Abstract
The South African solar resource is vast. By utilising this resource effectively, concentrating solar power (CSP) offers the ability to efficiently store thermal energy until needed for electricity generation. This technology can therefore assist the total electricity system to link demand and supply. Nevertheless, CSP is still entering the commercialisation phase and, as the learning rate sets in, the cost is expected to decrease significantly; thus providing a dispatchable renewable energy option that is competitive with conventional options. A major dilemma needs to be overcome. Until sufficient CSP capacity is installed each year, the localisation potential, and the overall economic benefit for the country, will not materialise. This in turn could stall the technology. This paper presents a techno-economic scenario to show that a CSP industry can be established now that exceeds the threshold for setting up economies of scale, reduces the cost of electricity, and increases energy security.
1. Introduction
Solar irradiation is arguably one of Africa's greatest endowments, with most of the continent being suitable for photovoltaic (PV) electricity generation and being the only continent straddling both sunbelts, the sunniest regions of the world where concentrating solar power (CSP) is most viable. The continent lags significantly in the deployment of both technologies, but as costs continue to drop it is expected that this will change (IEA, Citation2010a; Citation2010b). presents satellite-derived long-term annual average irradiation for direct normal irradiation and global horizontal irradiation (GeoModel Solar, Citation2013) applicable to CSP and PV power respectively.
Figure 1: Long-term average satellite-derived annual (left) direct normal irradiation and (right) global horizontal irradiation
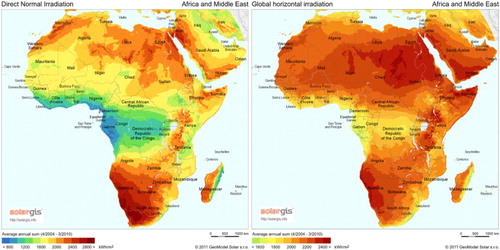
Many Southern African Development Community (SADC) countries have large areas of direct normal irradiation exceeding economically feasible thresholds of 2000 kWh/m2, and the south-western region is considered to have one of the best direct normal irradiation resources worldwide (IEA, Citation2010a). At the same time, much of the SADC is non-electrified (SADC, Citation2010). Many of the member countries have low rates of access to electricity (see ) and rely on expensive to operate emergency generators (Merven et al., Citation2010; IRENA, Citation2013a, Citation2013b).
Table 1: Summary of macroeconomic and electricity statistics in the SADC
With this in mind, solar energy – including CSP – presents an opportunity to replace the high-cost emergency generation and to further electrify the region. It is assumed that, to be worthwhile, the provision of electricity needs to be available on demand, which implies that electricity dispatch will be important.
South Africa has adopted a process to migrate towards a lower carbon energy system. The process includes an electricity plan, the Integrated Resource Plan (IRP) (DoE, Citation2011), which will continuously look ahead 20 years at a time, adjusting the mix of electricity generation based on priorities and improved information. The first revision of the IRP is generous towards renewable energy capacity but overwhelmingly favours more mature wind and PV technology, both intermittent renewable technologies that have little or no firm capacity requiring almost complete backup. The draft update of the IRP (DoE, Citation2013a) shows the plan beginning to evolve, particularly recognising the role of CSP providing dispatch electricity as mitigation for intermittency but also in recognition of cost risk for other technologies.
The current barrier for CSP is the high capital cost of these plants, which relates to the maturity of the technology. CSP is expected to benefit from cost reduction, but the longer the technology is not embraced regionally, the higher the probability of not benefiting from its use and industrial potential.
This paper outlines the expected value of CSP in the context of the regional electricity system, but with a specific focus on South Africa. An approach is proposed for the rapid deployment of CSP plants that can reduce the cost of electricity even in the short term in order to realise the expected economic benefits; by focusing on energy use, capacities, needs, gaps and resources in the country and the region. The objective of the paper is then to suggest the means for the South African CSP industry to reach critical mass by applying CSP to compete against the most expensive electricity generators in South Africa.
2. Electricity supply and energy resources in South Africa and the region
2.1 Existing electricity supply and demand
South Africa stands out in the SADC region for electricity use where electricity capacity, access and consumption per gross domestic product (GDP) significantly exceed that of all other countries. While South Africa exports some electricity, the majority of generation is non-renewable and accounts for over 80% of all electricity produced in the continental SADC. Electricity access and consumption in the rest of the SADC is generally very low and correlates with GDP per capita. Biomass, used directly for heating, dominates energy supply in the region. summarises population, GDP and the supply and demand of electricity in the region (Merven et al., Citation2010; IRENA, Citation2011; IEA, Citation2013).
Electricity access rates are very low in many countries – particularly further north, with Malawi (not tabulated) the lowest at 9%. In most cases where electricity access is low, the internal electricity generation and capacities are characterised by high renewable fractions, mostly due to hydropower. The Democratic Republic of Congo, Mozambique and Zambia all generate in excess of 99% of electricity by renewables (IRENA, Citation2011). Countries bordering South Africa (Namibia, Botswana and Zimbabwe) as well as Angola have varying levels of renewable electricity production and all rely on imports for some or most electricity; largely from South Africa. The amount of electricity from solar technologies is negligible (IEA, Citation2013).
