ABSTRACT
Modern agriculture has caused extensive soil pesticide contamination, which is a global concern. This study explored the potential of bamboo charcoal (BC) to alleviate quinclorac-induced stress in tobacco plants. A pot experiment was conducted to investigate the effects of BC and vinasse fertilizer (VF) on the growth and physiological parameters of tobacco plants subjected to quinclorac stress. At 30, 60, and 90 d after transplantation, BC and VF exhibited pronounced restorative effects on quinclorac-stressed tobacco plants. The treatments improved plant height, leaf length, and leaf width, and enhanced leaf quality and various biochemical indicators. Treatment with 500 g VF + 4% BC resulted in the lowest residual quinclorac levels in both the soil and leaves. Treatment with 500 g VF + 2% BC resulted in the most significant recovery of agronomic traits, whereas 500 g VF + 0.5% BC was the most effective in enhancing leaf quality. However, the effectiveness of BC decreased with time following application, and furthermore, quinclorac may be released from the BC. In conclusion, BC shows promise in alleviating the adverse effects of quinclorac stress in tobacco plants.
Introduction
Biochar exhibits a substantial specific surface area, porous microstructure, and robust chemical and biological stability, all of which confer the capacity to immobilize contaminants and mitigate their potential for soil contamination (Akhil et al. Citation2021; Jatav et al. Citation2021). These inherent attributes have driven the increasing adoption of biochar for air, water, and soil remediation (Ahmad et al. Citation2014). The mechanisms underlying these processes include physisorption, complexation, precipitation, and ion exchange (Liu et al. Citation2022).
Quinclorac (3,7-dichloroquinoline-8-carboxylic acid) is a quinoline carboxyl auxin herbicide that is used in rice, barley, sorghum, and other crops to the control of monocotyledonous and dicotyledonous weeds. In susceptible species, it induces 1-aminocyclopropane-1-carboxylic acid (ACC) synthase in the ethylene biosynthetic pathway, which activates epidermal growth and triggers abscisic acid (ABA) synthesis, leading to stomatal closure (Grossmann Citation2000). A previous study found that the leaves of tobacco, a follow-up crop sown one year after treatment with quinclorac, showed different dose-dependent visible phytotoxicity symptoms (Zhong et al. Citation2018). Quinclorac residues in the soil may become potential environmental hazards and disturb the natural ecological balance (Alonso et al. Citation2020).
Biochar has received increasing attention as a soil amendment treatment to remediate the phytotoxicity caused by herbicides in plants. Previous research has demonstrated that biochar can reduce the leaching of diuron and 2-(4-chloro-2-methylphenoxy)acetic acid (MCPA), probably because of its direct effect on the soil surface or the adsorption layer near the soil surface (Cederlund et al. Citation2017). Biochar can control pesticide transfer and enhance herbicide biodegradation. Research has confirmed that biochar has the potential to reduce the leaching and environmental pollution caused by polar imidazolinones (Saba et al. Citation2022) Earlier reports indicated the effectiveness of using activated carbon in transplanting water to reduce the phytotoxic effects on tobacco following pre-transplanting herbicide applications (Yelverton et al. Citation1992).
However, there have been reports suggesting that biochar incorporation into soil can stabilize pesticides through sorption, ultimately resulting in decreased pesticide bioavailability and ecotoxicity (Egamberdieva et al. Citation2021). The degree of pesticide adsorption by biochar affects its desorption, thereby regulating its bioavailability in the soil. Smaller biochar particles may have the high surface area and porosity, which helps provide more adsorption sites. This may retain pesticide molecules, thereby hindering pesticide activation and reducing the uptake of pesticides by plants (Yan et al. Citation2022; Cara et al. Citation2022). Some studies have also found that the addition of biochar to arsenic-contaminated paddy soils enhances the reduction of Fe in the soil, thereby increasing the release of As (Wang et al. Citation2017). After adsorbing phosphorus from the soil, soluble phosphate-modified biochar (BCP) has a higher phosphorus release risk (Cui et al. Citation2022), indicating that biochar may undergo a process of re-releasing pollutants after adsorption.
Many studies have focused on biochar as a soil amendment. However, reports on the release of soil contaminants after its absorption are still limited. In the present study, we explored the effects of bamboo charcoal (BC) on the dynamic changes in agronomic traits and biochemical indicators of tobacco plants, as well as the residual content of quinclorac in soil and tobacco leaves under various periods of quinclorac stress. This is of great significance for improving remediation technology using biochar in soil contaminated by herbicides in tobacco farming areas, and for avoiding the secondary pollution caused by biochar remediation in agriculturally contaminated soil.
Materials and methods
This study was conducted as a pot experiment in an experimental field station at Guizhou University, China. The soil was obtained from an uncultivated natural source in the Huaxi District of Guiyang City. The soil was yellowish-black, and was paved and exposed to the sun before use.
