Abstract
Every year, billions of migratory birds cover thousands of kilometers between their wintering grounds and their breeding areas. Along their migratory route, birds are often forced to break up their journey into several legs, mainly in correspondence with ecological barriers (e.g. seas and deserts), and stopover in areas where they can sleep and rebuild their energy stores. Stopover is a key migratory stage for many songbirds because strategies adopted at this stage may have direct bearing on the success of migration and, consequently, on the fitness of the individual. Although the restoration of energy reserves is likely the main goal for stopover in many cases, our recent studies suggest that there might be other reasons for stopping over, particularly after long non-stop flights. This seems to be the case of millions of songbirds that land on small coastal islands after crossing large waterbodies, although most of these sites do not provide sufficient refueling opportunities. Here, we aim to provide a new perspective on the role of such small islands by reviewing our recent findings on energy management in migratory songbirds. We are convinced that understanding the role of these islands for migratory birds, as well as how birds manage their energy at these sites, represents an important step toward an optimization of conservation strategies, since setting priorities for protection based on food availability alone may miss critical functions of stopover sites.
INTRODUCTION
Migration is the most energy-demanding life-history stage in the life cycle of migratory birds and it can cost up to 50% of the annual energy budget of an individual (Drent & Piersma Citation1990). Due to the high energy requirements to cross ecological barriers and the impossibility to carry enough energy reserves to complete their migratory journey in one leg (Klaassen Citation1996), many bird species are forced to break their migration in several legs, alternating long endurance flights with refueling stopovers (Schmaljohann et al. Citation2007). Given that the process of energy accumulation is slower than the energy consumption (Alerstam & Lindström Citation1990; Alerstam Citation2011), migratory birds can spend up to 80% of the total migratory period at stopover sites (Hedenström & Alerstam Citation1997). Indeed, this stage requires double the amount of energy that is invested in migratory flights (Wikelski et al. Citation2003; Schmaljohann et al. Citation2012) and, therefore, it makes the stopover phase particularly important for a successful migration.
Recovery from fatigue and restoration of energy reserves are often considered as the main purposes of stopover. Indeed, the amount of energy reserves – mostly subcutaneous fat which is the main fuel of migratory flights (McWilliams et al. Citation2004) – plays a key role in behavioral strategies during stopover, such as departure decision and refueling (Meissner Citation1998; Dierschke & Delingat Citation2001; Fusani et al. Citation2009; Goymann et al. Citation2010; Cohen et al. Citation2014; Deppe et al. Citation2015; Woodworth et al. Citation2015; Dossman et al. Citation2016). At stopover sites, as well as when preparing for migration, birds experience a rapid fuel accumulation that results from a drastic increase in food intake (King Citation1961; Metcalfe & Furness Citation1984; Bairlein Citation1985), changes in diet (Bairlein Citation1990; Dingle Citation1996; Berthold Citation2003; Cooper-Mullin & McWilliams Citation2016) and modifications of metabolic pathways (Dingle Citation1996; Berthold Citation2003) that lead to an increased lipogenesis and, consequently to a rapid deposition of fat reserves. After their arrival at the stopover site, migrants need to make decisions that can influence their pace of migration (Nilsson et al. Citation2013; Gómez et al. Citation2017; Schmaljohann & Both Citation2017; Schmaljohann Citation2018). Because of differences in body condition at departure from previous sites, distance between sites, and weather conditions encountered during flight, the amount of remaining energy reserves is typically not homogenous among birds landing at stopover sites (Pilastro & Spina Citation1997). Energy management at stopover may vary depending on species (Ferretti et al. Citation2020b; Maggini et al. Citation2020), habitat quality of the site (Alerstam Citation2011; Cohen et al. Citation2012; McCabe & Olsen Citation2015; Ferretti et al. Citation2019a), and migratory period (Nilsson et al. Citation2013; Horton et al. Citation2016).
According to theoretical models, birds seek to optimize either total energy investment or overall time of migration (Alerstam & Lindström Citation1990). Individuals that adopt an energy-minimizing strategy, also called energy minimizers, should seek to reach an optimal amount of energy reserves before leaving the stopover site. However, this may require a considerable amount of time and slow down the pace of migration. On the contrary, time minimizers should aim at completing their migratory journey in the shortest possible time and therefore minimize stopover duration by leaving the stopover site if it does not guarantee a rapid accumulation of energy reserves (Hedenström & Alerstam Citation1997). The latter strategy seems to be more common during spring than fall (Nilsson et al. Citation2013), likely due to the high competition for early arrival during the pre-nuptial period (Smith & Moore Citation2005; Drake et al. Citation2014). Migrants that arrive with good energy stores will try to maintain their body condition and resume migration in the shortest time (Bairlein Citation1985; Biebach et al. Citation1986; Goymann et al. Citation2010), whereas birds with poor energy reserves will seek to restore their energy reserves by actively exploiting the site (Schmaljohann et al. Citation2013; Cohen et al. Citation2014). In the latter case, the interplay between physiological condition and the rate at which individuals accumulate energy stores – the so-called fuel deposition rate (FDR) – is the most important factor affecting behavioral decisions. Migratory birds need to maintain a high FDR in order to reduce stopover duration (Alerstam & Lindström Citation1990; Jenni & Schaub Citation2003; Alerstam Citation2011). Many studies have shown that birds experiencing a high FDR show a better body condition at departure (Carpenter et al. Citation1983; Lindström & Alerstam Citation1992; Fransson & Weber Citation1997; Schmaljohann & Dierschke Citation2005; Bayly Citation2006, Citation2007; Delingat et al. Citation2008; Schmaljohann et al. Citation2013). Moreover, FDR decreases with increasing body condition (Schmaljohann & Eikenaar Citation2017). FDR is affected by a number of environmental factors such as predation risk (Fransson & Weber Citation1997; Weber et al. Citation1998), competition (Moore & Yong Citation1991), weather conditions (Jenni-Eiermann & Jenni Citation1994; Schaub & Jenni Citation2001) and abundance of food resources (Bibby & Green Citation1981; Graber & Graber Citation1983). Although the restoration of energy reserves is likely the main goal for stopover in many cases, recent studies of our group indicate that there might be other reasons for stopping over, particularly in certain stages of migration, for example soon after crossing large ecological barriers. Here, we will review our recent findings about energy management in migratory passerines after crossing the Mediterranean Sea and provide a new perspective on the role of small islands for migratory birds. We will start by discussing why billions of birds stopover on islands that do not offer sufficient resources for an efficient refueling. Then, we will explore behavioral strategies to manage energy reserves after long flights, as well as how such strategies may vary in response to some physiological and environmental factors. Finally, we will discuss new perspectives on the key role of small islands for migratory birds, to better understand stopover ecology and site exploitation.
