et al. Citation2010). A study has also shown that number of spermatogenic cells was also affected by AgNPs in a rat model (Miresmaeili et al. Citation2013). A single intravenous injection of AgNPs has also been shown to induce a significant effect on germ cells, mainly affecting spermatocyte development (Han JW et al. Citation2016). In addition, daily oral exposure of prepubertal Wistar rats to AgNPs induced sperm abnormalities; included a reduction in mitochondrial activity and damage inflicted on the sperm plasma membrane and acrosome (Mathias et al. Citation2015). High dose silver intake for 25 days can reduce the integrity of acrosome sperm and plasma membrane, the activity of mitochondria and improve the rate of sperm deformity (Mathias et al. Citation2015). A study also observed that NP induced toxicity by increasing ROS levels and pro-inflammatory levels, thus leading to oxidative stress, DNA damage, and apoptosis (Khanna et al. Citation2015). Although the molecular mechanisms of this phenomenon are not entirely understood, the relationship between ROS and sperm function was much more clear, which indicated that the endogenous ROS exposure enhanced the oxidative spermatozoa DNA damage (Castillo et al. Citation2011). Other studies observed primary isolated Sertoli cell apoptosis after exposing to TiO2, and the testicular morphology was altered and reduced mice DSP (Ritz et al. Citation2011). Intratracheal injection of 200 μg carbon NPs into a mice model also resulted in histological changes in the seminiferous tubules of male offspring and reduced DSP (Yoshida et al. Citation2009). The smaller 14 nm CB has a smaller antagonistic effect than 56 nm NPs, although the particle number is similar, suggesting that NP size may be a key determinant of spermatogenesis (Bai et al. Citation2010). Another study has also shown that Au particles can penetrate sperm cells, resulting in fragmentation (Wiwanitkit et al. Citation2009). The potential spermatotoxicity of Au in industrial use has also caused male sterility and epididymitis (Manin et al. Citation2007). Exposure to ZnO NPs to the mouse Sertoli cell line (TM-4) and spermatocyte cell line (GC2-spd) has shown induced ROS generation, resulted in DNA damage, and affected the expression of BTB proteins (ZO-1, occludin, claudin-5, and connexin-43) in Sertoli cells, which could adversely affect the integrity of the BTB (Liu et al. Citation2016).
NPs induced cell apoptosis
The toxic effects caused by NPs comprise tissue inflammation and the imbalance of the cellular redox state, resulting in cell death or apoptosis (Li et al. Citation2010). A study showed that NP damaged mitochondrial and membrane functions of SSCs, induced necrosis and apoptosis (Braydich-Stolle et al. Citation2005). ZnO NPs have also been reported to potentiate apoptosis in testicular cells through DNA damage caused by ROS, with likely adverse consequences for germ cells and male fertility (Han Z et al. Citation2016). Shen et al. also found that ZnO NPs induced apoptosis through ROS formation, resulted in loss of mitochondrial membrane, which leads to the increase of apoptosis in Sertoli and Leydig cells related to nuclear DNA damage (Shen et al. Citation2019). A study showed that AgNPs cause a reduction in mitochondrial activity and lead to apoptosis and necrosis of germline stem cells (Braydich-Stolle et al. Citation2005). AgNPs have also shown a significant increase in apoptotic cells, therefore, altering the expression of P53 and BCL2 genes in cultured Sertoli cells in vitro (Habas et al. Citation2018). Moreover, NPs can induce apoptosis and autophagy in the testicular tissues via increases in the gene and protein levels of cleaved caspase-8, cleaved caspase-3, Bax, LC3-II, Atg 5, and Beclin 1, accompanied by a decrease of BCL 2 (Shen et al. Citation2019). AgNP induced changes of testis morphology, sperm production, and apoptosis-related genes and proteins including caspase-3, implying that this process was potentially related to apoptosis (Wang et al. Citation2021). Furthermore, exposure to TiO2 NPs showed up-regulation of caspase-3, Nrbp2, and cytochrome c expression, and caused a significant reduction in the antioxidant capacity of SOD, CAT, GPx, GST, GR, Cyp1b1, Car3, Acaa2, and Axud1 expression in mouse testis (Zhao X et al. Citation2014). Titanium dioxide NPs have shown that expression of apoptotic related genes including Bid, FasL, caspase-3, and p38MAPK was significantly increased in mouse testicular tissue (Orazizadeh et al. Citation2015).