Materials
A 50%-wettable quinclorac powder produced by Jiangsu Kuaida Agrochemical Limited (Share Ltd.) was used in the experiment. BC was provided by the Lin Quan Tobacco Science Park, Guizhou Bijie Tobacco Co., Ltd. (Guizhou, China). The Science Park incinerated bamboo (Phyllostachys heterocycla var. pubescens (Pradelle) Ohwi) at 550 °C, followed by washing it with deionized water to remove surface residues, and drying it at 105 °C for 24 h. Subsequently, the resulting bamboo charcoal was broken down into specific size classes for future use. Vinasse fertilizer (VF), produced by Guizhou Jilong Ecological Science and Technology Co., Ltd., is a safe and refined fertilizer generated by the multi-stage harmless treatment of livestock manure using earthworms or biological agents, containing over 4% total nutrients and over 30% VF. The tobacco variety used was ‘Yunyan 87’, which was provided by Lin Quan Tobacco Science Park, Guizhou Bijie Tobacco Co., Ltd. (Guizhou, China). The basic properties of the BC and tested soils are listed in . Quinclorac standard product was purchased from Shanghai Aladdin Biochemical Technology Co., Ltd. (Shanghai, China). Methanol and acetic acid were obtained from Tianjin Fuyu Fine Chemical Co., Ltd. (Tianjin, China).
Table 1. The basic characteristics of the bamboo charcoal produced by Lin Quan tobacco Science Park from incinerated bamboo (Phyllostachys heterocycla var. pubescens.).
Table 2. The basic properties of the soil used in the potting experiment.
Treatments
The soil was accurately weighed at 10 kg and placed into a garden pot (depth × width = 15 cm × 25 cm) with the addition of 1 g of tobacco-specific fertilizer. Quinclorac was added at twice the conventional recommended dose; that is, the soil concentration of quinclorac was 0.2 mg kg−1. The highest application concentration of quinclorac in this experiment was determined based on its maximum field application, as well as the reference value of 225 kg dry soil m−2 at a 20 cm tillage layer in farmland for conversion. In the present study, the recommended dosage of quinclorac was 45 g m−2 and the volume of water was 45 mL m−2, resulting in a recommended concentration of 1 g L−1. Based on 0.2 g t−1, the recommended dosage of chemical was 0.2 mg quinclorac in 1 kg soil, i.e. the quinclorac concentration in soil was 0.2 mg kg−1 ×50% = 0.1 mg kg−1. Using the spraying method, 4 mL of 1 g L−1 quinclorac solution was added to increase the soil chemical content to 0.2 mg kg−1; this concentration was twice the recommended dosage of quinclorac. The liquid mixture was sprinkled several times on the soil in the garden plot and mixed evenly to prevent chemical loss. 1 d later, the seven treatments were fully mixed with the treated soil, each with 10 replicates. The treatments were as follows: CK: blank control, without application of quinclorac; CK1: 500 g VF, mixed with quinclorac-treated soil; CK2: no amendment control, 50% quinclorac in garden pots without other amendments; T1: 500 g VF with 0.5% BC for each plant, i.e. 500 g VF + BC of 495 kg ha−1; T2: 500 g VF with 1% BC for each plant, i.e. 500 g VF + BC of 990 kg ha−1; T3: 500 g VF with 2% BC for each plant, i.e. 500 g VF + BC of 1980 kg ha−1; T4: 500 g VF with 4% BC for each plant, i.e. 500 g VF + BC of 3960 kg ha−1. 2 d after adding the amendments, one seedling at the three-leaf stage with consistent and healthy growth was transplanted per pot, watered, and subjected to the regular management practices of premium tobacco.
Agronomic traits and biochemical parameters
The remediation rates of plant height, leaf length, leaf width, stem diameter, and leaf number of tobacco plants were determined 30, 60, and 90 d after transplanting. The soil and leaf samples of all treatments were collected before and 30, 60, and 90 d after remediation for the quinclorac residue test. Protein, starch, total sugar, reducing sugar, nicotine, and peroxidase (POD) activities were measured 90 d after remediation. The tobacco leaves from the top to the fourth most effective leaves were sampled for analysis. Antioxidant enzyme solution was extracted based on Moerschbacher et al. (Citation1988). POD was quantified using the methods described by Zhang and Kirkham (Citation1994). Starch, total sugar, and reducing sugar contents were estimated using the 3,5-dinitrosalicylic acid (DNS) method (Kim et al. Citation2014). The nicotine content was determined using an ultraviolet spectrophotometer (Wei et al. Citation2018). The remediation rate was calculated using the following equation:
Remediation rate = (treatment index – no amendment control index)/(blank control index – no amendment control index) × 100.
