Abstract
Methotrexate (MTX), a stoichiometric inhibitor of dihydrofolate reductase enzyme, is a chemotherapeutic agent for treating a diversity of neoplasms. In this study, we design and developed a new formulation of MTX that serves as drug carrier and examined its cytotoxic effect in vitro. This target drug delivery system is dependent on the release of the MTX within the lysosomal compartment. The iron oxide magnetic nanoparticles (IONPs) were first surface-coated with L-lysine and subsequently conjugated with MTX through amidation between the carboxylic acid end groups on MTX and the amine groups on the IONPs surface. MTX-conjugated L-lysine coated IONPs (F-Lys-MTX NPs) was characterized by X-ray diffraction, thermogravimetric analysis, differential scanning calorimetry, Fourier transform infrared spectroscopy, vibrating sample magnetometer, and transmission electron microscopy techniques. The cytotoxicity of the void of MTX and F-Lys-MTX NPs were compared to each other by MTT assay of the treated MCF-7 cell lines. The results showed that the ζ-potential of F-Lys-MTX NPs was about −5.49 mV and the average size was 43.72 ± 4.73 nm. Model studies exhibited the release of MTX via peptide bond cleavage in the presence of proteinase K and at low pH. These studies specify that F-Lys-MTX NPs have a very remarkable anticancer effect, for breast cancer cell lines.
Introduction
Cancer is one of the principal causes of morbidity and mortality in the world [Citation1]. Cancer is the second leading cause of death globally, and was responsible for 8.8 million deaths in 2015. Globally, nearly 1 in 6 deaths is due to cancer. Approximately 70% of deaths from cancer occur in low- and middle-income countries. Around one-third of deaths from cancer are due to the 5 leading behavioral and dietary risks: high body mass index, low fruit and vegetable intake, lack of physical activity, tobacco use, and alcohol use [Citation2]. Cancer can be treated by surgery, chemotherapy, radiation therapy, hormonal therapy, targeted therapy, and synthetic lethality. One of the main obstacles in chemotherapy of cancer is the biodistribution of the chemotherapeutic compound, which can lead to severe off-target side effects and toxicities [Citation3]. To address these limitations, an attractive method is to design a carrier system that can selectively deliver cytotoxic doses of therapeutic agents to cancer [Citation4–7]. Among the nanocarriers that used for drug delivery and cancer therapy [Citation8–12] iron oxide magnetic nanoparticles (IONPs) have attracted considerable attention in drug delivery systems [Citation13]. We have become interested in the development of amino acid coated IONPs for biological applications especially cancer studies [Citation14]. Cellular uptake of NPs can be improved by immobilizing an ‘address’ chemical or biological reagents including antibodies or low molecular weight targeting agents on the surface of a carrier, which can be recognized by a receptor overexpressed on tumor cells [Citation15]. Nevertheless, selection of specific targeting agents or drugs that can be effectively released from carrier inside target cells remains a challenge and is the central focus of the current studies in the field [Citation16–18]. An attractive receptor for tumor targeting is folate. Folate receptors are overexpressed in the cell membranes of many cancer cells [Citation19–21]. Folic acid (FA) has a small size, and thus facilitates the internalization of nanocarrier. FA is small, stable, nonimmunogenic, inexpensive and, in addition, has a very high affinity for its cell surface receptors [Citation21]. Covalent bonding of therapeutic agents on nanocarriers is usually favored because the bond strength makes nanoparticle drug conjugates highly stable and therefore is most likely to be disrupted only under harsh environments inside lysosomes. Methotrexate (MTX), an analog of FA, disturbs cellular folate metabolism by inhibiting dihydrofolate reductase (DHFR), its target enzyme [Citation22]. MTX shows not only a targeting role for folate receptors but also a therapeutic effect to many types of cancer cells that overexpress folate receptors on their surfaces [Citation23]. Although the MTX has been conjugated to big particles (∼20 μm) can facilitate its uptake by tumor cells, provide sustained and kinetic releases of MTX and improve its therapeutic efficacy [Citation24]. However, the large size of the conjugates may limit their applications only to direct intratumoral administration. Thus, to be effective, the drug carrier must be appropriately small to penetrate the vessels, perfuse out of the bloodstream, and set foot on the target cell of interest. MTX was conjugated with IONPs as a drug delivery system to study its cytotoxic effect on cancer cells [Citation25–27]. MTX-imbedded nanoparticles were believed to be taken up into cells to a more degree by the human folate receptor than free MTX [Citation28]. It is well known that the coated molecules on IONPs surface act as the highest interface between the IONPs and the body [Citation29]. In order to, many different types of materials and strategies can be used to surface coating of IONPs [Citation30–32]. Previously, we reported the synthesis and characterization of L-lysine coated IONPs (F-Lys NPs) [Citation14]. In this study, we developed a MTX-conjugated L-lysine coated IONPs (F-Lys-MTX NPs). Covalently modifying the surface of the F-Lys NPs via a peptide bond, MTX is not released from the surface of the nanoparticles under intravenous conditions. Instead, cleavage of the amide bond happens under conditions present in the lysosomal compartment, specifically, at low pH and in the presence of lysozymes, a typical environment inside the target cells. Due to the overexpressed folate receptor on target cancer cells as opposed to healthy normal cells, this release mechanism will greatly reduce toxic effects of MTX to healthy tissues within the body.