NPs disrupted hormones
Hormones are essential for maintaining and influencing the development of the male reproductive system and subsequently in controlling its activities once developed. Many studies in vitro and in vivo have reported that endocrine disruptive chemicals (EDCs) can exert a number of damaging effects including prostate diseases, testicular cancer and malformed reproductive tissue, poor semen quality (low sperm counts, low ejaculate volume, high number of abnormal sperm, low number of motile sperm), and other identified abnormalities of male reproductive tissues (Knez Citation2013). Some hormones could be affected after exposure to different NPs. It has reported that intravenous administration of Ag NPs at low dose (1 mg/kg/dose) significantly increased testosterone level in male mouse serum (Zhang et al. Citation2015). Cerium oxide NPs have toxic effects on sex hormones on the sexual glands and testosterone, as the level of testosterone was increased after 70 days (Karpenko et al. Citation2013). An in vitro study showed that NPs reduced mitochondrial and membrane functions of SSCs, causing production of steroid hormones by Leydig cells (Kim et al. Citation2006). A study reported that AgNPs had altered the sex hormone concentration in the plasma and testes and the expression of genes involved in steroidogenesis and the steroids metabolism included, Star, Cyp11a1, Hsd3b1, Hsd17b3, and Srd5a1 were significantly down-regulated after male rats exposed to AgNPs/AgSPs (Dziendzikowska et al. Citation2016). Moreover, AgNPs have interruption in functions of sex hormones, and can diminish the number of Leydig cells and sperm parameter indices (Baki et al. Citation2014). Adult male rats exposed to Mn2O3 NPs showed a significant reduction in LH, follicle-stimulating hormone (FSH), and testosterone (Negahdary et al. Citation2015). However, exposure of humans to NPs can cause modulation or alterations in the cell signaling pathways processes. These NPs penetrate into the cell and disrupt normal functions. A male rat, exposure to AgNPs has shown that decreases in sperm motility, velocity, kinematic parameters, and concentrations of LH, follicle stimulating hormone, and testosterone. This finding implies that AgNP triggered hormonal imbalance and induced oxidative stress in testis and epididymis, resulting in a negative effect sperm parameters (Olugbodi et al. Citation2020). NPs cause hormonal disruption, impact fertility, and act as EDCs. EDCs disrupt the body’s normal function due to their ability to block or mimic a hormone’s natural function (Dagar and Bagchi Citation2020). A study has shown that AuNPs can accumulate in the testes and decrease testosterone production in Leydig cells via a decrease the expression of 17α-hydroxylase, consequently affecting the quality of epididymal sperm (Liu et al. Citation2020). NPs can also affect spermatogenesis and sex hormones levels and potential of spermatozoa, so influencing fertility. Since NPs may play a vital role as EDCs, future studies on the potential endocrine toxicity of NPs should be considered with regards to the age, period of exposure, and mechanisms of action of possible epigenetically mediated relating to effects.
Conclusions
In spite of these findings, the effect of NPs on the male reproductive system function is becoming an important part of nanoscience research. The in vivo studies of engineered NPs confirmed the in vitro results and indicated that the harmful effect is related to their chemical composition, size, dose, means of administration, and duration of exposure. These studies have revealed that NPs can impair mitochondrial and membrane functions of male germ stem cells, and can induce apoptosis, DNA damage, and alterations in the production of sex hormones, causing adverse effect of spermatozoa, which may cause infertility. Moreover, the adverse effect of NPs on human reproductive health is based on the chemical structure, shape, size, agglomeration state, surface area, and functionalization of NPs. These are among the factors to be considered in studying nanotoxic effects on the male germ cells and somatic cells as well as the endocrine system. It is a necessary to evaluate the underlying mechanisms that can affect male reproductive function and identify research areas where further study needs to be carried out, in order to reach a deeper understanding of the role of NPs on male germ cells and supporting somatic cells, which could cause infertility.
Disclosure statement
We declare that we have no conflict of interest.
References
- Adebayo O, Akinloye O, Adaramoye O. 2018. Cerium oxide nanoparticle elicits oxidative stress, endocrine imbalance and lowers sperm characteristics in testes of Balb/c mice. Andrologia. 50(3):e12920.
- Adler S, Basketter D, Creton S, Pelkonen O, van Benthem J, Zuang V, Andersen KE, Angers-Loustau A, Aptula A, Bal-Price A, et al. 2011. Alternative (non-animal) methods for cosmetics testing: current status and future prospects-2010. Arch Toxicol. 85(5):367–485.