Determination of quinclorac residues in tobacco growing soil and tobacco leaves
Liquid chromatography (Waters 600E, Waters, U.S.A.) with a UV detector was used to measure quinclorac concentrations. The detection parameters were as follows: an Agilent 300SB-C18 (4.6 × 250 mm, 5 μm) chromatographic column was used with the mobile phase of methanol:1% acetic acid (60:40), a flow rate of 1 mL min−1, a detection wavelength of 240 nm, a column temperature of 30 °C, and a sample loading of 10 µL. The chemical content was determined by the retention time, and the concentration was quantified by the peak areas. The retention time of quinclorac was 12.708 min under these conditions. The quinclorac standard product (0.0100 g) was accurately weighed to prepare a standard solution of 100 mg kg−1 using methanol, which was serially diluted with methanol to six concentrations: 0.05, 0.10, 0.50, 1.00, 5.00, and 10.00 mg L−1, and their peak areas were determined using the detection method. The X-axis and Y-axis represent the sample loading colume and peak areas, respectively, to create the standard curve of the residue, and the regression equation Y = 5157× + 63.33 with an R2 value of 0.996 was obtained, where Y is the peak area and X is the residual quinclorac (mg kg−1). Three levels of quinclorac (0.01, 0.05, and 0.10 mg kg−1) were added to blank soil and tobacco leaves (20 g each), each with three replicates, and the recovery rate in soil and tobacco leaves was determined using the above-described conditions and instruments. The average recovery rate of quinclorac in soil was 96.8%~109%, with a standard deviation of 1.3%~2.2%, and it ranged from 85.6%–96.0% in tobacco leaves, with a standard deviation of 5.8%~7.1%. These data suggest that the accuracy and precision of the extraction and detection methods used in this study meet the basic requirements for pesticide residue analysis.
Statistical analysis
All the data represent the average values of at least three replicates with standard deviations. One-way analysis of variance (ANOVA) followed by Tukey’s F-test was performed. Data were analyzed using SPSS (version 18.0; SPSS Inc., Chicago, IL, U.S.A.).
Results and discussion
The addition of BC and VF improved soil quality and had a positive impact on the agronomic traits and biological activity of tobacco plants affected by quinclorac soil residues.
Agronomic traits of tobacco plants
The recovery rate of plant height ranged from 63.0% to 526% for the different treatments (30 d, 60 d, and 90 d after transplanting), and this effect decreased with an increase in growth duration (). The best effect was observed in the 500 g VF + 2% BC treatment, with recovery rates of 526%, 284%, and 149% at 30 d, 60 d, and 90 d after seedling transplantation.
Figure 1. Agronomic traits of tobacco plants.
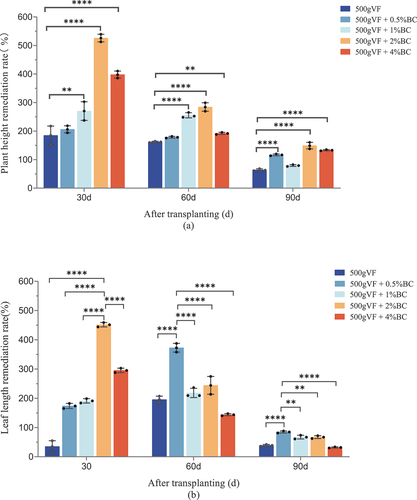
Treatment with VF mixed with contrasting masses of BC showed the desired remediation effect on the leaf length of quinclorac-stressed tobacco (). 30 d after seedling transfer, the recovery rate of leaf length exhibited an increase followed by a decrease as the mass of BC increased. Therefore, the best application effect was observed when using 500 g VF + 2% BC, the recovery rate of which was 451%, which was significantly different from the other treatments (p < 0.01). At 60 d, 500 g VF + 0.5% BC showed the best recovery rate of 372%, which was significantly different from that of the other treatments (p < 0.01). At 90 d, 500 g VF + 0.5% BC also presented the best recovery rate of 85%, but this was not significant when compared to the treatment with 500 g VF alone (p > 0.05), indicating that VF plays an important role in the remediation of leaf length in quinclorac-stressed tobacco 90 d after transfer.
The remediation effects of VF and BC on the width of quinclorac-exposed tobacco leaves are shown in . The quinclorac soil residue substantially reduced the leaf width of damaged tobacco, and the recovery rate ranged from 1.6% to 131%. During remediation, the recovery rate of the leaf width exhibited a declining trend in various treatments over time. The best effect was observed 30 d after remediation upon treatment with 500 VF + 2% BC at a rate of 131%. At 60 d and 90d, the treatment with 500 g VF + 0.5% BC showed the best remediation effect, but it was not significantly different from the other treatments (p > 0.05), suggesting that BC is not the major remediation factor for leaf width in stressed tobacco plants.