2.2 Considerations for future: energy resources and technologies
Coal is currently the most utilised primary energy source for electricity amongst the continental SADC countries. South African coal power plants alone produce approximately 70% of all the electricity in this region (IRENA, 2013). South Africa has the majority of all of Africa's known coal reserves; however, recent geological surveys for coal in the region suggest a sharp decline in the estimate of economically extractable coal reserves (Rutledge, Citation2011; SACRM, Citation2013). More recently, statistical methods first proposed by Hubbert (Citation1956) that predicted peak oil in the continental USA accurately have been refined by various authors, including Rutledge (Citation2011), and applied to the prediction of coal reserves and extraction rates for all coal regions including Southern Africa. The results generally indicate that this region is already near peak annual production and production will decline to 90% ultimate cumulative extraction by mid-century (Rutledge, Citation2011; Gauché et al., Citation2013). These newer, much lower extraction estimates are understandably not taken for granted. The South African Coal Roadmap of 2013 acknowledges these statistical models, but suggests that sufficient reserves exist for a longer period. However, due to worldwide demand growth, supply of coal to regional utilities could experience supply shortfalls as soon as 2018 (SACRM, Citation2013). Similar arguments can be made for reserves of gas, oil and nuclear fuels that all show signs of economically feasible production declining due to factors such as the cost of extraction, cost of carbon penalties and other uncertainties regarding safety and the environment (Gauché et al., Citation2013). In general, the import price of oil, coal and gas has increased by a factor of about five over the last 10 years, ignoring the high peak prior to the worldwide economic downturn of 2008 and the resulting drop in prices (IEA, Citation2013).
The capital cost for conventional power plants based on the South African IRP (DoE, Citation2011) range from about US$600/kW for open cycle gas turbines (OCGTs) to US$2500 to 3500/kW for supercritical coal with and without carbon capture and sequestration respectively, to US$5000/kW for nuclear plants. The target cost of electricity for conventional utility-scale power generation is in the range of US$0.05 to 0.10/kWh (IRENA, Citation2013a).
In the SADC region, diesel is extensively used for emergency, peaking and off-grid application. In general, these generators are used as a last resort due to the cost of fuel. In South Africa, large diesel-powered gas turbine plants have been installed as a stop-gap measure for mitigating recent reserve margin problems. The IRP (DoE, Citation2011) adds more of this generating type due to flexibility, capital cost and speed of deployment of these generators. Assuming unsubsidised diesel prices, the cost of electricity exceeds ZAR 5.00/kWh (US$0.50/kWhFootnote4) (Silinga & Gauché, Citation2013). Smaller generators used in remote, off-grid and emergency situations are assumed to result in similar or higher costs of electricity (IRENA, Citation2013b).
Most renewable energy technologies are expected to benefit from cost reduction due to technology learning. As example, the multi-decade learning rate of solar PV modules exceeds 20% and experts do not anticipate a slowdown in this learning rate to occur soon (IRENA, Citation2012a). The following summary of renewable energy costs is intended to only broadly contextualise the options for this study, and CSP will be covered in more detail in the following section. Most of the information is sourced from IRENA (Citation2012a, Citation2012b, Citation2012c, Citation2013a, Citation2013b).
With exceptions such as hydropower, renewable power generation is historically characterised by high capital costs and no or low fuel costs.
Capital costs for biomass power-generation technologies vary significantly in part due to lack of large-scale experience. Feedstock costs are also variable and not necessarily limited to zero in the case of waste products. Sustainability, transport and higher than average operating and maintenance costs are all identified as concerns. In the context of this paper, biomass can play an important role both because of the potentially significant resource and the potential for hybridisationFootnote5 with CSP.
Hydropower is the most utilised renewable power-generation source worldwide. When good resources are available, it has traditionally been the cheapest source of electricity and is expected to remain so. The capital costs for typical large-scale projects range from US$1500 to 2500/kW and as low as US$500/kW when reservoirs exist. Hydropower also offers flexibility due to turbine ramping rates and reservoir storage, and perhaps importantly in our context offers ‘blackstart’ assistance – the ability to resume provision of electricity to the grid in the event of a total grid shutdown. The potential for hydropower in the SADC is vast and under-utilised. The Democratic Republic of Congo has an estimated potential of 100 GW, with a single-site (Grand Inga) potential of 44GW representing the best site worldwide with steady capacity all year (Merven et al., Citation2010).
Wind power has matured to the point of being competitive with fossil fuel alternatives. Capital costs for onshore wind power are typically in the region of US$2000/kW but have been as low as US$1300/kW in China in 2010. PV power is also showing signs of reaching grid parity, with PV module prices dipping below US$1.0/W in early 2012. The high learning rate for PV power does not apply to the balance of the plant, which now is increasingly becoming significant, but PV system costs are nevertheless dropping and also in the region of US$2000/kW. Intermittency is the drawback to wind and PV power, but as long as the cost of electricity is competitive and dispatch solutions such as hydropower and CSP are available, these technologies will play an increasing role in the SADC.
To compare the cost of conventional and renewable energy technologies, the widely accepted measure is the levelised cost of electricity (LCOE). In its simplest form, the LCOE is determined by dividing all expected lifetime costs of the plant (capital, operating, maintenance and fuel) weighted by the opportunity cost (discount rate) over time by the total expected lifetime electricity output of the plant weighted by the same method. presents typical ranges for the LCOE and learning rates for the technologies applicable to the SADC.