- Anway MD, Skinner MK. 2008. Epigenetic programming of the germ line: effects of endocrine disruptors on the development of transgenerational disease. Reprod Biomed Online. 16(1):23–25.
- Bahadar H, Maqbool F, Niaz K, Abdollahi M. 2016. Toxicity of nanoparticles and an overview of current experimental models. Iran Biomed J. 20(1):1.
- Bai Y, Zhang Y, Zhang J, Mu Q, Zhang W, Butch ER, Snyder SE, Yan B. 2010. Repeated administrations of carbon nanotubes in male mice cause reversible testis damage without affecting fertility. Nat Nanotechnol. 5(9):683–689.
- Baki ME, Miresmaili SM, Pourentezari M, Amraii E, Yousefi V, Spenani HR, Talebi AR, Anvari M, Fazilati M, Fallah AA. 2014. Effects of silver nano-particles on sperm parameters, number of Leydig cells and sex hormones in rats. Iran J Reprod Med. 12(2):139.
- Ban Z, Zhou Q, Sun A, Mu L, Hu X. 2018. Screening priority factors determining and predicting the reproductive toxicity of various nanoparticles. Environ Sci Technol. 52(17):9666–9676.
- Barkalina N, Charalambous C, Jones C, Coward K. 2014. Nanotechnology in reproductive medicine: emerging applications of nanomaterials. Nanomedicine. 10(5):921–938.
- Ben-David Makhluf S, Qasem R, Rubinstein S, Gedanken A, Breitbart H. 2006. Loading magnetic nanoparticles into sperm cells does not affect their functionality. Langmuir. 22(23):9480–9482.
- Boisen AMZ, Shipley T, Jackson P, Hougaard KS, Wallin H, Yauk CL, Vogel U. 2012. NanoTiO2 (UV-Titan) does not induce ESTR mutations in the germline of prenatally exposed female mice. Part Fibre Toxicol. 9(1):19.
- Braydich-Stolle L, Hussain S, Schlager JJ, Hofmann M-C. 2005. In vitro cytotoxicity of nanoparticles in mammalian germline stem cells. Toxicol Sci. 88(2):412–419.
- Braydich-Stolle LK, Lucas B, Schrand A, Murdock RC, Lee T, Schlager JJ, Hussain SM, Hofmann M-C. 2010. Silver nanoparticles disrupt GDNF/Fyn kinase signaling in spermatogonial stem cells. Toxicol Sci. 116(2):577–589.
- Brohi RD, Wang L, Talpur HS, Wu D, Khan FA, Bhattarai D, Rehman ZU, Farmanullah F, Huo LJ. 2017. Toxicity of nanoparticles on the reproductive system in animal models: a review. Front Pharmacol. 8:606.
- Campos VF, de Leon PM, Komninou ER, Dellagostin OA, Deschamps JC, Seixas FK, Collares T. 2011. NanoSMGT: transgene transmission into bovine embryos using halloysite clay nanotubes or nanopolymer to improve transfection efficiency. Theriogenology. 76(8):1552–1560.
- Carriere M, Sauvaigo S, Douki T, Ravanat J-L. 2017. Impact of nanoparticles on DNA repair processes: current knowledge and working hypotheses. Mutagenesis. 32(1):203–213.
- Castillo J, Simon L, de Mateo S, Lewis S, Oliva R. 2011. Protamine/DNA ratios and DNA damage in native and density gradient centrifuged sperm from infertile patients. J Androl. 32(3):324–332.
- Chen Y, Xue Z, Zheng D, Xia K, Zhao Y, Liu T, Long Z, Xia J. 2003. Sodium chloride modified silica nanoparticles as a non-viral vector with a high efficiency of DNA transfer into cells. Curr Gene Ther. 3(3):273–279.
- Chen L, Zheng L, Lv Y, Liu H, Wang G, Ren N, Liu D, Wang J, Boughton RI. 2010. Chemical assembly of silver nanoparticles on stainless steel for antimicrobial applications. Surf Coat Technol. 204(23):3871–3875.
- Chou C-C, Hsiao H-Y, Hong Q-S, Chen C-H, Peng Y-W, Chen H-W, Yang P-C. 2008. Single-walled carbon nanotubes can induce pulmonary injury in mouse model. Nano Lett. 8(2):437–445.
- Dagar G, Bagchi G. 2020. Nanoparticles as potential endocrine disruptive chemicals. In: NanoBioMedicine. Singapore: Springer; p. 411–429.