The remediation effects of VF and BC on the stem diameters of quinclorac-stressed tobacco leaves under various treatments are shown in . 30 and 60 d after seedling transplantation, the recovery rate of stem diameters improved with an increase in the concentration of BC, and they exhibited a positive correlation. In both periods, the best recovery rates were observed in the combined treatment of 500 g VF + 4% BC at 114% and 295%. With the extension of the remediation time (30 d, 60 d, and 90 d), the recovery rate of stem diameter expressed a trend of initial increase and subsequent decrease. At 90 d, the recovery rate under the 500 g VF treatments with three different masses (1%, 2%, and 4%) of BC was 50.1%, which was statistically similar to that of the other treatments (p > 0.05). These findings imply that while BC initially helps to remediate the phytotoxicity caused by quinclorac, its effectiveness decreases over time. This could mean that the remediation effects of BC may have a limited duration or may decline over time owing to various factors, such as environmental conditions or soil interactions.
The use of BC significantly increased the height and leaf length of tobacco plants (p < 0.05; ). This positive effect has also been observed in various plants, such as Cassia alata L (Huang et al. Citation2018), maize (Rafique et al. Citation2017), and tomatoes (Kul et al. Citation2021). This effect may be attributed to a reduction in pesticide toxicity and the provision of nutrients by BC and VF. The external surface of biochar contains abundant oxygen-containing functional groups, such as carboxyl (−COOH), hydroxyl (−OH), and ester (−COOR) groups, which can result in specific interactions between the biochar and organic pollutants (Liu et al. Citation2018) Additionally, the presence of biochar significantly increases the cation exchange capacity (CEC) of soil (Yavari et al. Citation2023), thereby enhancing the adsorption behavior of biochar towards pollutants. The application of VF and its combination with BC exhibited remediation effects on the agronomic traits of quinclorac-stressed tobacco plants during all test periods. This is similar to a report by Cheng et al. (Citation2022) who discussed the effective immobilization and degradation of neonicotinoid pesticides using biochar and composted chicken manure (CCM) to mitigate their adverse effects and improve soil fertility in agricultural fields. The results of this study revealed that mixing BC with VF is an effective method for remediating the phytotoxicity of quinclorac in tobacco plants. This indicated that BC and VF can help reduce the harmful effects of quinclorac on tobacco crops.
Table 3. Remediation effect of different concentrations of bamboo charcoal treatment on the agronomic traits of flue-cured tobacco exposed to high residual quinclorac.
POD activity and protein content
As shown in , the POD activity in CK2 was the highest, indicating that quinclorac can increase the enzyme activity of POD in tobacco leaves to enhance stress resistance. With various amendment treatments, the POD activity in tobacco planted in quinclorac-affected soil was significantly lower than that in CK2, and the best remediation effect on POD was observed when treated with 500 g VF + 0.5% BC, followed by 500 g VF alone. The impact of the amendments was reduced with an increase in the concentration of BC. Therefore, a low concentration of BC is favorable for reducing the POD activity in quinclorac-stressed tobacco leaves, thus alleviating the toxicity of quinclorac. The addition of biochar can enhance plant tolerance and alleviate oxidative stress by regulating POD activity and gene expression (Mehmood et al. Citation2018).
Figure 2. POD activity and protein content. POD activities (a) and protein contents (b) in tobacco remediated with mixed bamboo charcoal and vinasse fertilizer to quinclorac phytotoxicity.
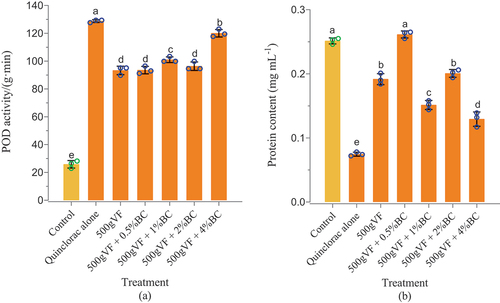
As shown in , the protein content of the negative control (CK) and other amendment treatments ranged from 0.0747 to 0.2613 mg L−1. The protein content in the treatments of VF alone, CK2, and VF mixed with BC at various concentrations (1%, 2%, and 4%) was lower than that of CK, and CK2 showed a significantly lower average protein content than CK, suggesting that residual quinclorac in soil reduced the protein content in the affected tobacco. In addition, the protein content with amendments was substantially higher than that with CK2. The highest protein level (0.2613 mg L−1) was observed after treatment with 500 g VF + 0.5% BC, showing the best remediation effect with a recovery rate of 106%. The second highest recovery rate was 71.4%, which was observed with the combined treatment of 500 g VF + 2% BC (T3) and was significantly different from other treatments. Many researchers have discovered that biochar can increase plant protein content, promote plant growth, and enhance plant resistance to abiotic stress (Younis et al. Citation2016; Kiran and Prasad Citation2019). These data indicate that applying the combined amendments of VF and BC can compensate for the negative effects of quinclorac on the protein synthesis pathway in tobacco.