Figure 2: LCOE ranges and learning rates for electricity options in the SADC
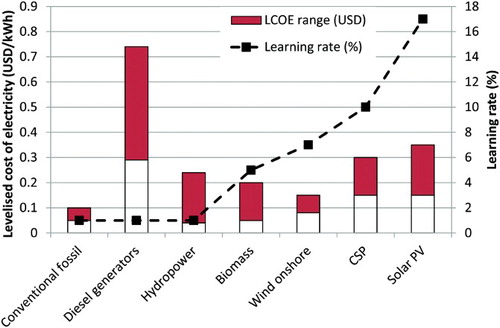
The generally accepted benchmark for competing in the electric market is to compete against the low-cost mature conventional technologies. The US Department of Energy target is set at US$0.06/kWh (Kolb et al., Citation2011). Most SADC countries rely on diesel generators for emergency or remoteness reasons that result in high electricity costs, or, as is the case for much of the SADC, the majority of the population has no access to electricity. The implication is that for the SADC the marginal cost of electricity should be especially important in considering the competitiveness of renewables. While all feasible renewable technologies except for hydropower are more expensive than conventional benchmarks, they are all below the cost of diesel generators. Their respective learning rates suggest that renewables will continue to be the right choice for much of the region moving forward.
The Southern African Power Pool scenario models reported by IRENA (2013c) show a decline in the use of fossil power in most scenarios. In the period 2030–50, a sudden shift towards CSP occurs. The shift towards CSP commences earlier and results in more generation of electricity when the Inga project is not factored in, although to 2030 most scenarios have little to no CSP capacity. The method uses least-cost optimisation of a multi-year bottom-up (technology behaviour) energy system. Each country is modelled as a single node interlinked with transmission lines. Technology performance (capacity factor and efficiency), cost, learning rates and basic principles for time of day, week and impact of seasons are inputs to the model. The model does not account for real spatial–sequential weather and resource due to the already high computational burden. Similarly, the 2013 draft revision of the South African IRP (DoE, Citation2013a) shows that while the CSP allocation is likely to grow for a number of reasons including risk of fuel availability, the uptake of the technology only occurs at the very end of the period to 2030 according to similar system models. The document suggests that the allocation be moved forward, however, to fulfil the commitment to national goals of carbon dioxide reduction and the move to a green economy (NPC, Citation2011; DEA & UNEP, Citation2013).
The analysis that underpins this paper uses a more detailed spatial–sequential model (Gauché et al., Citation2012), which can be considered complimentary to the aforementioned systems models as it only considers individual scenarios for a system.
3. Concentrating solar power technology and value
CSP is the least mature large-scale renewable technology, only recently (2011) exceeding 1 GW of installed capacity worldwide. The oldest running CSP plants were commissioned between 1984 and 1991 in California and it took almost another 20 years before new plants were commissioned (IEA, Citation2010a; IRENA, Citation2012b). At this time over 2700MW of CSP capacity is operational, including the recently commissioned 392 MW Ivanpah central receiver plant in California and the 280 MW Solana parabolic trough plant in Arizona (NREL, Citation2013). CSP capacity has more than doubled since reaching the 1000 MW threshold in 2011.
illustrates the basic principles of a state-of-the-art molten salt central receiver system with storage. Similar principles govern all CSP technologies but the configuration and capabilities of plants can differ widely. The charging cycle consists of the optical (sunlight) to thermal conversion and storage. Solar irradiation is converted to high-temperature thermal energy by making use of reflectors, typically mirrors that focus sunlight to a line or a point. Thermal energy is either stored for later use in molten salt tanks or directly used for power generation. Most new utility-scale CSP plants have thermal energy storage systems capable of maintaining turbine operation for several hours at full turbine output.
Figure 3: Schematic layout of a two-tank molten salt-based central receiver CSP plant
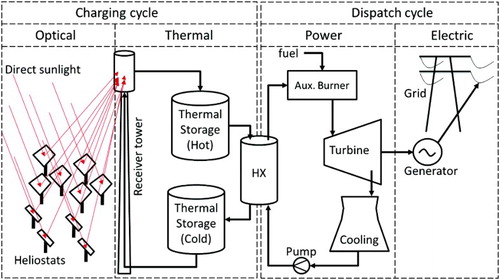
The dispatch cycle discharges the thermal storage through a steam generator heat exchanger () to produce superheated steam. From this point, the cycle is very similar to a conventional coal power plant where a steam turbine produces power and the low-pressure steam is condensed (cooled) before it is pumped back to the steam generator.
The charging and dispatch cycles are independent of each other provided there is a general balance between them over a 24-hour period. Two fundamental principles differentiate CSP storage from storage for other intermittent renewables. Firstly, thermal storage is commercially demonstrated at utility scale with very high net efficiencies, typically in excess of 99%, making this type of energy storage the most efficient type (IEA, Citation2010a). Secondly, while thermal storage is lower in cost than many other storage options, the real cost value relates to the separation of the charge and discharge cycle, which permits a trade-off between the storage capacity and turbine rating. All other things equal, when adding storage to increase the capacity factor of a CSP plant, the turbine rating is decreased accordingly to give about the same cumulative electricity generation over a period of time. When the marginal capital cost of the turbine and storage are similar, increasing storage in a CSP plant does not increase the cost of the plant. In the context of South Africa and the region, it is unlikely that turbine equipment will be produced locally but an increasing share of thermal storage systems is likely to be locally sourced in time. Thus a double benefit potentially exists for CSP with storage: good flexibility for dispatch coupled with increased local participation.