- Del Vento F, Vermeulen M, Ucakar B, Poels J, Des Rieux A, Wyns C. 2019. Significant benefits of nanoparticles containing a necrosis inhibitor on mice testicular tissue autografts outcomes. Int J Mol Sci. 20(23):5833.
- Dziendzikowska K, Krawczyńska A, Oczkowski M, Królikowski T, Brzóska K, Lankoff A, Dziendzikowski M, Stępkowski T, Kruszewski M, Gromadzka-Ostrowska J. 2016. Progressive effects of silver nanoparticles on hormonal regulation of reproduction in male rats. Toxicol Appl Pharmacol. 313:35–46.
- Erdely A, Liston A, Salmen-Muniz R, Hulderman T, Young S-H, Zeidler-Erdely PC, Castranova V, Simeonova PP. 2011. Identification of systemic markers from a pulmonary carbon nanotube exposure. J Occup Environ Med. 53(6 Suppl.):S80–S86.
- Gao G, Ze Y, Zhao X, Sang X, Zheng L, Ze X, Gui S, Sheng L, Sun Q, Hong J, et al. 2013. Titanium dioxide nanoparticle-induced testicular damage, spermatogenesis suppression, and gene expression alterations in male mice. J Hazard Mater. 258–259:133–143.
- Golobič M, Jemec A, Drobne D, Romih T, Kasemets K, Kahru A. 2012. Upon exposure to Cu nanoparticles, accumulation of copper in the isopod Porcellio scaber is due to the dissolved Cu ions inside the digestive tract. Environ Sci Technol. 46(21):12112–12119.
- Gopalan RC, Osman IF, Amani A, De Matas M, Anderson D. 2009. The effect of zinc oxide and titanium dioxide nanoparticles in the Comet assay with UVA photoactivation of human sperm and lymphocytes. Nanotoxicology. 3(1):33–39.
- Gromadzka-Ostrowska J, Dziendzikowska K, Lankoff A, Dobrzyńska M, Instanes C, Brunborg G, Gajowik A, Radzikowska J, Wojewódzka M, Kruszewski M. 2012. Silver nanoparticles effects on epididymal sperm in rats. Toxicol Lett. 214(3):251–258.
- Habas K, Brinkworth MH, Anderson D. 2018. Silver nanoparticle-mediated cellular responses in isolated primary Sertoli cells in vitro. Food Chem Toxicol. 116(Pt B):182–188.
- Habas K, Brinkworth MH, Anderson D. 2020. A male germ cell assay and supporting somatic cells: its application for the detection of phase specificity of genotoxins in vitro. J Toxicol Environ Health B Crit Rev. 23(3):91–106.
- Han JW, Jeong J-K, Gurunathan S, Choi Y-J, Das J, Kwon D-N, Cho S-G, Park C, Seo HG, Park J-K, et al. 2016. Male- and female-derived somatic and germ cell-specific toxicity of silver nanoparticles in mouse. Nanotoxicology. 10(3):361–373.
- Han Z, Yan Q, Ge W, Liu Z-G, Gurunathan S, De Felici M, Shen W, Zhang X-F. 2016. Cytotoxic effects of ZnO nanoparticles on mouse testicular cells. Int J Nanomedicine. 11:5187–5203.
- Hansson GC. 2012. Role of mucus layers in gut infection and inflammation. Curr Opin Microbiol. 15(1):57–62.
- Hong F, Li W, Ji J, Ze X, Diao E. 2020. Nanostructured titanium dioxide (TiO(2)) reduces sperm concentration involving disorder of meiosis and signal pathway. J Biomed Nanotechnol. 16(5):659–671.
- Hong F, Wang Y, Zhou Y, Zhang Q, Ge Y, Chen M, Hong J, Wang L. 2016. Exposure to TiO2 nanoparticles induces immunological dysfunction in mouse testitis. J Agric Food Chem. 64(1):346–355.
- Hong F, Zhao X, Si W, Ze Y, Wang L, Zhou Y, Hong J, Yu X, Sheng L, Liu D, et al. 2015. Decreased spermatogenesis led to alterations of testis-specific gene expression in male mice following nano-TiO2 exposure. J Hazard Mater. 300:718–728.
- Hozyen HF, Khalil HMA, Ghandour RA, Al-Mokaddem AK, Amer MS, Azouz RA. 2020. Nano selenium protects against deltamethrin-induced reproductive toxicity in male rats. Toxicol Appl Pharmacol. 408:115274.