Experimental section
Materials
L-Lysine, FeCl3·6H2O and FeCl2·4H2O were purchased from Merck (Darmstadt, Germany). 1-Ethyl-3-[3-(dimethylamino) propyl] carbodiimide (EDC), N-hydroxysuccinimide (NHS), and 3–(4, 5-dimethylthiazol-2-yl)-2, 5-diphenyltetrazolium bromide (MTT) were obtained from Sigma-Aldrich (St. Louis, MO, USA). Ammonium hydroxide (25 wt % NH3 in water (NH4OH)) and acetone were purchased from Mojallali Company, Iran. Methotrexate sodium (MTX) was purchased from Zahravi Company, Iran. All other chemicals and solvents were from the general lab or HPLC grades, purchased locally.
Synthesis of L-lysine coated IONPs (F-Lys NPs)
F-Lys NPs were synthesized by the one pot synthesis method [Citation14]. About 1.11 g of ferric chloride hexahydrate (FeCl3·6H2O) and 0.4 g of ferrous chloride tetrahydrate (FeCl2·4H2O) (molar ratio 2:1, respectively) were dissolved in 150 ml deionized (DI) water, and the mixture was stirred vigorously (12,000 rpm) under N2 atmosphere at 60 °C for 15 min. After that, 1.6 g of L-lysine rapidly added to the mixture and the reaction was stirred for another 20 min. Then under vigorous stirring and N2 gas, 20 ml NH4OH solution (25 wt % NH3 in water) was drop-wise added till the pH was raised to ∼11 at which a black suspension was formed and stirring continued for 6 h. Prepared nanoparticles were separated magnetically (magnetic separation was carried out for 5 min using an external magnet (1.2 Tesla)), washed with distilled water four times, and dried in an oven at 50 °C overnight.
Synthesis of MTX-conjugated L-lysine coated IONPs (F-Lys-MTX NPs)
To conjugate the MTX on the surface of F-Lys NPs, 100 mg F-Lys NPs was dispersed in 15 ml of DMSO. Then, the suspension of F-Lys NPs was mixed with 25 mg free MTX, 52.5 mg EDC and 31.6 mg NHS. The pH of the solution was adjusted to 8.2 by addition of triethylamine. The resulting suspension was stirred overnight (20 h) at room temperature (∼30 °C) in the dark. Following MTX conjugation, the modified nanoparticles were again isolated with an external magnet (magnetic separation was carried out for 10 min using an external magnet (1.2 Tesla)) and washed five times with DI water.
Characterization of the F-Lys-MTX NPs
FTIR analysis
The chemical structure of samples was known by Fourier transform infrared spectroscopy (FTIR) (Bruker, Tensor 27). Standard disks were prepared by mixing 2 mg of selected samples with 200 mg of KBr medium by grinding and then compressing the resulting powder into transparent pellets (pressure, 12 Ton). The FTIR spectra of the KBr disks were recorded using the aforementioned instrument from 400 to 4000 cm−1. Each spectrum was obtained using 16 scans.
Colloidal stability
The nanoparticles ζ-potential was determined by dynamic light scattering using a nano/zetasizer (Malvern Instruments, Worcestershire, UK, model Nano ZS). Particles were analyzed in phosphate-buffered saline (PBS, pH =7.4). Each spectrum was obtained using 25 scans.