In addition to storage, the plant is able to generate high-quality steam more rapidly and over a wider range of output than in a coal boiler due to the immediate availability of thermal energy and absence of coal boiler instability at part load. These two characteristics make CSP dispatchable, allowing for electricity to be produced to match demand. Dinter & Mayorga Gonzalez (Citation2013) present power generation performance for Andasol 3, a 50 MW utility-scale grid connected parabolic trough CSP plant with a thermal storage system capable of producing full turbine output for 7.5 hours without sun. The operational flexibility of Andasol 3 is demonstrated with examples of producing baseloadFootnote6 electricity, continuous supply of electricity in poor weather, response to power reduction requests (without curtailment) and profit maximisation for time of day pricing.
A variety of CSP technologies exist that can generally be placed into four categories; as depicted in .
Table 2: CSP categories summarising strengths, drawback and relevance to the SADC
While capital costs are expected to drop significantly for CSP, the technology is currently capital intensive. In 2011, the installed cost ranged from around US$4600/kW for a parabolic trough without storage to US$10 500/kW for a central receiver with up to 15 hours of storage (IRENA, Citation2012c). Fichtner (Citation2010) claims that more than 80% of the LCOE for parabolic troughs and central receivers is in the cost of capital, which will be true for deployment in the SADC region. As much of the region claims excellent solar resources, the LCOE can be as low as US$0.14 to 0.18/kWh despite these capital costs. presents the general breakdown of capital and construction cost of a typical existing Spanish 50 MW parabolic trough plant with 7.5 hours of storage (E&Y & Fraunhofer, Citation2011) and an expected commercial 100 MW central receiver with 9 hours of storage (Kolb et al., Citation2011).
Figure 4: Cost fractions for (left) 50 MW parabolic trough, 7.5 hour storage (E&Y & Fraunhofer, Citation2011) and (right) 100 MW molten salt central receiver, 9 hours storage (Kolb et al., Citation2011)
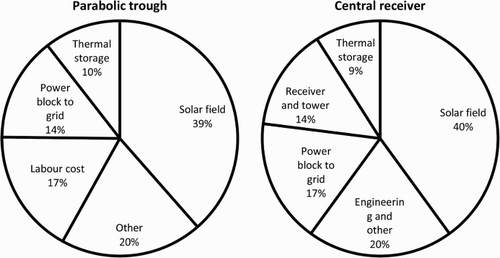
Central receiver systems are generally expected to lead to the greatest cost reduction in CSP (Kolb et al., Citation2011; IRENA, Citation2012b), and while Kolb et al. (Citation2011) suggest a roadmap for central receiver technology achieving US$0.08/kWh, the same authors cite the US Department of Energy SunShot program to drive down the cost of CSP to US$0.06/kWh for systems with very high capacity factors.
While CSP appears to have a strong case, several factors have been identified that could prevent a full realisation of the value proposition. In short, local economic benefit requires a certain minimum annual capacity to be committed for a sustainable period of time. Such a commitment could trigger an internal self-sustaining CSP industry that realises all of the preceding value proposition factors. Less than the minimum threshold could result in a holistically less competitive CSP industry reliant on higher levels of importing. Gauché et al. (Citation2013) argue that, in this instance, South Africa risks a more vulnerable electricity supply system as all other supply guarantee solutions have potential short-term to long-term risks mostly related to fuel price vulnerability and availability.
CSP is still an emerging technology with less than 3 GW of installed capacity worldwide. According to E&Y & enolcon (Citation2013), ‘the industrial landscape provides the opportunity for South Africa to be a first mover’ as a regional industrial and research and development hub. In conclusion, the report suggests that the most important priority is for the government to review the allocation for CSP to provision peak electricity by considering a two-tier tariff structure including a premium during peak hours. Coincidentally, a two-tier price tariff was announced during 2013 and two projects have been approved, increasing the CSP allocation by 200 MW. The tariff base price payable for 12 hours per day is ZAR 1640/MWh (about US$160/MWh) with a 270% peak premium for 5 additional hours per day (DoE, Citation2013b).
Gauché et al. (Citation2012) proposed a value proposition of CSP for South Africa, which argues that CSP is the only known viable electricity dispatch technology that does not suffer from potentially serious fuel supply or cost vulnerability; as soon as the 2030 horizon. This argument also implies that prioritising coal and nuclear in the original IRP (DoE, Citation2011) for a stable baseload is potentially paradoxical and this appears to be echoed in the draft revision (DoE, Citation2013a). In addition, CSP in combination with other renewables offers a very high degree of local inclusion due to the better match with existing manufacturing capabilities and the distributed nature of the technology. When taking the background and context of this paper into account, the value of CSP now and with the future in mind could be represented by a multi-dimensional spider plot as qualitatively demonstrated in .
The comparison between electricity generators takes technology and regional context into account. It is therefore only intended as a qualitative comparison and subjectivity is unavoidable. As an example, PV power could be argued to be the best short-term opportunity for electrification in the SADC due the technology's highly flexible implementation value, but this is not conclusive. A scenario whereby independent power producers construct CSP plants in neighbouring countries to supply both self-consumption and South Africa with evening peak power could be similarly viable. The host country economically benefits with little or no in-country cost in the CSP case, whereas the small distributed PV case may struggle with finance and security.
It is not difficult to argue the strength of the sustainability and potential of the solar resource. Hydropower has a very clear value in terms of resource, cost and dispatch, provided security can be addressed. Industrial potential and employment inclusiveness (defined as offering non-centralised employment opportunities) ranges, widely favouring renewables.
CSP plants are currently expensive to construct and have a reasonably high (low-scoring) LCOE. Due to dispatchability, however, the LCOE impact to the system is good because existing dispatch solutions other than hydropower rely on high-cost diesel generation. In summary, other than the related coupling between LCOE per plant, technology maturity and capital cost, CSP is by these measures a very good fit for South Africa and the region.