Barrier crossing: why do migrants land on small islands during sea crossing?
During migration, large waterbodies are major ecological barriers for billions of terrestrial birds. Due to the lack of landing opportunities encountered during such crossing, migrants engage in exhausting multi-hour flights that in case of nocturnal migrating birds may extend their travel into day time (Grattarola et al. Citation1999; Deppe et al. Citation2015; Adamík et al. Citation2016) and, consequently, may lead to an extreme depletion of energy reserves. In this ecological context, small islands, such as those in the Northern Gulf of Mexico (Moore et al. Citation1990) and in the Mediterranean Sea (Spina et al. Citation1993), gain a considerable importance for migrants by providing suitable sites to land. Regardless of their ecological requirements, a wide range of species stops on these islands (Moore et al. Citation1990; Spina et al. Citation1993) even when they are not far from the mainland and do not provide much variety of habitat type. Especially during northward migration, Tyrrhenian islands are good sites where to investigate site-exploitation strategies after sea crossing. The fact that most individuals stop despite having enough energy reserves to continue their migratory flight (Pilastro & Spina Citation1997) seems to indicate reasons for landing other than refueling, which challenges the common view that the main goal of stopover after barrier crossing is energy recovery (Carmi et al. Citation1992; Klaassen Citation1996; Aamidor et al. Citation2011). These islands are usually just a few kilometers from the coast and offer scarce opportunities for foraging and limited freshwater sources, which does not make them the most suitable sites for replenishing energy reserves. A recent study based on mark-recapture data showed that most of the individuals captured on the island of Ponza (40°55ʹN, 12°58ʹE) did not stop over for longer than 1 day and, in general, did not increase their body condition during this period with the exception of species that breed in habitats similar to those found on the island (Maggini et al. Citation2020). These findings indicate a strong dependency of refuelling on stopover habitat (Cohen et al. Citation2012; McCabe & Olsen Citation2015) and raise the question why all other species land at these sites although more suitable habitats are available a few kilometers away. Once landed, individuals that experienced an extreme depletion of energy reserves during the migratory flight will try to improve their condition by intensively refueling (Ferretti et al. Citation2019a) and carefully managing their energy reserves (Ferretti et al. Citation2019a, Citation2019b). However, most of them hardly increase their energy stores at these sites (Maggini et al. Citation2020) despite considerable investments in food searching (Cohen et al. Citation2012), which has negative effects on FDR (). For this reason, these individuals leave the site soon after landing (Schmaljohann & Eikenaar Citation2017), as confirmed by the relatively short time (41.3 hr, Goymann et al. Citation2010) that lean birds spend on these small islands. On the other hand, some individuals manage to reach these sites in good conditions. Despite they having an amount of energy reserves that would allow them to continue their migratory flight, they decide to stop for few hours (Goymann et al. Citation2010) before resuming migration on the following night. During their stay, fat individuals spent most of their time asleep (Schwilch et al. Citation2002; Ferretti et al. Citation2019b, Citation2020b), indicating a strong pressure to recover for sleep lost during the endurance migratory flight. The fact that these birds stop before reaching the coast, regardless of the presence of an optimal habitat, and mainly sleep during their permanence on these small islands, suggests a homeostatic regulation of sleep following endurance flight (Rattenborg et al. Citation2009) that leads to land at the first available area. Moreover, small coastal islands may host a low density of potential predators (Beauchamp Citation2004) and, for this reason, be optimal sites to stop when sleep recovery is the primary goal. Taken together, these evidences indicate that the use of islands may be less related to refueling than generally thought. Indeed, other physiological aspects seem to explain better than FDR why so many songbirds stopover at these sites after sea crossing.