- Huang C, Li B, Xu K, Liu D, Hu J, Yang Y, Nie H, Fan L, Zhu W. 2017. Decline in semen quality among 30,636 young Chinese men from 2001 to 2015. Fertil Steril. 107(1):83–88.e2.
- Hussein MM, Ali HA, Saadeldin IM, Ahmed MM. 2016. Querectin alleviates zinc oxide nanoreprotoxicity in male albino rats. J Biochem Mol Toxicol. 30(10):489–496.
- Iavicoli I, Fontana L, Leso V, Bergamaschi A. 2013. The effects of nanomaterials as endocrine disruptors. Int J Mol Sci. 14(8):16732–16801.
- Iona S, Klinger F, Sisti R, Ciccalese R, Nunziata A, De Felici M. 2002. A comparative study of cytotoxic effects of N-ethyl-N-nitrosourea, adriamycin, and mono-2-ethylhexyl) phthalate on mouse primordial germ cells. Cell Biol Toxicol. 18(2):131–145.
- Karimi S, Khorsandi L, Nejaddehbashi F. 2019. Protective effects of curcumin on testicular toxicity induced by titanium dioxide nanoparticles in mice. JBRA Assist Reprod. 23(4):344–351.
- Kashiwada S. 2006. Distribution of nanoparticles in the see-through medaka (Oryzias latipes). Environ Health Perspect. 114(11):1697–1702.
- Khan I, Saeed K, Khan I. 2019. Nanoparticles: properties, applications and toxicities. Arab J Chem. 12(7):908–931.
- Khanna P, Ong C, Bay BH, Baeg GH. 2015. Nanotoxicity: an interplay of oxidative stress, inflammation and cell death. Nanomaterials (Basel). 5(3):1163–1180.
- Kim D, Jeong YY, Jon S. 2010. A drug-loaded aptamer-gold nanoparticle bioconjugate for combined CT imaging and therapy of prostate cancer. ACS Nano. 4(7):3689–3696.
- Kim JS, Yoon T-J, Yu KN, Kim BG, Park SJ, Kim HW, Lee KH, Park SB, Lee J-K, Cho MH. 2006. Toxicity and tissue distribution of magnetic nanoparticles in mice. Toxicol Sci. 89(1):338–347.
- Knez J. 2013. Endocrine-disrupting chemicals and male reproductive health. Reprod Biomed Online. 26(5):440–448.
- Koga K, Izumi G, Mor G, Fujii T, Osuga Y. 2014. Toll-like receptors at the maternal-fetal interface in normal pregnancy and pregnancy complications. Am J Reprod Immunol. 72(2):192–205.
- Kong L, Gao X, Zhu J, Cheng K, Tang M. 2016. Mechanisms involved in reproductive toxicity caused by nickel nanoparticle in female rats. Environ Toxicol. 31(11):1674–1683.
- Kong L, Hu W, Gao X, Wu Y, Xue Y, Cheng K, Tang M. 2019. Molecular mechanisms underlying nickel nanoparticle induced rat Sertoli-germ cells apoptosis. Sci Total Environ. 692:240–248.
- Lan Z, Yang W-X. 2012. Nanoparticles and spermatogenesis: how do nanoparticles affect spermatogenesis and penetrate the blood–testis barrier. Nanomedicine (Lond). 7(4):579–596.
- Leclerc L, Klein J-P, Forest V, Boudard D, Martini M, Pourchez J, Blanchin M-G, Cottier M. 2015. Testicular biodistribution of silica-gold nanoparticles after intramuscular injection in mice. Biomed Microdev. 17(4):66.
- Lee J-H, Huh Y-M, Jun Y-w, Seo J-w, Jang J-t, Song H-T, Kim S, Cho E-J, Yoon H-G, Suh J-S, et al. 2007. Artificially engineered magnetic nanoparticles for ultra-sensitive molecular imaging. Nat Med. 13(1):95–99.
- Lewinski N, Colvin V, Drezek R. 2008. Cytotoxicity of nanoparticles. Small. 4(1):26–49.
- Li P-W, Kuo T-H, Chang J-H, Yeh J-M, Chan W-H. 2010. Induction of cytotoxicity and apoptosis in mouse blastocysts by silver nanoparticles. Toxicol Lett. 197(2):82–87.