Tobacco leaf quality
The impact of the combined treatment with BC and VF on the leaf quality of quinclorac-phytotoxic tobacco is shown in 90 d after seedling transfer, the starch, total sugar, and reducing sugar contents in CK2 were lower than those in CK, but the nicotine content was higher. In all remediation treatments, the starch content in tobacco leaves exhibited an increase, followed by a decrease with an increase in charcoal concentration. A peak content of 27.9% was observed after treatment with 500 g of VF. Treatment with 500 g VF + 0.5% BC resulted in the highest total sugar and sugar content (16.7% and 14.1%, respectively); however, their overall pattern showed a downward trend. No significant variation was observed in the nicotine content (p > 0.05), among which CK2 had the highest level (2.3%).
Figure 3. Tobacco leaf quality.
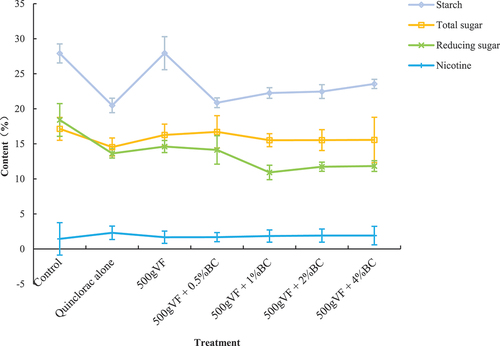
The sole application of 500 g VF showed the best remediation effect on leaf starch, reducing sugar, and nicotine in quinclorac-stressed tobacco 90 d after treatment with amendments. In addition, 500 g VF + 0.5% BC restored the total sugar content to an optimal level, indicating that combining VF and a low mass of BC had a stronger effect on restoring the leaf quality of tobacco under quinclorac contamination.
The extent of the effect of biochar on the efficacy of herbicides depends on the chemical properties of the herbicide molecules and their modes of action, as well as the aging of biochar in soils (Gámiz et al. Citation2019). Yang et al. (Citation2019) reported that five activated carbon carriers showed good adsorption and sustained release effects on 2, 4-D sodium, and pore size distribution and chemical interactions were the main factors affecting herbicide loading and the release of activated carbon carriers. In this experiment, 90 d after transplanting, the quality, POD, and protein content of quinclorac-damaged tobacco leaves treated with a low mass of biochar were better than those treated with a high mass of biochar. This is probably because the high concentration of BC absorbed more quinclorac from the soil before the plant resettling stage because of its higher porosity and total specific surface area compared to the low charcoal concentration. Thus, at 60 d, the long half-life of the previously adsorbed quinclorac and its reduced self-degradation resulted in higher chemical release and the release characteristics of BC itself, which increased the quinclorac concentration in the soil and reduced the phytotoxicity symptoms in tobacco. This lead to a reduction in plant quality and the content of POD enzyme activity and protein. Thus, although biochar may be a potentially attractive tool for the remediation of contaminated soils, further evaluation of the effects of different biochars on the degradation of various pesticides under both short- and long-term conditions is required to determine their ecological efficacy and explore their field potential before wide-scale application. Other factors to consider are the number of pollutants that biochar can retain before saturation and the persistence of the biochar-pollutant complex.
Quinclorac residue
The mixed application of BC and VF reduced the amount of residual quinclorac in the soil (). During the time course of remediation, the soil residual concentration of quinclorac exhibited a reduction-elevation-decline trend in treatments T1, T2, T3, and T4. At 30 d, the concentration of quinclorac residue in all amendment treatments (CK1, T1, T2, T3, and T4) was lower than that in CK2, and the lowest concentration (0.0293 mg kg−1) was observed in T4, which was 84.8% lower than that in the control. The residual concentration of the herbicide was measured in all treatments at 60 d, and was 0.0416, 0.0786, 0.0712, 0.0860, and 0.0912 mg kg−1 in the CK1, T1, T2, T3, and T4 treatments. Of these, the treatment with 500 g VF + 4% BC exhibited the highest residual concentration. This is very similar to the findings of Saba et al. (Citation2022), who reported that, in a short period of time, the immobilization capacity of biochar for herbicides increased with the application rate. This suggests that increasing the dosage or application rate of BC has a positive effect on mitigating the harmful effects of quinclorac on tobacco plants. The soil concentration of quinclorac ranged from 0 to 0.0633 mg kg−1 among the various treatments at 90 d. No residue was detected in T1 and T2, but T4 showed the highest level of 0.0633 mg kg−1. These results suggest that mixing BC in VF has an absorption effect on quinclorac to reduce its level in soil, but that BC releases quinclorac as time passes, which elevates quinclorac concentrations in the soil.
Figure 4. Quinclorac residue.