Fig. 1. Rates of energy accumulation of migrating songbirds that spent at least 1 day on Ponza. Rates of energy accumulation (presented as “Boxplots”) were calculated based on bird’s body mass at last capture subtracted from body mass at first capture relative to lean body mass and time spent at the stopover site. To improve accuracy of this measurement, we used the number of hours between the first and the last capture as “time of permanence”. Lean body mass was estimated following Kelsey et al. (Citation2019). Data from Maggini et al. (Citation2020)
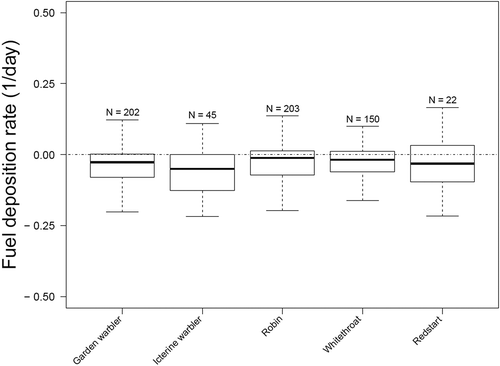
ENERGY MANAGEMENT ON SMALL ISLANDS
The role of locomotion
When migrants with heightened energy demands are concentrated in unfamiliar areas, competition for available food resources can reduce the rate at which they meet nutritional requirements (Carpenter et al. Citation1983; Moore & Yong Citation1991). In case birds land at a site that is suitable for refueling, a fast energy recover ybecomes the main goal of stopover (Alerstam & Hedenström Citation1998), and consequently birds initiate intensive foraging (Schaub & Jenni Citation2001). However, intense foraging may increase the exposure to potential predators (Lima & Dill Citation1990), therefore behavioral decisions aiming to optimize energy accumulation during stopover are supposed to be the result of a trade-off between energetic needs and predation risk. Several studies have confirmed this hypothesis (Sih Citation1980; Lima Citation1988; Pomeroy et al. Citation2006) and behavioral decisions can be summarized in a simple rule-of-thumb: migratory birds actively search for areas with abundant food (Cohen et al. Citation2012), however in case of high predation risks they move to safer stopover sites even though the new site may not guarantee a high FDR (Lima & Dill Citation1990; Brown & Kotler Citation2004), as may be the case of small coastal islands. For example, when released in a stopover site characterized by few food sources – a scenario similar to the one on small islands – Red-eyed vireos (Vireo olivaceus) moved further and searched for habitat types that could guarantee higher food availability (Cohen et al. Citation2012). Nevertheless, there are cases in which moving to different sites might be very hazardous, as for birds with very poor energy reserves. When leaving the site is not an option, the only alternative is to optimize energy consumption by the modulation of energy invested in locomotion. In a recent comparative study conducted on the island of Ponza, we showed species-specific responses to a gradient of food availability among songbirds. By using the amount of diurnal locomotion as a proxy for food searching behavior (Morton Citation1967; Astheimer et al. Citation1992; Fokidis et al. Citation2011), we found that a few species show an intense diurnal activity regardless of food availability, whereas most of the species increased locomotor activity with decreasing amount of food (Ferretti et al. Citation2019a). Although it appears plausible that the reduction of diurnal locomotion in response to food availability has an energy-saving function (Bäckman et al. Citation2016; Schofield et al. Citation2018) – as suggested by the comparison of metabolic data between migrating birds displaying different behaviors (Ferretti et al. Citation2019b), our current knowledge does not allow to rule out that reduction in locomotion is also functional to reduce potential predation risk. Indeed, birds that find limited food sources are expected to increase their activity in the attempt to improve refueling (Cohen et al. Citation2012; Ferretti et al. Citation2019a), although this strategy may increase their exposure to potential predators (Lima Citation1986; Lind & Cresswell Citation2006). From this perspective, the habitat homogeneity that characterizes most of small islands may be beneficial for birds after sea crossing. In fact, at these sites the lack of food sources is often counterbalanced by a reduced predation risk (Beauchamp Citation2004) which potentially allows to invest more time and energy into refueling. A lower predation risk on small islands might be attributed to two main factors: the size of the site and the number of individuals sharing the site. Small islands can support only a low number of resident predators (Del Monte-Luna et al. Citation2004), mainly due to the competition for food sources out of the migratory season and breeding spots (i.e. nest sites in the case of raptors). Although aerial predators might migrate or increase their home range, this is not possible for mammalians predators whose population is significantly affected by potential limitations due to the size of the island (Del Monte-Luna et al. Citation2004), an aspect that may reduce the overall predation risk. Migrating birds may also benefit from sharing the site with thousands of individuals. This reduces the risk of being caught thanks to an increased group alertness and a “dilution effect” (Sirot Citation2006) if attacked by the few predators present on islands. Taken together, the aforementioned reasons seem to make small islands optimal sites to stopover no matter if the priority is to replenish energy reserves or simply rest.