- Li X, Yang X, Yuwen L, Yang W, Weng L, Teng Z, Wang L. 2016. Evaluation of toxic effects of CdTe quantum dots on the reproductive system in adult male mice. Biomaterials. 96:24–32.
- Lipskind ST, Gargiulo AR. 2013. Computer-assisted laparoscopy in fertility preservation and reproductive surgery. J Minim Invasive Gynecol. 20(4):435–445.
- Liu Y, Li X, Xiao S, Liu X, Chen X, Xia Q, Lei S, Li H, Zhong Z, Xiao K. 2020. The effects of gold nanoparticles on Leydig cells and male reproductive function in mice. Int J Nanomedicine. 15:9499–9514.
- Liu Q, Xu C, Ji G, Liu H, Mo Y, Tollerud DJ, Gu A, Zhang Q. 2016. Sublethal effects of zinc oxide nanoparticles on male reproductive cells. Toxicol In Vitro. 35:131–138.
- Lopes IMD, de Oliveira IM, Bargi-Souza P, Cavallin MD, Kolc CSM, Khalil NM, Quináia SP, Romano MA, Romano RM. 2019. Effects of silver nanoparticle exposure to the testicular antioxidant system during the prepubertal rat stage. Chem Res Toxicol. 32(6):986–994.
- Mahfouz R, Sharma R, Thiyagarajan A, Kale V, Gupta S, Sabanegh E, Agarwal A. 2010. Semen characteristics and sperm DNA fragmentation in infertile men with low and high levels of seminal reactive oxygen species. Fertil Steril. 94(6):2141–2146.
- Manin O, Nikolaev V, Kolomiĭtsev A, Lebedenko I. 2007. Comparative toxicological evaluation of domestic golden alloys for soldering. Stomatologiia (Mosk). 86(1):64–67.
- Manke A, Wang L, Rojanasakul Y. 2013. Mechanisms of nanoparticle-induced oxidative stress and toxicity. Biomed Res Int. 2013:942916.
- Massarsky A, Trudeau VL, Moon TW. 2014. Predicting the environmental impact of nanosilver. Environ Toxicol Pharmacol. 38(3):861–873.
- Mathias FT, Romano RM, Kizys MM, Kasamatsu T, Giannocco G, Chiamolera MI, Dias-da-Silva MR, Romano MA. 2015. Daily exposure to silver nanoparticles during prepubertal development decreases adult sperm and reproductive parameters. Nanotoxicology. 9(1):64–70.
- Miresmaeili SM, Halvaei I, Fesahat F, Fallah A, Nikonahad N, Taherinejad M. 2013. Evaluating the role of silver nanoparticles on acrosomal reaction and spermatogenic cells in rat. Iran J Reprod Med. 11(5):423–430.
- Mohammad I. 2019. Gold nanoparticle: an efficient carrier for MCP I of Carica papaya seeds extract as an innovative male contraceptive in albino rats. J Drug Delivery Sci Technol. 52:942–956.
- Morgan AM, Ibrahim MA, Noshy PA. 2017. Reproductive toxicity provoked by titanium dioxide nanoparticles and the ameliorative role of Tiron in adult male rats. Biochem Biophys Res Commun. 486(2):595–600.
- Morishita Y, Yoshioka Y, Satoh H, Nojiri N, Nagano K, Abe Y, Kamada H, Tsunoda S-i, Nabeshi H, Yoshikawa T, et al. 2012. Distribution and histologic effects of intravenously administered amorphous nanosilica particles in the testes of mice. Biochem Biophys Res Commun. 420(2):297–301.
- Negahdary M, Arefian Z, Dastjerdi HA, Ajdary M. 2015. Toxic effects of Mn2O3 nanoparticles on rat testis and sex hormone. J Nat Sci Biol Med. 6(2):335–339.
- Nirmal NK, Awasthi KK, John PJ. 2017. Effects of nano-graphene oxide on testis, epididymis and fertility of wistar rats. Basic Clin Pharmacol Toxicol. 121(3):202–210.
- Niska K, Zielinska E, Radomski MW, Inkielewicz-Stepniak I. 2018. Metal nanoparticles in dermatology and cosmetology: interactions with human skin cells. Chem Biol Interact. 295:38–51.
- Olugbodi JO, David O, Oketa EN, Lawal B, Okoli BJ, Mtunzi F. 2020. Silver nanoparticles stimulates spermatogenesis impairments and hematological alterations in testis and epididymis of male rats. Molecules. 25(5):1063.