Thermal analysis
Differential scanning calorimetry (DSC) (Mettler Toledo, model Star SW 9.30, Schwerzenbach, Switzerland) was applied for thermal analysis of the nanoparticles. Samples were heated at a rate of 15 °C min−1 and the analysis was recorded from 30 to 300 °C.
Also, the thermal stability was determined by thermogravimetric analysis (TGA, Linseis Instruments model, STA PT 1000, USA). The TGA thermograms were recorded at a heating rate of 10 °C min−1 in the temperature range of 30–500 °C min−1 under the N2 atmosphere.
Determination of particle size
The morphology and size of the samples were confirmed by the transmission electron microscopy (TEM; Cambridge 360–1990 Stereo Scan Instrument-EDS).
Structure characterization
The X-ray diffraction (XRD) patterns were recorded with a Bruker AXS model D8 Advance diffractometer using Cu Ka radiation (k = 1.542 A) with the Bragg angle ranging from 10° to 90°.
Magnetic properties
Sample magnetization curves were obtained using a vibrating sample magnetometer (VSM) (VSM Megnetic Daghigh Daneshpajouh Co, Kashan, Iran) at 300 K under a reciprocating altered magnetic field (−10,000 to 10,000 Oe), and saturation magnetization was obtained from hysteresis loops.
Quantification of MTX conjugated on the F-Lys NPs
UV–Vis spectroscopy was used to determine the amount of MTX conjugated on the F-Lys NPs surface. F-Lys-MTX NPs (2.3 mg) and Proteinase K enzyme (2.3 mg) were dispersed in 1 ml of PBS (pH =7.4). The resulting suspension was placed within a dialysis sac (Mw 12 kDa) and incubated at 37 °C while immersed in 5 ml of PBS (pH =7.4). Then, the concentration of MTX in the dialysate was measured at 304 nm using an UV–Vis spectrophotometer and compared to the standard curve produced previously.
Drug release study
Drug release study was performed to evaluate the release behavior of MTX from F-Lys-MTX NPs. Briefly, 5 mg of F-Lys-MTX NPs and Proteinase K enzyme (5 mg) were dispersed in 1 ml PBS (pH =5.4) and the resulting suspension was placed within a dialysis sac (Mw 12 kDa) and incubated at 37 °C while immersed in 10 ml of PBS (pH =5.4). Then, at predetermined time intervals, 2 ml of the dialysate was taken out and replaced by 2 ml fresh PBS. The concentration of MTX in the dialysate was measured at 304 nm using an UV–Vis spectrophotometer.
In vitro cytotoxicity and anticancer activity
Cell viability against pure MTX and F-Lys-MTX NPs were studied by MTT assay test on MCF-7 cell lines. Briefly, MCF-7 cell lines were seeded in 96-well plate and continued to incubate for 24 h in culture medium (DMEM) supplement with 10% fetal bovine serum, 1% antibody containing penicillin and streptomycin at 37 °C and in an atmosphere of 5% CO2 for cell adhesion. After 24 h incubation, the cells were treated with 100 µL fresh culture medium per well containing F-Lys-MTX NPs with different MTX concentration in triplicate. After the cells were incubated for 48 and 72 h, the medium was removed and washed with PBS, respectively. MTT solution was added into each well and the cells were incubated for another 4 h. Then the cell medium was discarded, and 100 µl of DMSO was added to per well to dissolve the crystals of dye. After mild shaking for 15 min, the absorbance ratio of the number of surviving cells in F-Lys-MTX NPs treated samples was compared with the control. The cytotoxicity of pure MTX was obtained by the same procedure.
Results and discussion
The goal of this project was to synthesize and characterize of IONPs for delivery of MTX into cancer cells through covalent bonding interactions. In order to formation of amid bond as a covalent linkage L-lysine was used to coat of IONPs and react with the MTX. Finally, we compared their anticancer efficacy on MCF-7 human breast cancer cells which are known to have folate-receptors overexpressed on their cell membranes.
Synthesis of MTX-conjugated L-lysine coated IONPs (F-Lys-MTX NPs)
The F-Lys NPs were synthesized by controlled co-precipitation of Fe (II) and Fe (III) ions according to the previously reported method [Citation14]. The F-Lys NPs afterward conjugated with MTX by covalent binding via carbodiimide chemistry (. Although the MTX conjugation may occur through either α or γ carboxylic acid groups on the glutamic acid residue. MTX favored the linkage with the distal γ-carboxyl residue with the L-lysine amino group on particles surfaces which was similar to FA conjugation manner reported in the literature [Citation33,Citation34]. The amount of MTX conjugated on the F-Lys NPs surface is 8.9%.