The role of sleep
Locomotion is not the only behavior that can be modulated in order to optimize energy investment. Sleep is a fundamental behavior of all organisms and occupies most of bird’s lives (Toates Citation1980). Even though the functions of sleep are still poorly understood, we know that animals during sleep can lower their metabolic rate by 15–20% compared to the wake phase (Stahel et al. Citation1984; Fontvieille et al. Citation1994; Jung et al. Citation2011; Markwald et al. Citation2013). The lower metabolic rate can be related to a small decrease in body temperature, facilitated by physiological mechanisms (i.e. vasoconstriction and piloerection) that reduce the body’s thermal conductance (Berger & Phillips Citation1995). Therefore, the modulation of sleep based on the need for physiological recovery can be a good strategy to optimize energy expenditure (Allison & Van Twyver Citation1970; Berger Citation1975). How birds sleep during migration remains a mystery, although several non-mutually exclusive hypotheses have been formulated (Rattenborg et al. Citation2004; Rattenborg Citation2006). Previous field studies on nocturnal migratory songbirds suggest that sleep occurs during migration as a homeostatic process (Borbély & Tobler Citation2011; Lesku et al. Citation2011): birds profit from stopover sites after crossing large ecological barriers to recover from sleep loss accumulated during non-stop flights (Bairlein Citation1985; Biebach et al. Citation1986; Ferretti et al. Citation2019b, Citation2020b). Indeed, birds may sleep either during the day or during the night depending on their amount of energy reserves and on their migratory strategy (Ferretti et al. Citation2019b, Citation2020b). Moreover, in migrating Whitethroats (Sylvia communis) the total amount of sleep is positively correlated with changes in energy reserves (Ferretti et al. Citation2020b), confirming an energy-saving function of sleep. However, the duration of sleep is not the only parameter of this behavior that can be regulated to manage energy expenditure. Another aspect that is important for energy saving is the posture displayed during sleep (Amlaner & Ball Citation1983). It has been observed in different species that the posture assumed during sleep is often associated with environmental conditions, in particular external temperature. The Black-billed magpie adopts a posture with the beak tucked in the scapular feathers more readily in the coldest winter nights than in warmer ones, suggesting that this posture can reduce heat loss by reducing the exposed surface (Reebs Citation1986). The use of certain positions depending on environmental factors has been studied also in mallards, where it was seen that the transition to a posture with the head turned backward is favored by climatic conditions such as rain and lower temperatures (Midtgård Citation1978). An association between the tucked posture and low ambient temperature has been found also in passerines (Wellmann & Downs Citation2009). The hypothesis of a link between posture preference and environmental temperature has been further supported by a recent study that showed that birds with particular morphology – i.e. larger bills in relation to body size – and/or living at northern latitudes have a higher probability to sleep with their head turned backwards and tucked in the scapular feathers (Pavlovic et al. Citation2019). The association between sleep posture and energy consumption has been recently confirmed also in nocturnal migratory songbirds during spring migration () (Ferretti et al. Citation2019b). Taken together, these results suggest a direct relationship between sleep posture displayed and energy expenditure. Although the amount of energy stores may affect the sleep pattern species-specifically, the strong influence of energetic status on posture preference seems to be a strategy that is shared across migratory songbirds (Ferretti et al. Citation2020b). Indeed, energy challenged individuals spend more time with their head tucked in than those that have large energy depots. Lean individuals trade-off safety for a lower energy consumption by tucking in their head under the scapular feathers. By contrast, fat individuals prefer to sleep with their head facing forward, which allows a faster reaction to potential predators (Ferretti et al. Citation2019b). The low energy consumption during tucked-in sleep is reflected also in the variation in energy reserves in both Whitethroats and Garden warblers, with tucked individual showing a lower change in energy reserve (Ferretti et al. Citation2020b). Although it is not clear whether each posture is associated with a specific sleep architecture, the reduction of metabolic rate in Garden warblers sleeping tucked in seems to be resulting from a reduced conductance achieved by increasing insulation on featherless body part, e.g. the eyes and the bill (Ferretti et al. Citation2019b). Indeed, birds have been estimated to save an amount of energy equivalent to 9 hr of fueling by simply displaying the tucked posture during the whole night (Ferretti et al. Citation2020a).
Fig. 2. Energy consumption in sleeping Garden warblers. Energy consumption decreases by almost 14% when birds tuck their head in. If we consider that Garden warblers on Ponza spend 62% of the night sleeping (i.e. 5 hr between 20:00 and 4:00; Ferretti et al. Citation2020b), this species can save approximately 0.68 kJ/night by spending the whole night tucked in. This amount of energy corresponds to 0.02 g of fat (considering the energy equivalent of fat as 37.6 kJ/g; Jenni & Jenni-Eiermann Citation1998). Redrawn from Ferretti et al. (Citation2019b)
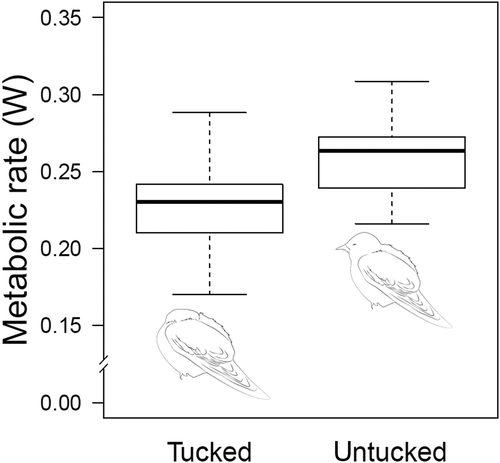
CONCLUSION
In this review, we discussed the roles of small coastal islands for migrating songbirds and examined recent studies challenging the common view that refueling is the primary goal during stopover at these sites. Indeed, several songbird species landing on these islands likely prioritize recovery from sleep loss experienced during their nocturnal migratory flight and exploit these sites for opportunistic refueling only in case of a drastic depletion of energy reserves. When the habitat does not provide sufficient resources for refueling, lean individuals try to preserve their already poor energy reserves by adopting behavioral strategies (e.g. sleeping with their head tucked into the feathers or modulating locomotor activity) aimed at optimizing energy saving as to allow relocation to more favorable refueling areas the following day. Such behavioral strategies favoring rest and sleep recovery will be facilitated by the scarcity of predators on small coastal islands. Future studies should focus on the interplay between species-specific physiological adaptations, stopover site exploitation, and habitat quality. Indeed, these aspects are determinant for understanding the ecophysiology and energetics of bird migration. This is overwhelmingly important for small islands that may represent critical migratory bottlenecks because of the direct impact that they may have on population dynamics at the breeding grounds (Dunn et al. Citation2004; Osenkowski et al. Citation2012).