4. Concentrating solar power scenario models for South Africa
Gauché et al. (Citation2012) developed and validated a first-principle CSP model that predicts hourly performance of CSP plants with thermal storage and hybridisation. The model has been validated using performance data for existing CSP plants and developed for large multi-plant systems simulations and optimisation.
4.1 Baseload case
To test the model in a large system, the authors decided to test the ability of a distributed fleet of CSP plants to provide system baseload electricity, defined as the ability to produce electricity at one system output level all year. The model plant had the same specification as the Gemasolar plant in Spain, a system with 15 hours of thermal storage at full load and no cost reduction from scale or learning. The plant was replicated across the whole of South Africa separated by 0.375° latitude and longitude, resulting in 823 Gemasolar-like plants.
The basic results are summarised as follows:
The LCOE for individual plants ranged from ZAR 1.48/kWh (US$0.15/kWh) in Upington to ZAR 2.01/kWh (US$0.20/kWh) in Stellenbosch conditions, which are very similar to those of the original plant.
Baseload can be achieved when managing the dispatch with care. When one-quarter of all plants are producing full power at any one time, representing the equivalent of 4 GW of capacity, power output is constant for all but a few hours of the year and never dips below 75% output. This is illustrated by the full year output in , noting that the event in late August 2005 is a solar data malfunction. This case is only a theoretical exercise and demonstrates an inefficient use of CSP. A high amount of energy is unused to ensure this baseload leading to an LCOE of about R5.00/kWh (US$0.50/kWh). Additionally, because no consideration was given to optimal plant location, this scenario could be considered a reasonable worst case.
Individual plants have multiple days without output if allowed to operate independently and without time-of-day pricing, whereas the system of plants can produce power throughout the year if operation is managed. Initial analysis of the results suggests that a more geographically distributed system is needed if stability of supply is desired. While not yet quantified, this does potentially imply reasonable separation of plants or groups of plants separated in east–west directions and, to some extent, in north–south directions. Only the region east of the South African escarpment appears to be a poor choice for location by the nature of the resource.
A tangential discovery is that a fleet of dispatch-capable CSP plants can within reason be controlled to suit different needs. While this is not viable inter-seasonally without additional cost, it did stimulate the follow-up studies to identify usage opportunities for CSP.
Figure 6: Baseload case power output over a full year using solar data from 2005
Note: Anomalous data in late August due to satellite data malfunction.
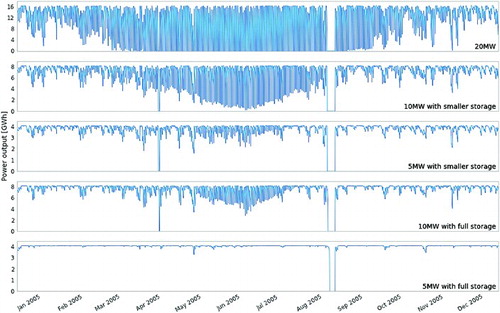
4.2 Peak electricity
The avoided cost of electricity, that is the cost of the more expensive generators used as a last resort, should present an opportunity for CSP given the technology's flexibility in dispatch. In South Africa, diesel-powered OCGT plants are increasingly used for this purpose. According to Silinga & Gauché (Citation2013), the LCOE of these plants is over ZAR 5.00/kWh (US$0.50/kWh) without real fuel price increases. The previous analysis suggests that a poorly configured CSP system of plants could match this cost, suggesting that a system optimised for the task will reduce the avoided cost. The proposition is that a fleet of contemporary CSP plants at current expected capital costs can be optimised to reduce the cost of peak electricity in the short term.
The IRP allocates over 5000 MW of additional OCGT capacity up to 2030. Assuming that the total fraction of dispatch solutions reasonably represents the South African peak needs, an annual hourly peak demand profile was generated assuming that anything above 90% of the daily peak would be defined as peak power. The study assumes that no major new infrastructure is possible in the short term and therefore a fleet of CSP plants needs to be close to existing high-capacity sub-stations. The same system model was adapted to handle dispatch according to any required demand and various scenarios were tested.
The favoured scenario consists of 3300 MW of optimised CSP capacity distributed along the high-capacity line to Cape Town, producing about 80% of the peak needs. In , the larger circular shaped areas show suitable land close to existing infrastructure. The extent and distribution of these sites fortuitously allows for a near ideal system. The remaining 20% is guaranteed by the same fleet of OCGT plants. Assuming the same costs of fuel and using the base cost values in Kolb et al. (Citation2011), the LCOE of the combined peaking solution drops to ZAR 2.78/kWh (US$0.28/kWh). In , real fuel price inflation is added to test sensitivity of the LCOE. Scenario 1 is the baseline of OCGT plants and Scenario 3 is the favoured virtual hybrid (separate CSP and OCGT) system. The combined system offers a peak guarantee, is significantly lower in cost, and is largely insensitive to price volatility.