- Ong C, Lee QY, Cai Y, Liu X, Ding J, Yung L-YL, Bay B-H, Baeg G-H. 2016. Silver nanoparticles disrupt germline stem cell maintenance in the Drosophila testis. Sci Rep. 6:20632.
- Orazizadeh M, Daneshi E, Hashemitmar M, Absalan F, Khorsandi L. 2015. Protective effect of beta‐carotene against titanium dioxide nanoparticles induced apoptosis in mouse testicular tissue. Andrologia. 47(7):816–825.
- Pawar K, Kaul G. 2014. Toxicity of titanium oxide nanoparticles causes functionality and DNA damage in buffalo (Bubalus bubalis) sperm in vitro. Toxicol Ind Health. 30(6):520–533.
- Pérez-Labrada F, López-Vargas ER, Ortega-Ortiz H, Cadenas-Pliego G, Benavides-Mendoza A, Juárez-Maldonado A. 2019. Responses of tomato plants under saline stress to foliar application of copper nanoparticles. Plants. 8(6):151.
- Pinho AR, Martins F, Costa MEV, Senos AM, Silva OA, Pereira ML, Rebelo S. 2020. In vitro cytotoxicity effects of zinc oxide nanoparticles on spermatogonia cells. Cells. 9(5):1081.
- Pinho AR, Rebelo S, Pereira ML. 2020. The impact of zinc oxide nanoparticles on male (In) fertility. Materials. 13(4):849.
- Rafiee Z, Khorsandi L, Nejad-Dehbashi F. 2019. Protective effect of zingerone against mouse testicular damage induced by zinc oxide nanoparticles. Environ Sci Pollut Res Int. 26(25):25814–25824.
- Ritz C, Ruminski W, Hougaard KS, Wallin H, Vogel U, Yauk CL. 2011. Germline mutation rates in mice following in utero exposure to diesel exhaust particles by maternal inhalation. Mutat Res. 712(1–2):55–58.
- Santonastaso M, Mottola F, Colacurci N, Iovine C, Pacifico S, Cammarota M, Cesaroni F, Rocco L. 2019. In vitro genotoxic effects of titanium dioxide nanoparticles (n‐TiO2) in human sperm cells. Mol Reprod Dev. 86(10):1369–1377.
- Shalviri A, Cai P, Rauth AM, Henderson JT, Wu XY. 2012. Evaluation of new bi-functional terpolymeric nanoparticles for simultaneous in vivo optical imaging and chemotherapy of breast cancer. Drug Deliv Transl Res. 2(6):437–453.
- Shen J, Yang D, Zhou X, Wang Y, Tang S, Yin H, Wang J, Chen R, Chen J. 2019. Role of autophagy in zinc oxide nanoparticles-induced apoptosis of mouse LEYDIG cells. Int J Mol Sci. 20(16):4042.
- Sleiman HK, Romano RM, Oliveira CA, Romano MA. 2013. Effects of prepubertal exposure to silver nanoparticles on reproductive parameters in adult male Wistar rats. J Toxicol Environ Health A. 76(17):1023–1032.
- Solier S, Pommier Y. 2014. The nuclear γ-H2AX apoptotic ring: implications for cancers and autoimmune diseases. Cell Mol Life Sci. 71(12):2289–2297.
- Takeda K, Suzuki K-i, Ishihara A, Kubo-Irie M, Fujimoto R, Tabata M, Oshio S, Nihei Y, Ihara T, Sugamata M. 2009. Nanoparticles transferred from pregnant mice to their offspring can damage the genital and cranial nerve systems. J Health Sci. 55(1):95–102.
- Tang Y, Chen B, Hong W, Chen L, Yao L, Zhao Y, Aguilar ZP, Xu H. 2019. ZnO nanoparticles induced male reproductive toxicity based on the effects on the endoplasmic reticulum stress signaling pathway. Int J Nanomedicine. 14:9563–9576.
- Taylor U, Barchanski A, Garrels W, Klein S, Kues W, Barcikowski S, Rath D. 2012. Toxicity of gold nanoparticles on somatic and reproductive cells. Adv Exp Med Biol. 733:125–133.
- Karpenko NA, Malukin YV, Koreneva EM, Klochkov VK, Kavok NS, Smolenko NP, Pochernyaeva SS. 2013. The effects of chronic intake of nanoparticles of cerium dioxide or gadolinium ortovanadate into aging male rats. Proceedings of the International Conference Nanomaterials: Applications and Properties; Sumy State University Publishing.