AUTHOR CONTRIBUTIONS
A. Ferretti, I. Maggini and L. Fusani equally contributed to this study.
ACKNOWLEDGEMENTS
Research of our group presented in this review was made possible by the contributions of many students, personnel and volunteers in the field and laboratory. The ringing station in Ponza is operating within the long-term ringing project ‘Piccole Isole’ coordinated by Dr Fernando Spina, Istituto Superiore per la Protezione e la Ricerca Ambientale.
DISCLOSURE STATEMENT
No potential competing interest was reported by the authors.
Additional information
Funding
REFERENCES
- Aamidor SE, Bauchinger U, Mizrahy O, McWilliams SR, Pinshow B. 2011. During stopover, migrating blackcaps adjust behavior and intake of food depending on the content of protein in their diets. Integr Comp Biol. 51(3):385–393. doi:10.1093/icb/icr054
- Adamík P, Emmenegger T, Briedis M, Gustafsson L, Henshaw I, Krist M, Laaksonen T, Liechti F, Procházka P, Salewski V, Hahn S. 2016. Barrier crossing in small avian migrants: individual tracking reveals prolonged nocturnal flights into the day as a common migratory strategy. Sci Rep. 6(1):21560. doi:10.1038/srep21560
- Alerstam T. 2011. Optimal bird migration revisited. J Ornithol. 152(S1):5–23. doi:10.1007/s10336-011-0694-1
- Alerstam T, Hedenström A. 1998. The development of bird migration theory. J Avian Biol. 29(4):343–369. doi:10.2307/3677155
- Alerstam T, Lindström A. 1990. Optimal bird migration: the relative importance of time, energy, and safety. In: Gwinner E, editor. Bird migration: physiology and ecophysiology. Berlin, Heidelberg (Germany): Springer-Verlag; p. 331–351.
- Allison T, Van Twyver H. 1970. The evolution of sleep. Nat Hist. 79:56–65.
- Amlaner CJ Jr, Ball NJ. 1983. A synthesis of sleep in wild birds. Behaviour. 87(1–2):85–119. doi:10.1163/156853983X00138
- Astheimer LB, Buttemer WA, Wingfield JC. 1992. Interactions of corticosterone with feeding, activity and metabolism in passerine birds. Ornis Scand. 23(3):355–365. doi:10.2307/3676661
- Bäckman J, Andersson A, Alerstam T, Pedersen L, Sjöberg S, Thorup K, Tøttrup AP. 2016. Activity and migratory flights of individual free-flying songbirds throughout the annual cycle: method and first case study. J Avian Biol. 47:309–319. doi:10.1111/jav.01068
- Bairlein F. 1985. Body weights and fat deposition of Palaearctic passerine migrants in the central Sahara. Oecologia. 66(1):141–146. doi:10.1007/Bf00378566
- Bairlein F. 1990. Nutrition and food selection in migratory birds. In: Gwinner E, editor. Bird migration. Berlin, Heidelberg (Germany): Springer-Verlag; p. 198–213.
- Bayly NJ. 2006. Optimality in avian migratory fuelling behaviour: a study of a trans-Saharan migrant. Anim Behav. 71(1):173–182. doi:10.1016/j.anbehav.2005.04.008
- Bayly NJ. 2007. Extreme fattening by sedge warblers, Acrocephalus schoenobaenus, is not triggered by food availability alone. Anim Behav. 74(3):471–479. doi:10.1016/j.anbehav.2006.11.030
- Beauchamp G. 2004. Reduced flocking by birds on islands with relaxed predation. Proc R Soc Lond B. 271(1543):1039–1042. doi:10.1098/rspb.2004.2703
- Berger RJ. 1975. Bioenergetic functions of sleep and activity rhythms and their possible relevance to aging. In: Thorbecke GJ, editor. Biology of aging and development. Boston (MA): Springer US; p. 191–202.
- Berger RJ, Phillips NH. 1995. Energy conservation and sleep. Behav Brain Res. 69(1–2):65–73. doi:10.1016/0166-4328(95)00002-B
- Berthold P. 2003. Genetic basis and evolutionary aspects of bird migration. Adv Stud Behav. 33:175–229.
- Bibby CJ, Green RE. 1981. Autumn migration strategies of reed and sedge warblers. Ornis Scand. 12:1–12. doi:10.2307/3675898
- Biebach H, Friedrich W, Heine G. 1986. Interaction of bodymass, fat, foraging and stopover period in trans-Sahara migrating passerine birds. Oecologia. 69(3):370–379. doi:10.1007/Bf00377059
- Borbély AA, Tobler I. 2011. Manifestations and functional implications of sleep homeostasis. In: Montagna P, Chokroverty S, editors. Handbook of clinical neurology. Vol. 98. Amsterdam (Netherlands): Elsevier; p. 205–213.