Figure 7: Model locations (small black circles) and identified suitable land (large circular black regions) for a short-term rollout of CSP peaking plants
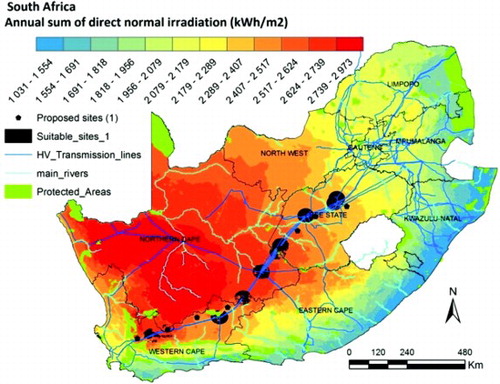
Figure 8: Peaking electricity costs for diesel OCTS with and without CSP fuel savers
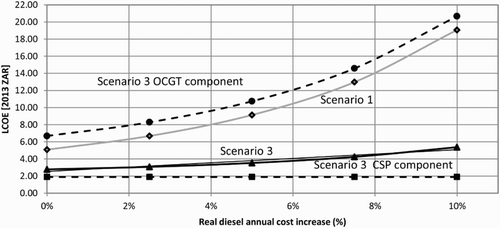
An analysis of how this system could be affordable reveals that plants need to be constructed in as distributed manner as possible, because grouping all plants in one sub-region (larger circular areas in ) leads to significant under-production and over-production due to the influence of weather systems. In turn, this causes under-utilisation of capital and a higher LCOE.
By extending this concept, the sunny regions around the SADC would probably drop the cost of electricity further all other things equal, and a further proposition is that South Africa and its neighbours would jointly benefit from such expansion.
5. Approaches to realise the economic benefits of CSP in South Africa and the region
The IRP is intended to be a continuously updated process and legally needs to be implemented. Systems analysis has been used to specify the generation type and capacity each year in a 20-year horizon. Very detailed analysis of options and scenarios, while increasingly important during a transition to a lower carbon, resulted in a narrowly prescriptive plan in its 2011 release. A generous allocation of renewables was included, amounting to 17.8 GW or 42% of new capacity in 2030. Nuclear power has been prioritised as a matter of security of supply due to concerns that PV and wind power will not provide. The full allocation of PV power and the majority of wind power are assumed to require backup generation using dispatch solutions such as the diesel-powered OCGTs, pumped storage and imported hydropower. In the event that the internal supply of gas is sufficient enough in a reasonably short period to power future dispatch plants and/or imported hydropower is realised, this prescription could be sensible. Under different circumstances, the current allocation of renewables could be viewed as virtually tokenistic and unnecessarily expensive.
A number of arguments can be made for risk contingencies in the electricity plan for the region and in the IRP. Northern hydropower may not materialise despite the excellent resource and due to regional political instability. Transmission infrastructure already connects SADC countries and would need to be improved for the realisation of hydropower. The cost burden of this infrastructure could be shared between distributed solar and the hydropower projects. Nuclear power has long lead times and there is uncertainty regarding cost. This poses unnecessary risk for sustained reserve margin in the future. Shale gas might emerge as a cost-effective resource in South Africa, but not for the next decade. Coal availability has several risk factors, not the least market pressure for export.
These contingency risks are generally reflected in the draft IRP revision (DoE, Citation2013a) and both solar energy technologies are beneficiaries. CSP is specifically identified as a dispatch solution to replace some reliance on coal, nuclear and gas. In all scenarios of the draft IRP revision, however, CSP capacity implementation only occurs at the very end of the IRP horizon due to the learning rate only making it economically viable then. The document calls for the CSP capacity to be moved forward into a gradual rollout and in recognitions of the priorities of the green economy (NPC, Citation2011) despite the costs.
This dilemma is becoming more widely accepted; CSP is emerging to be an attractive electricity solution for the region, but cost and a limited annual rollout may prevent the regional broader benefit of economic participation. Two approaches are suggested to address this dilemma.
5.1 First approach: Rapidly commence with a hybrid OCGT and CSP peaking system
CSP is immediately competitive as a fuel saver to existing and future diesel-powered OCGT plants, and a CSP peaking system can be deployed with existing transmission infrastructure. The capital cost and construction time of OCGT plants are low and the plans for these plants should be maintained for supply security reasons. A fleet of CSP plants optimised for provision of peak power should be rolled out incrementally. This fleet has a capacity of over 3 GW and saves 80% of the fuel costs of the OCGT plants. The cost savings incrementally finance the ongoing CSP rollout such that as each CSP plant comes online, it adds to the fuel savings.
This virtual hybrid system has an additional quality due to the number of independent dispatch units in the system. It offers a high degree of robustness for any use ranging from the supply of sharp peaks to the moderation of steep ramp changes in PV and wind power. It could support baseload power if needed. This could be considered valuable in uncertain future periods but in normal times simply provides flexibility to avoid more expensive generation options.
This approach is characterised by the use of imported CSP technology, increasing local content and an initially high cost per plant. By phasing the rollout of the CSP fleet, the capital costs are not incurred at once, the risks associated with the plants are reduced and the system benefits from a learning rate. The capacity of over 3 GW can be delivered in 10 years with about 400 MW per year commencing construction that, if maintained, will exceed the threshold for local suppliers to complete.
As this hybrid system is competitive against current peaking costs, the plan might not need specific interventions except to allow CSP to play this role. The public are not exposed to the capital risk of the projects in the event that this fits into the Renewable Energy Independent Power Producer Procurement Programme. National training and research and development activities would probably need to be supported at a much higher level in order to provide a local supply of skills and supplies.
This approach probably results in an ecosystem prepared to move to the next level of CSP rollout. A decade of CSP learning would have occurred and if the entire fleet is delivered a sense of the appropriateness of the technology will be given. Intellectual property ranging from improvements to manufacturing, new components and new plant concepts could begin to position South Africa as a first mover.