- Tremellen K. 2008. Oxidative stress and male infertility—a clinical perspective. Hum Reprod Update. 14(3):243–258.
- Wang E, Huang Y, Du Q, Sun Y. 2021. Alterations in reproductive parameters and gene expression in Balb/c mice testes after exposure to silver nanoparticles. Andrologia. 53(1):e13841.
- Wang R, Song B, Wu J, Zhang Y, Chen A, Shao L. 2018. Potential adverse effects of nanoparticles on the reproductive system. Int J Nanomedicine. 13:8487–8506.
- Wang AZ, Bagalkot V, Vasilliou CC, Gu F, Alexis F, Zhang L, Shaikh M, Yuet K, Cima MJ, Langer R, et al. 2008. Superparamagnetic iron oxide nanoparticle–aptamer bioconjugates for combined prostate cancer imaging and therapy. ChemMedChem. 3(9):1311–1315.
- Wells PG, Bhuller Y, Chen CS, Jeng W, Kasapinovic S, Kennedy JC, Kim PM, Laposa RR, McCallum GP, Nicol CJ, et al. 2005. Molecular and biochemical mechanisms in teratogenesis involving reactive oxygen species. Toxicol Appl Pharmacol. 207(2 Suppl.):354–366.
- Wiwanitkit V, Sereemaspun A, Rojanathanes R. 2009. Effect of gold nanoparticles on spermatozoa: the first world report. Fertil Steril. 91(1):e7–e8.
- Xia X, Wang L, Yang X, Hu Y, Liu Q. 2020. Acute damage to the sperm quality and spermatogenesis in male mice exposed to curcumin-loaded nanoparticles. Int J Nanomedicine. 15:1853–1862.
- Xu Y, Wang N, Yu Y, Li Y, Li YB, Yu YB, Zhou XQ, Sun ZW. 2014. Exposure to silica nanoparticles causes reversible damage of the spermatogenic process in mice. PLoS One. 9(7):e101572.
- Yan S-Q, Xing R, Zhou Y-F, Li K-L, Su Y-Y, Qiu J-F, Zhang Y-H, Zhang K-Q, He Y, Lu X-P, et al. 2016. Reproductive toxicity and gender differences induced by cadmium telluride quantum dots in an invertebrate model organism. Sci Rep. 6(1):34182.
- Yauk C, Polyzos A, Rowan-Carroll A, Somers CM, Godschalk RW, Van Schooten FJ, Berndt ML, Pogribny IP, Koturbash I, Williams A, et al. 2008. Germ-line mutations, DNA damage, and global hypermethylation in mice exposed to particulate air pollution in an urban/industrial location. Proc Natl Acad Sci U S A. 105(2):605–610.
- Yoshida S, Hiyoshi K, Ichinose T, Takano H, Oshio S, Sugawara I, Takeda K, Shibamoto T. 2009. Effect of nanoparticles on the male reproductive system of mice. Int J Androl. 32(4):337–342.
- Yoshida S, Hiyoshi K, Oshio S, Takano H, Takeda K, Ichinose T. 2010. Effects of fetal exposure to carbon nanoparticles on reproductive function in male offspring. Fertil Steril. 93(5):1695–1699.
- Zhang XF, Gurunathan S, Kim JH. 2015. Effects of silver nanoparticles on neonatal testis development in mice. Int J Nanomedicine. 10:6243–6256.
- Zhang X, Yue Z, Zhang H, Liu L, Zhou X. 2020. Repeated administrations of Mn3O4 nanoparticles cause testis damage and fertility decrease through PPAR-signaling pathway. Nanotoxicology. 14(3):326–340.
- Zhang D, Zhang Z, Wu Y, Fu K, Chen Y, Li W, Chu M. 2019. Systematic evaluation of graphene quantum dot toxicity to male mouse sexual behaviors, reproductive and offspring health. Biomaterials. 194:215–232.
- Zhao H, Gu W, Ye L, Yang H. 2014. Biodistribution of PAMAM dendrimer conjugated magnetic nanoparticles in mice. J Mater Sci Mater Med. 25(3):769–776.
- Zhao X, Sheng L, Wang L, Hong J, Yu X, Sang X, Sun Q, Ze Y, Hong F. 2014. RETRACTED ARTICLE: mechanisms of nanosized titanium dioxide-induced testicular oxidative stress and apoptosis in male mice. Part Fibre Toxicol. 11(1):47.