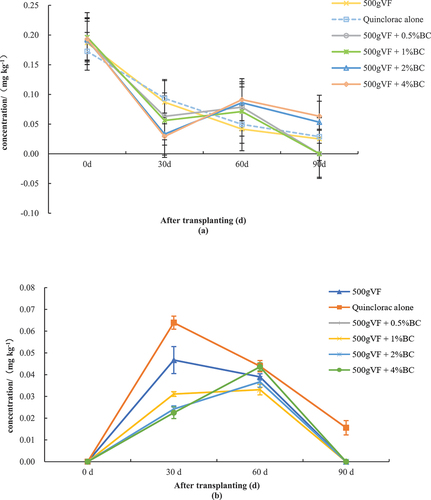
The effects of mixing BC at various concentrations with VF on the residual concentration of quinclorac in tobacco leaves during different periods are shown in . During all periods of remediation, the leaf quinclorac concentration in the treatments with amendments was lower than that of CK2, suggesting that VF or its combination with BC reduces the concentration of quinclorac residues in tobacco leaves. In the three intervals (periods of 0–30 d, 30–60 d, and 60–90 d), the leaf residual quinclorac exhibited elevation-reduction-reduction in CK1, CK2, and T1. The residual concentration at 30 d was the greatest at 0.0639, 0.0467, and 0.0400 mg kg−1 for CK2, CK1, and T1. However, the trend was the same in T2, T3, and T4, showing an elevation-elevation-reduction trend. Of these treatments, the largest concentrations of residual quinclorac were observed at 60 d, which were 0.0436, 0.0367, and 0.0331 mg kg−1 for T4, T3, and T2.
This study found that with an increase in remediation time, the restoration of various agronomic traits decreased. In addition, the quinclorac residue in the soil and leaves of the no-amendment control decreased with increasing time, whereas the residue under the mixed treatment of 500 g VF with 2% or 4% BC was elevated during the period of 30–60 d. BC adsorbs quinclorac in the soil, reducing its bioavailability. This indicates that tobacco plants absorb less quinclorac, potentially reducing their exposure to pesticides. However, BC was not able to fully adsorb quinclorac, and over time, quinclorac may have been released from bamboo charcoal into the tobacco-planting soil. This secondary release can also be advantageous, as it may prolong the effectiveness of the herbicide, leading to longer-lasting weed control in tobacco fields. Luo et al. (Citation2023) also reported that using biochar to recover phosphorus from wastewater and using it as a slow-release fertilizer holds promising application prospects, addressing both environmental pollution and phosphorus resource shortage issues. Further validation of the mechanism of the mixed treatment using VF and BC on the absorption and release of residual quinclorac in soil is needed, which can provide a scientific theoretical basis for the study of bioremediation of soil phytotoxicity caused by residual quinclorac and risk avoidance of secondary soil pollution during bioremediation.
Conclusion
In conclusion, the effectiveness of combining BC and VF in reducing the residual quinclorac content in tobacco-planted soil was demonstrated. This approach not only reduced quinclorac residues but also exerted a positive effect on the growth and physiological parameters of tobacco plants, mitigating the adverse effects of quinclorac-induced stress. Application of BC and VF enhanced tobacco plant resilience to quinclorac stress. Notably, the efficacy of BC in quinclorac remediation diminished over time, which is potentially attributable to BC aging. The sustained slow-release effect of BC on herbicides, such as quinclorac, offers the potential for extended herbicide effectiveness, providing long-term weed control in tobacco fields. However, this benefit comes with the challenge of potential environmental pollution and the need for careful management.
This study represents a pioneering evaluation of the long-term effects of BC on quinclorac dynamics. Additionally, we report the process of quinclorac adsorption and release by BC. However, certain aspects such as the potential interaction between VF and BC and the economic considerations associated with remediating quinclorac-contaminated soil were not addressed in this study. Therefore, further research is warranted to investigate the interplay between VF and BC on soil microflora and enzyme activity, as well as to assess its economic feasibility. Additionally, exploring the long-term environmental impacts of BC in diverse agricultural systems will contribute to advancing sustainable agricultural practices and pesticide mitigation measures.
Highlights
Mixing bamboo charcoal with vinasse fertilizer effectively remediate the phytotoxicity of quinclorac to tobacco.
Increases in the bamboo charcoal’ application rates enhanced their effects.
The effectiveness of bamboo charcoal in quinclorac phytotoxicity remediation diminishes over time.
Quinclorac adsorption was insufficient by bamboo charcoal and was prone to secondary release in tobacco-planting soil.
Acknowledgments
The 13th Batch of Guizhou Outstanding Young Scientific and Technological Talents Project (grant no. QKHPTRC5614-2021).
Disclosure statement
No potential conflict of interest was reported by the authors.
Data availability statement
The data used to support the findings of this study are available from the corresponding author upon reasonable request.