5.2 Second approach: Spread into the SADC and start to supply base electricity with other renewables
While the first approach is implemented, more certainty will emerge regarding the real or political impacts of climate change, impact of wind and PV power, availability of hydropower from the north, shale gas and decisions regarding a nuclear fleet.
A system that is more widely distributed is likely to be more cost effective, particularly to address the apparent imbalance between summer and winter supply and the demand for wind and solar power. Neighbouring sunny countries such as Namibia and Botswana are unlikely to benefit significantly from industrialisation of CSP but these countries have excellent solar resources particularly in winter due to their latitude. Expanding the distributed CSP fleet into these countries could benefit the whole of the SADC:
South Africa will have access to a more cost-optimal dispatch solution and will serve as a major industrial supplier to the plants.
Neighbouring countries could earn export revenue and simultaneously increase internal electricity access rates and employment.
If northern hydropower projects become more likely, better transmission infrastructure might already be in place.
In the event that CSP succeeds through the first approach and has a reasonable learning rate, a crossover in the cost of electricity between most of the renewable technologies and coal power could occur through this approach. A similar argument can be made about nuclear power, particularly if plant capacity factors reduce with increasing renewable fractions.
Without sufficient reserve capacity, South Africa will need policy interventions to keep coal reserves for energy security even if world market prices are very attractive. In the event that sufficient reserve margin exists due to any combination of CSP and hydropower dispatch solutions, the ability to economically benefit from exporting can be exercised.
The latter implies that CSP in combination with other renewable systems begins to replace not just peaking electricity, but it also enters the space of other generators where it competes at the marginal cost level.
5.3 Beyond the two approaches
It is not possible to predict disruptive events that could and so often tend to happen. However, in the event that fossil supply limitations set in earlier than expected and sustainable nuclear power options are not deemed safe enough, predictions by IRENA (Citation2012b) and the draft IRP (DoE, Citation2013a) suggest that, in most scenarios, solar power becomes the dominant supply of electricity in the period between 2030 and 2050. The annual new capacity required in this period probably requires a sustained and perhaps increased build effort. In order for solar energy to be the primary supply of electricity at that time, the cost of supplying the capacity could be considerable and could place pressure on trade balances unless it substantially came from industry within the SADC due to the first few phases.
6. Conclusions
This paper suggests the means for the South African CSP industry to reach critical mass, by applying CSP to compete against the most expensive electricity generators in South Africa (first approach), and to expand this into other SADC countries (second approach).
If South Africa takes action through the first approach and rolls out a robust virtual hybrid electricity guarantee system, reserve margin can be increased while dropping the cost of electricity in the whole electricity system, and this can trigger the critical mass to sustainably create an industry. If provision is made in the IRP to allow CSP to complete for the dispatch or peaking category without reducing capacity of OCGTs, the public takes little risk. With increasing reserve margins, the ability to exploit the opportunity cost of coal by export becomes more tangible. Regardless of reserve estimates, coal demand will continue to increase worldwide.
While all assumptions require close scrutiny, the addition of over 3 GW of CSP has many significant benefits that appear to vastly outweigh the risks.
The only important intervention now is facilitating CSP capacity to the fullest extent in the provision of peaking electricity. Awareness due to the scale of the rollout will probably lead to government interest in investing in training, research and industry initiatives.
In making this provision, the gap between the world-class solar resource and its exploitation to the benefit of the region can be narrowed.
Notes
4Assuming US$1.00 = ZAR 10.00.
5CSP plants typically use conventional steam turbines making it viable to use multiple sources of energy, referred to as hybridising or hybridisation (IEA, Citation2010a).
6Baseload is the term used for electricity generation to meet minimum demand over a period of time and is usually supplied by large efficient baseload power plants characterised by high-capacity factors (high number of operating hours at full load per year).
References
- DEA & UNEP (Department of Environmental Affairs & United Nations Environment Programme), 2013. Green Economy Modelling Report for South Africa: Focus on the Sectors of Natural Resource Management, Agriculture, Transport and Energy. South African Department of Environmental Affairs, Pretoria. https://www.environment.gov.za/sites/default/files/docs/greeneconomy_modelingreport.pdf Accessed 24 October 2013.
- Dinter F & Mayorga Gonzalez D, 2013. Operability, reliability and economic benefits of CSP with thermal energy storage: First year of operation of ANDASOL 3. Paper rpesented at the Solar Power and Chemical Energy Systems Conference (SolarPACES 2013), 17–20 September, Las Vegas, NV.
- DoE (Department of Energy), 2011. Integrated Resource Plan for Electricity (IRP): 2010–2030. Revision 2. http://www.DoE-irp.co.za/content/IRP2010_2030_Final_Report_20110325.pdf Accessed 30 July 2012.
- DoE (Department of Energy), 2013a. Integrate Resources Plan for Electricity (IRP): 2010–2030. Update Report 2013. http://www.DoE-irp.co.za/content/IRP2010_2030_Final_Report_20110325.pdf Accessed 4 December 2013.
- DoE (Department of Energy), 2013b. Renewable Energy IPP Procurement Programme, Bid Window 3 Preferred Bidders’ Announcement, 4 November. http://www.record.org.za/resources/doc_download/79-department-of-energy-list-of-ipp-preferred-bidders-window-3-04nov2013.pdf Accessed 12 December 2013.
- E&Y (Ernst & Young) & enolcon, 2013. Assessment of the Localisation, Industrialisation, and Job Creation Potential of CSP Infrastructure Projects in South Africa – A 2030 Vision for CSP. http://0101.nccdn.net/1_5/1c8/164/360/GIZ-CSPStudy-FinalReportJune2013.pdf Accessed 27 November 2013.