Characterization of the F-Lys-MTX NPs
FTIR analysis
FTIR was used to confirm that L-lysine and MTX were successfully conjugated on the nanoparticles. FTIR spectra of IONPs, pure L-Lysine, F-Lys NPs, pure MTX, and F-Lys-MTX NPs are shown in . For the bare IONPs (), the peak at 610 cm−1 and 451 cm−1 relates to Fe–O group [Citation35]. For nanoparticles coated with L-lysine (), the peaks at ∼3428, 2922, 1628, and 1388 cm−1 related to N-H stretching vibration overlaps with OH stretching, C–H stretching of methylene groups, C = O and C-O stretching vibrations, respectively. shows the characteristic FTIR absorption peaks of Pure MTX in 1644 and 1603 cm−1. Besides, Spectra of nanoparticles modified with MTX following L-lysine conjugation show increased absorbance at 1620 and 1560 cm−1 [Citation25,Citation36]. In addition, spectra of F-Lys-MTX NPs show appearance of a new peak at 1200, 1561, and 3200 cm−1 indicative of the presence of MTX on the nanoparticle through amide bonds within the MTX structure and amide bonding between L-lysine and MTX (). Also, F-Lys-MTX NPs also exhibit a strong peak at 606 and 447 cm−1 which are assigned to the Fe–O vibration [Citation29].
Colloidal stability
The presence of MTX on the nanoparticles surface was further confirmed with the change of surface charge. ζ-Potential of synthesized F-Lys NPs was negatively charged (ζ-potential = −19.3 mV). Following the conjugation of MTX, the ζ-potential of nanoparticles decreased (ζ-potential = −5.49 mV) due to the conjugation of the nanoparticles surface amine groups with MTX.
Thermal analysis
DSC can also provide supportive evidence for the synthesis of the F-Lys-MTX NPs. shows thermograms of pure L-lysine, pure MTX, bare IONPs, F-Lys NPs, and F-Lys-MTX NPs. As indicated in melting points (dec.) of L-lysine shifted to a lower temperature after coating on IONPs surface, which confirms the covering of IONPs with L-lysine. In thermograms, the melting points of MTX and F-Lys NPs appeared at 153.22 °C and 203.86 °C, respectively, whereas the DSC thermogram of F-Lys-MTX NPs exhibited a single endothermic peak at 249.79 °C. As it is clear, there was no peak visible near the MTX and F-Lys NPs melting point for the F-Lys-MTX NPs thermogram, which confirms the formation of conjugate and absence of any unconjugated MTX in the product.
Figure 3. DSC thermograms of (A) bare Fe3O4, (B) pure L-Lysine, (C) F-Lys NPs, (D) F-Lys-MTX NPs, and (E) pure MTX.
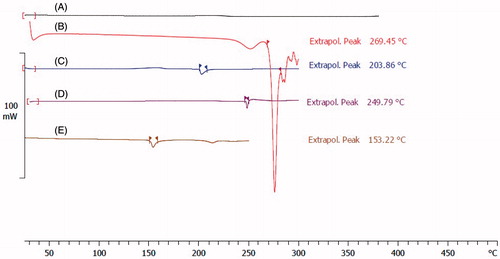
shows the TGA curves of the F-Lys and F-Lys-MTX nanoparticles. The TGA curves of the nanoparticles provide more evidence on the organic content of the nanoparticles. About 5.71% weight loss is observed after heating F-Lys NPs to 500 °C in N2. This weight loss can be attributed to the evaporation of adsorbed water, the decomposition of organic layer [Citation27]. After MTX grafting, the F-Lys-MTX NPs exhibit a much higher weight loss (about 18.25%), which is reliable with the increase in organic content of the nanoparticles upon grafting of MTX.
Determination of particle size
shows the TEM studies and size distribution measured from TEM images of F-Lys-MTX NPs. As shown in the images, nanoparticles are dispersed, have a globular shape and narrow size distribution with the average size of 43.72 ± 4.73 (mean ± SD) nm.