Additional information
Funding
References
- Ahmad M, Rajapaksha AU, Lim JE, Zhang M, Bolan N, Mohan D, Vithanage M, Lee SS, Ok YS. 2014. Biochar as a sorbent for contaminant management in soil and water: a review. Chemosphere. 99:19–33. doi: 10.1016/j.chemosphere.2013.10.071.
- Akhil D, Lakshmi D, Kartik A, Vo D-V, Arun J, Gopinath KP. 2021. Production, characterization, activation and environmental applications of engineered biochar: a review. Environ Chem Lett. 19(3):2261–2297. doi: 10.1007/s10311-020-01167-7.
- Alonso FG, Mendes KF, Junqueira LV, Takeshita V, Almeida CDS, Tornisielo VL. 2020. Distribution and formation of degradation products of 14C-quinclorac in five tropical soils. Arch Agron Soil Sci. 66(11):1598–1609. doi: 10.1080/03650340.2019.1681589.
- Cara IG, Țopa D, Puiu I, Jităreanu G. 2022. Biochar a promising strategy for pesticide-contaminated soils. Agriculture. 12(10):1579. doi: 10.3390/agriculture12101579.
- Cederlund H, Börjesson E, Stenström J. 2017. Effects of a wood-based biochar on the leaching of pesticides chlorpyrifos, diuron, glyphosate and MCPA. J Environ Manage. 191:28–34. doi: 10.1016/j.jenvman.2017.01.004.
- Cheng H, Tang G, Wang S, Rinklebe J, Zhu T, Cheng L, Feng S. 2022. Combined remediation effects of biochar and organic fertilizer on immobilization and dissipation of neonicotinoids in soils. Environ Int. 169:107500. doi: 10.1016/j.envint.2022.107500.
- Cui H, Dong T, Hu L, Xia R, Jing Zhou Z, Zhou J. Adsorption and immobilization of soil lead by two phosphate-based biochars and phosphorus release risk assessment. Sci Total Environ. 2022 Jun. 824:153957. doi:10.1016/j.scitotenv.2022.153957.
- Egamberdieva D, Jabbarov Z, Arora NK, Wirth S, Bellingrath-Kimura SD. 2021. Biochar mitigates effects of pesticides on soil biological activities. Environ Sustain. 4(2):335–342. doi: 10.1007/s42398-021-00190-w.
- Gámiz B, Velarde P, Spokas KA, Cox L. 2019. Dynamic effect of fresh and aged biochar on the behavior of the herbicide mesotrione in soils. J Agric Food Chem. 67(34):9450–9459. doi: 10.1021/acs.jafc.9b02618.
- Grossmann K. 2000. Mode of action of auxin herbicides: a new ending to a long, drawn out story. Trends Plant Sci. 5(12):506–508. doi: 10.1016/S1360-1385(00)01791-X.
- Huang L, Li Y, Zhao M, Chao Y, Qiu R, Yang Y, Wang S. 2018. Potential of cassia alata L. Coupled with biochar for Heavy Metal Stabilization in multi-Metal mine tailings. Int J Environ Res Public Health. 15(3):494. doi: 10.3390/ijerph15030494.
- Jatav HS, Rajput VD, Minkina T, Singh SK, Chejara S, Gorovtsov A, Barakhov A, Bauer T, Sushkova S, Mandzhieva S, et al. 2021. Sustainable approach and safe use of biochar and its possible consequences. Sustainability. 13(18):10362. doi: 10.3390/su131810362.
- Kim J, Kwon Y-K, Kim JH, Heo S-J, Lee Y, Lee S-J, Shim W-B, Jung W-K, Hyun J-H, Kwon KK, et al. 2014. Effective microwell plate-based screening method for microbes producing cellulase and xylanase and its application. J Microbiol Biotechnol. 24(11):1559–1565. doi:10.4014/jmb.1405.05052.
- Kiran BR, Prasad MNV. 2019. Biochar and rice husk ash assisted phytoremediation potentials of ricinus communis L. for lead-spiked soils. Ecotoxicol Environ Saf. 183:109574. doi: 10.1016/j.ecoenv.2019.109574.
- Kul R, Arjumend T, Ekinci M, Yildirim E, Turan M, Argin S. 2021. Biochar as an organic soil conditioner for mitigating salinity stress in tomato. Soil Sci Plant Nutr. 67(6):693–706. doi: 10.1080/00380768.2021.1998924.
- Liu T, Lawluvy Y, Shi Y, Ighalo JO, He Y, Zhang Y, Yap P-S. 2022. Adsorption of cadmium and lead from aqueous solution using modified biochar: a review. J Environ Chem Eng. 10(1):106502. doi: 10.1016/j.jece.2021.106502.
- Liu Y, Lonappan L, Brar SK, Yang S. 2018. Impact of biochar amendment in agricultural soils on the sorption, desorption, and degradation of pesticides: a review. Sci Total Environ. 645:60–70. doi: 10.1016/j.scitotenv.2018.07.099.