- E&Y & Fraunhofer (Ernst & Young & Fraunhofer Institute for Solar Energy Systems), 2011. MENA Assessment of the Local Manufacturing Potential for Concentrated Solar Power (CSP) Projects. Final Report. The World Bank, Washington, DC.
- Fichtner, 2010. Technology Assessment of CSP Technologies for a Site Specific Project in South Africa Final Report. The World Bank and ESMAP, Washington, DC.
- Gauché, P, Pfenninger, S, Meyer, AJ, von Backström, TW & Brent, AC, 2012. Modeling dispatchability potential of CSP in South Africa. Paper rpesented at the Southern African Solar Energy Conference (SASEC 2012), 21–23 May, Stellenbosch, South Africa.
- Gauché P, von Backström TW & Brent AC, 2013. A concentrating solar power value proposition for South Africa. Journal of Energy in Southern Africa 24(1), 67–76.
- GeoModel Solar, 2013. SolarGIS Solar Radiation Maps. http://solargis.info/doc/87 Accessed 8 December 2013.
- Grobbelaar S, Brent AC, and Gauché P, 2013. Developing a competitive CSP industry in South Africa: Current gaps and recommended next steps. Development Southern Africa 31(3), 475–93. doi: 10.1080/0376835X.2014.891971
- Hubbert, MK, 1956. Nuclear energy and the fossil fuels. Drilling and Production Practice, 7–25. American Petroleum Institute, Houston, TX. http://www.hubbertpeak.com/hubbert/1956/1956.pdf Accessed 17 February 2012.
- IEA (International Energy Agency), 2010a. Technology Roadmap: Concentrating Solar Power. http://www.iea.org/papers/2010/csp_roadmap.pdf Accessed 30 July 2012.
- IEA (International Energy Agency), 2010b. Technology Roadmap: Solar Photovoltaic Energy. http://www.iea.org/publications/freepublications/publication/pv_roadmap.pdf Accessed 8 December 2013.
- IEA (International Energy Agency), 2013. Key World Energy Statistics 2013. http://www.iea.org/publications/freepublications/publication/KeyWorld2013.pdf Accessed 29 November 2013.
- IRENA (International Renewable Energy Agency), 2011. Renewable Energy Country Profiles. http://www.irena.org/DocumentDownloads/Publications/CountryProfiles_Africa_WEB.pdf Accessed 30 November 2013.
- IRENA (International Renewable Energy Agency), 2012a. Renewable Energy Technologies: Cost Analysis Series, Vol 1: Power Sector, Issue 4/5 Solar Photovoltaics. http://www.irena.org/DocumentDownloads/Publications/RE_Technologies_Cost_Analysis-SOLAR_PV.pdf Accessed 8 December 2013.
- IRENA (International Renewable Energy Agency), 2012b. Renewable Energy Technologies: Cost Analysis Series, Vol 1: Power Sector, Issue 2/5 Concentrating Solar Power. http://www.irena.org/DocumentDownloads/Publications/RE_Technologies_Cost_Analysis-CSP.pdf Accessed 8 December 2013.
- IRENA (International Renewable Energy Agency), 2012c. Renewable Power Generation Costs: Summary for Policy Makers. http://www.irena.org/DocumentDownloads/Publications/Renewable_Power_Generation_Costs.pdf Accessed 30 November 2013
- IRENA (International Renewable Energy Agency), 2013a. Renewable Power Generation Costs in 2012: An Overview. http://www.irena.org/DocumentDownloads/Publications/Overview_Renewable%20Power%20Generation%20Costs%20in%202012.pdf Accessed 30 November 2013.
- IRENA (International Renewable Energy Agency), 2013b. Southern African Power Pool: Planning and Prospects for Renewable Energy. http://www.irena.org/DocumentDownloads/Publications/SAPP.pdf Accessed 30 November 2013.
- Kolb, GJ, Ho, CK, Mancini, TR & Gary, JA, 2011. Power tower technology roadmap and cost reduction plan. Sandia Nation Laboratories, Albuquerque, NM. Report SAND2011-2419.
- Merven B, Hughes A & Davis S, 2010. An analysis of energy consumption for a selection of countries in the Southern African Development Community. Journal of Energy in Southern Africa 21(1), 11–24.
- NPC (National Planning Commission), 2011. National Development Plan: Vision for 2030. http://www.npconline.co.za/ Accessed 24 October 2013.
- NREL (National Renewable Energy Laboratory), 2013. Concentrating Solar Power Projects. http://www.nrel.gov/csp/solarpaces/ Accessed 8 December 2013.
- Rutledge, D., 2011. Estimating long-term world coal production with logit and probit transforms. International Journal of Coal Geology 85, 23–33. doi: 10.1016/j.coal.2010.10.012
- SACRM, 2013. The South African Coal Roadmap. http://www.fossilfuel.co.za/initiatives/2013/SACRM-Roadmap.pdf Accessed 1 December 2013
- SADC (Southern African Development Community), 2010. Regional Energy Access Strategy and Action Plan. Energy for Development (euei pdf) Project. http://www.euei-pdf.org/regional-studies/regional-energy-access-strategy-and-action-plan Accessed 10 December 2013.
- Silinga CN, Gauché P, 2013. Scenarios for a South African CSP peaking system in the short term. Paper presented at the Solar Power and Chemical Energy Systems Conference (SolarPACES 2013), 17–20 September, Las Vegas, NV.