Structure characterization
The X-ray powder diffraction pattern is presented in . The position and relative intensity of all characteristic diffraction peaks of the F-Lys-MTX NPs closely matched with the pattern of the standard Fe3O4 nanoparticles listed in ASTM XRD standard card (JCPDS 019–0629). The peaks shown in F-Lys-MTX NPs spectrum are at 2θ equals 18.49°, 30.48°, 35.84°, 43.22°, 54.06°, 57.39°, and 62.88° which are accorded well to the characteristic peaks and their relative intensities of spinel structure of magnetite corresponding to the (111), (220), (311), (400), (422), (511), and (440) (hkl) planes, respectively. The XRD pattern revealed the formation of single-phase IONPs.
Magnetic properties
To study the magnetic properties of the F-Lys-MTX NPs, magnetization measurements were performed using a VSM. The saturation magnetization (MS) of the bare IONPs was determined to be 75.29 emu/g ( Bare IONPs). Modification of magnetic nanoparticles surface by L-lysine, and MTX decreased MS to 45.18 emu/g ( F-Lys-MTX NPs). The higher MS of F-Lys-MTX NPs was beneficial for cancer thermochemotherapy and imaging applications.
Drug release study
The mechanism of drug conjugation must be designed to reach significant drug release inside target cells. Previously, Kohler et al. have demonstrated that the covalent bond between an amine-modified nanoparticles and MTX were readily hydrolyzable under intracellular conditions, and allowing the MTX to inhibit the DHFR and stop the FA cycle reducing cellular viability [Citation25,Citation26]. Following their uptake via receptor-mediated endocytosis, lysosome proteases then cleave the peptide bond between the MTX and the nanoparticle, allowing the MTX to be released from the particle surface inside the target cell. Once the MTX is free from the nanoparticle surface, it may enter the cellular cytosol. Once the MTX will be released from the nanoparticle surface, it may inhibit the cell cycle. It was hypothesized that the proteases found in the lysosomal compartment might be able to break down the peptide bond, and releasing free MTX into the cellular cytoplasm. To trial this model, the F-Lys-MTX NPs were incubated with proteinase K solution at acidic pH conditions, similar to conditions found in the lysosome. Results of MTX release as measured by UV–Vis absorbance at 304 nm are shown in . Studies were conducted from 0 to 72 h to obtain an understanding of the kinetics of the MTX release from the nanoparticles. However, the data suggest that MTX release occurred before to the 12 h interval, demonstrating that the protease readily cleaves the peptide bond.
In vitro cytotoxicity and anticancer activity
The in vitro anticancer effects of F-Lys-MTX NPs against human breast adenocarcinoma cell lines (MCF7) were estimated using a MTT assay (. The data exhibit that cell toxicity is directly commensurate to F-Lys-MTX NPs concentration. After 72 h in culture, cells illustrated the downward trend in viability indicative of the cytotoxicity of both the F-Lys-MTX NPs and free MTX over time. The F-Lys-MTX NPs and the free MTX show a similar reduction in cell viability establishing the ability of the cells to cleave MTX in the lysosomes, thus allowing the freed MTX to reduce cellular viability. In addition, a dose response for the F-Lys-MTX NPs was clarified through the viability results.
Figure 9. Cytotoxicity analysis of free MTX and F-Lys-MTX NPs against human breast adenocarcinoma (MCF7) cell lines after incubated for (A) 48 h and (B) 72 h.
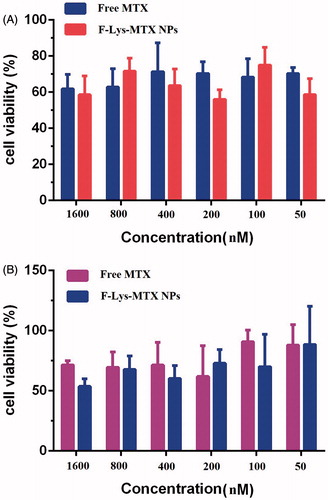
Also, the results of us previously study shown no toxicity for F-Lys NPs in various concentrations [Citation14]. In conclusion, these studies specify that F-Lys-MTX NPs have a very remarkable anticancer effect, for breast cancer cell lines.
Conclusions
IONPs were successfully conjugated with MTX and characterized by FTIR, XRD, DSC, VSM, and TEM techniques. Model studies revealed the release of MTX via peptide bond cleavage in the presence of proteinase K, at low pH. These results suggest that if this system is delivered intravenously, MTX conjugated on nanoparticles will be released largely in lysosomes inside target cells following the particle internalization where pH is low, and many enzymes exist, which may minimize the drug side effects to normal cells. Following 72 h in cell culture medium, MTX-conjugated nanoparticles showed a similar reduction in cellular viability in human breast cancer (MCF-7) cells compared to the void of MTX. This target drug delivery system may allow real-time monitoring of drug delivery by MRI due to the magnetic nature of the synthesized nanoparticles.