- Brown JS, Kotler BP. 2004. Hazardous duty pay and the foraging cost of predation. Ecol Lett. 7(10):999–1014. doi:10.1111/j.1461-0248.2004.00661.x
- Carmi N, Pinshow B, Porter WP, Jaeger J. 1992. Water and energy limitations on flight duration in small migrating birds. Auk. 109:268–276. doi:10.2307/4088195
- Carpenter FL, Paton DC, Hixon MA. 1983. Weight gain and adjustment of feeding territory size in migrant hummingbirds. Proc Natl Acad Sci USA. 80(23):7259–7263. doi:10.1073/pnas.80.23.7259
- Cohen EB, Moore FR, Fischer RA. 2014. Fuel stores, time of spring, and movement behavior influence stopover duration of Red-eyed Vireo Vireo olivaceus. J Ornithol. 155(3):785–792. doi:10.1007/s10336-014-1067-3
- Cohen EB, Moore FR, Fischer RA, Fenton B. 2012. Experimental evidence for the interplay of exogenous and endogenous factors on the movement ecology of a migrating songbird. PLoS ONE. 7(7):e41818. doi:10.1371/journal.pone.0041818
- Cooper-Mullin C, McWilliams SR. 2016. The role of the antioxidant system during intense endurance exercise: lessons from migrating birds. J Exp Biol. 219(23):3684–3695. doi:10.1242/jeb.123992
- Delingat J, Bairlein F, Hedenström A. 2008. Obligatory barrier crossing and adaptive fuel management in migratory birds: the case of the atlantic crossing in northern wheatears (Oenanthe oenanthe). Behav Ecol Sociobiol. 62(7):1069–1078. doi:10.1007/s00265-007-0534-8
- Del Monte-Luna P, Brook BW, Zetina-Rejón MJ, Cruz-Escalona VH. 2004. The carrying capacity of ecosystems. Global Ecol Biogeogr. 13(6):485–495. doi:10.1111/j.1466-822X.2004.00131.x
- Deppe JL, Ward MP, Bolus RT, Diehl RH, Celis-Murillo A, Zenzal TJ, Moore FR, Benson TJ, Smolinsky JA, Schofield LN, et al. 2015. Fat, weather, and date affect migratory songbirds’ departure decisions, routes, and time it takes to cross the Gulf of Mexico. Proc Natl Acad Sci USA. 112(46):E6331–E6338. doi:10.1073/pnas.1503381112
- Dierschke V, Delingat J. 2001. Stopover behaviour and departure decision of northern wheatears, Oenanthe oenanthe, facing different onward non-stop flight distances. Behav Ecol Sociobiol. 50(6):535–545. doi:10.1007/s002650100397
- Dingle H. 1996. A taxonomy of movement. In: Dingle H, editor. Migration the biology of life on the move. Oxford (UK): Oxford University Press; p. 3–12.
- Dossman BC, Mitchell GW, Norris DR, Taylor PD, Guglielmo CG, Matthews SN, Rodewald PG. 2016. The effects of wind and fuel stores on stopover departure behavior across a migratory barrier. Behav Ecol. 27(2):567–574. doi:10.1093/beheco/arv189
- Drake A, Rock CA, Quinlan SP, Martin M, Green DJ, Saino N. 2014. Wind speed during migration influences the survival, timing of breeding, and productivity of a neotropical migrant, Setophaga petechia. PLoS ONE. 9(5):e97152. doi:10.1371/journal.pone.0097152
- Drent R, Piersma T. 1990. An exploration of the energetics of leap-frog migration in arctic breeding waders. In: Gwinner E, editor. Bird migration. Berlin, Heidelberg (Germany): Springer-Verlag; p. 399–412.
- Dunn E, Hussell DJT, Francis C, McCracken J. 2004. A comparison of three count methods for monitoring songbird abundance during spring migration: capture, census and estimated totals. Stud Avian Biol. 29:116–122.