- Luo D, Wang L, Nan H, Cao Y, Wang H, Kumar TV, Wang C. 2023. Phosphorus adsorption by functionalized biochar: a review. Environ Chem Lett. 21(1):497–524. doi: 10.1007/s10311-022-01519-5.
- Mehmood S, Saeed DA, Rizwan M, Khan MN, Aziz O, Bashir S, Ibrahim M, Ditta A, Akmal M, Mumtaz MA, et al. 2018. Impact of different amendments on biochemical responses of sesame (sesamum indicum L.) plants grown in lead-cadmium contaminated soil. Plant Physiol Biochem. 132:345–355. doi: 10.1016/j.plaphy.2018.09.019.
- Moerschbacher BM, Noll UM, Flott BE, Reisener H-J. 1988. Lignin biosynthetic enzymes in stem rust infected, resistant and susceptible near-isogenic wheat lines. Physiol Mol Plant Pathol. 33(1):33–46. doi: 10.1016/0885-5765(88)90041-0.
- Rafique M, Sultan T, Ortas I, Chaudhary HJ. 2017. Enhancement of maize plant growth with inoculation of phosphate-solubilizing bacteria and biochar amendment in soil. Soil Sci Plant Nutr. 63(5):460–469. doi: 10.1080/00380768.2017.1373599.
- Saba Y, Asadpour R, Kamyab H, Sara Y, Kutty SRM, Baloo L, TSBA M, Chelliapan S, Sidik ABC. 2022. Efficiency of carbon sorbents in mitigating polar herbicides leaching from tropical soil. Clean Technol Environ Policy. 24(1):251–260. doi: 10.1007/s10098-021-02113-z.
- Saba Y, Kamyab H, Abd Manan TS B, Chelliapan S, Asadpour R, Sara Y, Sapari NB, Baloo L, Sidik ABC, Kirpichnikova I. 2022. Bio-efficacy of imidazolinones in weed control in a tropical paddy soil amended with optimized agrowaste-derived biochars. Chemosphere. 303:134957. doi: 10.1016/j.chemosphere.2022.134957.
- Wang N, Xue X-M, Juhasz AL, Chang Z-Z, Li H-B. 2017. Biochar increases arsenic release from an anaerobic paddy soil due to enhanced microbial reduction of iron and arsenic. Environ Pollut. 220:514–522. doi: 10.1016/j.envpol.2016.09.095.
- Wei XN, Liu Y , Tang YL. 2018. Nicotine content of tobacco leaf estimated by UV spectrum. IOP Conf Ser Earth Environ Sci. 185(1):012017. doi: 10.1088/1755-1315/185/1/012017.
- Yang J, Zang W, Zhang Z, Wang P, Yang Q. 2019. The enhanced and tunable sustained release of pesticides using activated carbon as a carrier. Materials. 12(23):4019. doi: 10.3390/ma12234019.
- Yan P, Zou Z, Li X, Liping Z, Lan Z, Fu J, Wenyan H. 2022. Biochar changed the distribution of imidacloprid in a plant–soil–groundwater system. Chemosphere. 307:136213. doi: 10.1016/j.chemosphere.2022.136213.
- Yavari S, Kamyab H, Asadpour R, Yavari S, Sapari NB, Baloo L, Manan TSBA, Ashokkumar V, Chelliapan S. 2023. The fate of imazapyr herbicide in the soil amended with carbon sorbents. Biomass Conv Bioref. 13(9):7561–7569. doi: 10.1007/s13399-021-01587-7.
- Yelverton FH, Worsham AD, Peedin GF. 1992. Activated Carbon Reduces Tobacco (Nicotiana tabacum) Injury from Soil-Applied Herbicides. Weed Technol. 6(2):310–316. doi: 10.1017/S0890037X00034783.
- Younis U, Malik SA, Rizwan M, Qayyum MF, Ok YS, Shah MHR, Rehman RA, Ahmad N. 2016. Biochar enhances the cadmium tolerance in spinach (Spinacia oleracea) through modification of cd uptake and physiological and biochemical attributes. Environ Sci Pollut Res. 23(21):21385–21394. doi: 10.1007/s11356-016-7344-3.
- Zhang J, Kirkham MB. 1994. Drought-stress-induced changes in activities of superoxide dismutase, Catalase, and peroxidase in wheat species. Plant Cell Physiol. 35(5):785–791. doi: 10.1093/oxfordjournals.pcp.a078658.
- Zhong Q, Wan S, Shen C, Liu Y. 2018. Decay of quinclorac in acidic paddy soil and risk evaluation to the subsequent Crop, tobacco (nicotiana tabacum L.). Bull Environ Contam Toxicol. 101(2):284–287. doi: 10.1007/s00128-018-2372-y.