Disclosure statement
The authors declare that they have no conflict of interest.
Additional information
Funding
References
- Nosrati H, Rashidi N, Danafar H, et al. Anticancer activity of tamoxifen loaded tyrosine decorated biocompatible Fe3O4 magnetic nanoparticles against breast cancer cell lines. J Inorg Organomet Polym Mater. 2017. DOI:10.1007/s10904-017-0758-7
- Gharebaghi F, Dalali N, Ahmadi E, et al. Preparation of wormlike polymeric nanoparticles coated with silica for delivery of methotrexate and evaluation of anticancer activity against MCF7 cells. J Biomater Appl. 2017;31:1305–1316.
- Gewirtz DA, Bristol ML, Yalowich JC. Toxicity issues in cancer drug development. Curr Opin Investig Drugs. 2010;11(6):612–614.
- Nosrati H, Salehiabar M, Davaran S, et al. New advances strategies for surface functionalization of iron oxide magnetic nano particles (IONPs). Res Chem Intermed. 2017;43:7423–7442.
- Rostami M, Aghajanzadeh M, Zamani M, et al. Sono-chemical synthesis and characterization of Fe3O4@mTiO2-GO nanocarriers for dual-targeted colon drug delivery. In Research on chemical intermediates; 2017. DOI:10.1007/s11164-017-3204-0
- Zamani M, Rostami M, Aghajanzadeh M, et al. Mesoporous titanium dioxide@ zinc oxide–graphene oxide nanocarriers for colon-specific drug delivery. J Mater Sci. 2018;53:1634–1645.
- Manjili H, Malvandi H, Mousavi M-S, et al. Preparation and physicochemical characterization of biodegradable mPEG-PCL coreshell micelles for delivery of artemisinin. Pharm Sci. 2016;22:234–243.
- Nomani A, Nosrati H, Manjili H, et al. Preparation and characterization of copolymeric polymersomes for protein delivery. Drug Res. 2017;90:261–280.
- Danafar H. Applications of copolymeric nanoparticles in drug delivery systems. Drug Res (Stuttg). 2016;66:506–519.
- Kheiri Manjili H, Sharafi A, Attari E, et al. Pharmacokinetics and in vitro and in vivo delivery of sulforaphane by PCL–PEG–PCL copolymeric-based micelles. Artif Cells Nanomed Biotechnol. 2017;45:1728–1739.
- Danafar H. Study of the composition of polycaprolactone/poly (ethylene glycol)/polycaprolactone copolymer and drug-to-polymer ratio on drug loading efficiency of curcumin to nanoparticles. Jundishapur J Nat Pharm Prod. 2017;12:e34179.
- Danafar H, Manjili H, Najafi M. Study of copolymer composition on drug loading efficiency of enalapril in polymersomes and cytotoxicity of drug loaded nanoparticles. Drug Res (Stuttg). 2016;66:495–504.
- Nosrati H, Salehiabar M, Manjili HK, et al. Preparation of magnetic albumin nanoparticles via a simple and one-pot desolvation and co-precipitation method for medical and pharmaceutical applications. Int J Biol Macromol. 2017 [Oct 31]. DOI:10.1016/j.ijbiomac.2017.10.180
- Nosrati H, Salehiabar M, Attari E, et al. Green and one-pot surface coating of iron oxide magnetic nanoparticles (IONPs) with natural amino acids and its biocompatibility investigation. Appl Organomet Chem. 2017 [Sep 29]. DOI:10.1002/aoc.4069
- Peng X-H, Qian X, Mao H, et al. Targeted magnetic iron oxide nanoparticles for tumor imaging and therapy. Int J Nanomedicine. 2008;3:311–321.
- Rapoport N, Christensen D, Fain H, et al. Ultrasound-triggered drug targeting of tumors in vitro and in vivo. Ultrasonics. 2004;42:943–950.
- Di Stefano G, Kratz F, Lanza M, et al. Doxorubicin coupled to lactosaminated human albumin remains confined within mouse liver cells after the intracellular release from the carrier. Dig Liver Dis. 2003;35:428–433.