- Ferretti A, Maggini I, Cardinale M, Fusani L. 2020a. Heat loss in sleeping garden warblers (Sylvia borin) during migration. J Therm Biol. 94:102772. doi:10.1016/j.jtherbio.2020.102772
- Ferretti A, Maggini I, Lupi S, Cardinale M, Fusani L. 2019a. The amount of available food affects diurnal locomotor activity in migratory songbirds during stopover. Sci Rep. 9(1):19027. doi:10.1038/s41598-019-55404-3
- Ferretti A, McWilliams SR, Rattenborg NC, Maggini I, Cardinale M, Fusani L. 2020b. Energy stores, oxidative balance, and sleep in migratory garden warblers (Sylvia borin) and whitethroats (Sylvia communis) at a spring stopover site. Integr Organ Biol. 2(1):obaa010. doi:10.1093/iob/obaa010
- Ferretti A, Rattenborg NC, Ruf T, McWilliams SR, Cardinale M, Fusani L. 2019b. Sleeping unsafely tucked in to conserve energy in a nocturnal migratory songbird. Curr Biol. 29(16):2766–2772.e2764. doi:10.1016/j.cub.2019.07.028
- Fokidis HB, Hurley L, Rogowski C, Sweazea K, Deviche P. 2011. Effects of captivity and body condition on plasma corticosterone, locomotor behavior, and plasma metabolites in curve-billed thrashers. Physiol Biochem Zool. 84(6):595–606. doi:10.1086/662068
- Fontvieille AM, Rising R, Spraul M, Larson DE, Ravussin E. 1994. Relationship between sleep stages and metabolic rate in humans. Am J Physiol Endocrinol Metab. 267(5):E732–E737. doi:10.1152/ajpendo.1994.267.5.E732
- Fransson T, Weber TP. 1997. Migratory fuelling in blackcaps (Sylvia atricapilla) under perceived risk of predation. Behav Ecol Sociobiol. 41(2):75–80. doi:10.1007/s002650050366
- Fusani L, Cardinale M, Carere C, Goymann W. 2009. Stopover decision during migration: physiological conditions predict nocturnal restlessness in wild passerines. Biol Lett. 5(3):302–305. doi:10.1098/rsbl.2008.0755
- Gómez C, Bayly NJ, Norris DR, Mackenzie SA, Rosenberg KV, Taylor PD, Hobson KA, Daniel Cadena C. 2017. Fuel loads acquired at a stopover site influence the pace of intercontinental migration in a boreal songbird. Sci Rep. 7(1):3405. doi:10.1038/s41598-017-03503-4
- Goymann W, Spina F, Ferri A, Fusani L. 2010. Body fat influences departure from stopover sites in migratory birds: evidence from whole-island telemetry. Biol Lett. 6(4):478–481. doi:10.1098/rsbl.2009.1028
- Graber JW, Graber RR. 1983. Feeding rates of warblers in spring. Condor. 85(2):139–150. doi:10.2307/1367247
- Grattarola A, Spina F, Pilastro A. 1999. Spring migration of the garden warbler (Sylvia borin) across the Mediterranean Sea. J Ornithol. 140(4):419–430. doi:10.1007/Bf01650986
- Hedenström A, Alerstam T. 1997. Optimum fuel loads in migratory birds: distinguishing between time and energy minimization. J Theor Biol. 189(3):227–234. doi:10.1006/jtbi.1997.0505
- Horton KG, Van Doren BM, Stepanian PM, Farnsworth A, Kelly JF. 2016. Seasonal differences in landbird migration strategies. Auk. 133(4):761–769. doi:10.1642/AUK-16-105.1
- Jenni L, Jenni-Eiermann S. 1998. Fuel supply and metabolic constraints in migrating birds. J Avian Biol. 29(4):521–528. doi:10.2307/3677171
- Jenni L, Schaub M. 2003. Behavioural and physiological reactions to environmental variation in bird migration: a review. In: Berthold P, editor. Avian migration. Berlin, Heidelberg, New York: Springer; p. 155–171.
- Jenni-Eiermann S, Jenni L. 1994. Plasma metabolite levels predict individual body-mass changes in a small long-distance migrant, the Garden warbler. Auk. 111(4):888–899. doi:10.2307/4088821
- Jung CM, Melanson EL, Frydendall EJ, Perreault L, Eckel RH, Wright KP. 2011. Energy expenditure during sleep, sleep deprivation and sleep following sleep deprivation in adult humans. J Physiol. 589(1):235–244. doi:10.1113/jphysiol.2010.197517
- Kelsey NA, Schmaljohann H, Bairlein F. 2019. A handy way to estimate lean body mass and fuel load from wing length: a quantitative approach using magnetic resonance data. Ringing Migr. 34(1):8–24. doi:10.1080/03078698.2019.1759909
- King JR. 1961. The bioenergetics of vernal premigratory fat deposition in the white-crowned sparrow. Condor. 63(2):128–142. doi:10.2307/1365526
- Klaassen K. 1996. Metabolic constraints on long-distance migration in birds. J Exp Biol. 199(Pt 1):57–64. Available from: http://jeb.biologists.org/content/199/1/57
- Lesku JA, Vyssotski AL, Martinez-Gonzalez D, Wilzeck C, Rattenborg NC. 2011. Local sleep homeostasis in the avian brain: convergence of sleep function in mammals and birds? Proc R Soc Lond B. 278(1717):2419–2428. doi:10.1098/rspb.2010.2316
- Lima SL. 1986. Predation risk and unpredictable feeding conditions: determinants of body mass in birds. Ecology. 67(2):377–385. doi:10.2307/1938580
- Lima SL. 1988. Vigilance during the initiation of daily feeding in dark-eyed juncos. Oikos. 53(1):12–16. doi:10.2307/3565657
- Lima SL, Dill LM. 1990. Behavioral decisions made under the risk of predation: a review and prospectus. Can J Zool. 68(4):619–640. doi:10.1139/z90-092
- Lind J, Cresswell W. 2006. Anti-predation behaviour during bird migration; the benefit of studying multiple behavioural dimensions. J Ornithol. 147(2):310–316. doi:10.1007/s10336-005-0051-3
- Lindström Å, Alerstam T. 1992. Optimal fat loads in migrating birds: a test of the time-minimization hypothesis. Am Nat. 140(3):477–491. doi:10.1086/285422
- Maggini I, Cardinale M, Sundberg JH, Spina F, Fusani L, Ambrosini R. 2020. Recent phenological shifts of Migratory birds at a Mediterranean spring stopover site: Species wintering in the Sahel advance passage more than tropical winterers. PLoS ONE. 15(9):e0239489. doi:10.1371/journal.pone.0239489
- Markwald RR, Melanson EL, Smith MR, Higgins J, Perreault L, Eckel RH, Wright KP Jr. 2013. Impact of insufficient sleep on total daily energy expenditure, food intake, and weight gain. Proc Natl Acad Sci USA. 110(14):5695–5700. doi:10.1073/pnas.1216951110
- McCabe JD, Olsen BJ. 2015. Tradeoffs between predation risk and fruit resources shape habitat use of landbirds during autumn migration. Auk. 132(4):903–913. doi:10.1642/Auk-14-213.1
- McWilliams SR, Guglielmo C, Pierce B, Klaassen M. 2004. Flying, fasting, and feeding in birds during migration: a nutritional and physiological ecology perspective. J Avian Biol. 35(5):377–393. doi:10.1111/j.0908-8857.2004.03378.x
- Meissner W. 1998. Fat reserves in Dunlins Calidris alpina during autumn migration through Gulf of Gdańsk. Ornis Svecica. 8:91–102.