- ŘíhováJelinkova B, Strohalm M, Št'astný J, et al. Antiproliferative effect of a lectin-and anti-Thy-1.2 antibody-targeted HPMA copolymer-bound doxorubicin on primary and metastatic human colorectal carcinoma and on human colorectal carcinoma transfected with the mouse Thy-1.2 gene. Bioconjugate Chem. 2000;11:664–673.
- Sudimack J, Lee RJ. Targeted drug delivery via the folate receptor. Adv Drug Deliv Rev. 2000;41:147–162.
- Gabizon A, Horowitz AT, Goren D, et al. Targeting folate receptor with folate linked to extremities of poly (ethylene glycol)-grafted liposomes: in vitro studies. Bioconjugate Chem. 1999;10:289–298.
- Stella B, Arpicco S, Peracchia MT, et al. Design of folic acid‐conjugated nanoparticles for drug targeting. J Pharm Sci. 2000;89:1452–1464.
- Aghajanzadeh M, Zamani M, Molavi MH, et al. Preparation of metal–organic frameworks UiO-66 for adsorptive removal of methotrexate from aqueous solution. J Inorg Organomet Polym Mater. 2017;10. DOI:10.1007/s10904-017-0709-3
- Duthie SJ. Folic-acid-mediated inhibition of human colon-cancer cell growth. Nutrition. 2001;17:736–737.
- Narayani R, Rao KP. Controlled release of anticancer drug methotrexate from biodegradable gelatin microspheres. J Microencapsul. 1994;11:69–77.
- Kohler N, Sun C, Wang J, et al. Methotrexate-modified superparamagnetic nanoparticles and their intracellular uptake into human cancer cells. Langmuir. 2005;21:8858–8864.
- Kohler N, Sun C, Fichtenholtz A, et al. Methotrexate‐immobilized poly (ethylene glycol) magnetic nanoparticles for MR imaging and drug delivery. Small. 2006;2:785–792.
- Li M, Neoh K-G, Wang R, et al. Methotrexate-conjugated and hyperbranched polyglycerol-grafted Fe 3 O 4 magnetic nanoparticles for targeted anticancer effects. Eur J Pharm Sci. 2013;48:111–120.
- Chen Y-H, Tsai C-Y, Huang P-Y, et al. Methotrexate conjugated to gold nanoparticles inhibits tumor growth in a syngeneic lung tumor model. Mol Pharm. 2007;4:713–722.
- Salehiabar M, Nosrati H, Davaran S, et al. Facile synthesis and characterization of L-aspartic acid coated iron oxide magnetic nanoparticles (IONPs) for biomedical applications. Drug Res. 2017 [Oct 16]. DOI:10.1055/s-0043-120197
- Rasekh M, Ahmad Z, Cross R, et al. Facile preparation of drug-loaded tristearin encapsulated superparamagnetic iron oxide nanoparticles using coaxial electrospray processing. Mol Pharm. 2017;14:2010–2023.
- Zhang C, Yao Z-, Ding C, et al. The tri-needle coaxial electrospray engineering of magnetic polymer yolk-shell particles possessing dual-imaging modality, multi-agent compartments and trigger release potential. ACS Appl Mater Interfaces. 2017;9:21485–21495.
- Shaabani A, Nosrati H, Seyyedhamzeh M. Cellulose@ Fe2O3 nanoparticle composites: magnetically recyclable nanocatalyst for the synthesis of 3-aminoimidazo [1, 2-a] pyridines. Res Chem Intermed. 2015;41:3719–3727.
- Sun C, Sze R, Zhang M. Folic acid‐PEG conjugated superparamagnetic nanoparticles for targeted cellular uptake and detection by MRI. J Biomed Mater Res Part A. 2006;78:550–557.
- Danafar H, Hamidi M. Pharmacokinetics and bioequivalence study of amlodipine and atorvastatin in healthy male volunteers by LC-MS. Pharm Sci. 2015;21:167–174.
- Yu S, Chow GM. Carboxyl group (–CO 2 H) functionalized ferrimagnetic iron oxide nanoparticles for potential bio-applications. J Mater Chem. 2004;14:2781–2786.
- Gao F, Yan Z, Zhou J, et al. Methotrexate-conjugated magnetic nanoparticles for thermochemotherapy and magnetic resonance imaging of tumor. J Nanopart Res. 2012;14:1160–1171.