- Metcalfe NB, Furness RW. 1984. Changing priorities: the effect of pre-migratory fattening on the trade-off between foraging and vigilance. Behav Ecol Sociobiol. 15(3):203–206. doi:10.1007/Bf00292976
- Midtgård U. 1978. Resting postures of the Mallard Anas platyrhynchos. Ornis Scand. 9(2):214–219. doi:10.2307/3675884
- Moore FR, Kerlinger P, Simons TR. 1990. Stopover on a gulf coast barrier island by spring trans-gulf migrants. Wilson Bull. 102:487–500. http://www.jstor.org/stable/4162904
- Moore FR, Yong W. 1991. Evidence of food-based competition among passerine migrants during stopover. Behav Ecol Sociobiol. 28(2):85–90. doi:10.1007/BF00180984
- Morton ML. 1967. Diurnal feeding patterns in white-crowned sparrows, Zonotrichia leucophrys gambelii. Condor. 69(5):491–512. doi:10.2307/1366149
- Nilsson C, Klaassen RH, Alerstam T. 2013. Differences in speed and duration of bird migration between spring and autumn. Am Nat. 181(6):837–845. doi:10.1086/670335
- Osenkowski JE, Paton PW, Kraus D. 2012. Using long-term constant-effort banding data to monitor population trends of migratory birds: a 33-year assessment of adjacent coastal stations. Condor. 114:470–481. doi:10.1525/cond.2012.110169
- Pavlovic G, Weston MA, Symonds MRE, Portugal S. 2019. Morphology and geography predict the use of heat conservation behaviours across birds. Funct Ecol. 33(2):286–296. doi:10.1111/1365-2435.13233
- Pilastro A, Spina F. 1997. Ecological and morphological correlates of residual fat reserves in passerine migrants at their spring arrival in southern Europe. J Avian Biol. 28(4):309–318. doi:10.2307/3676944
- Pomeroy AC, Butler RW, Ydenberg RC. 2006. Experimental evidence that migrants adjust usage at a stopover site to trade off food and danger. Behav Ecol. 17(6):1041–1045. doi:10.1093/beheco/arl043
- Rattenborg NC. 2006. Do birds sleep in flight? Naturwissenschaften. 93(9):413–425. doi:10.1007/s00114-006-0120-3
- Rattenborg NC, Mandt BH, Obermeyer WH, Winsauer PJ, Huber R, Wikelski M, Benca RM, G. M. Morris R. 2004. Migratory sleeplessness in the white-crowned sparrow (Zonotrichia leucophrys gambelii). PLoS Biol. 2(7):e212. doi:10.1371/journal.pbio.0020212
- Rattenborg NC, Martinez-Gonzalez D, Lesku JA. 2009. Avian sleep homeostasis: convergent evolution of complex brains, cognition and sleep functions in mammals and birds. Neurosci Biobehav Rev. 33(3):253–270. doi:10.1016/j.neubiorev.2008.08.010
- Reebs SG. 1986. Sleeping behavior of black-billed magpies under a wide range of temperatures. Condor. 88:524–526. doi:10.2307/1368284
- Schaub M, Jenni L. 2001. Variation of fuelling rates among sites, days and individuals in migrating passerine birds. Funct Ecol. 15(5):584–594. doi:10.1046/j.0269-8463.2001.00568.x
- Schmaljohann H. 2018. Proximate mechanisms affecting seasonal differences in migration speed of avian species. Sci Rep. 8(1):4106. doi:10.1038/s41598-018-22421-7
- Schmaljohann H, Both C. 2017. The limits of modifying migration speed to adjust to climate change. Nature Clim Change. 7(8):573–576. doi:10.1038/nclimate3336
- Schmaljohann H, Dierschke V. 2005. Optimal bird migration and predation risk: a field experiment with northern wheatears Oenanthe oenanthe. J Anim Ecol. 74(1):131–138. doi:10.1111/j.1365-2656.2004.00905.x
- Schmaljohann H, Eikenaar C. 2017. How do energy stores and changes in these affect departure decisions by migratory birds? A critical view on stopover ecology studies and some future perspectives. J Comp Physiol A. 203:411–429. doi:10.1007/s00359-017-1166-8
- Schmaljohann H, Fox JW, Bairlein F. 2012. Phenotypic response to environmental cues, orientation and migration costs in songbirds flying halfway around the world. Anim Behav. 84(3):623–640. doi:10.1016/j.anbehav.2012.06.018
- Schmaljohann H, Korner-Nievergelt F, Naef-Daenzer B, Nagel R, Maggini I, Bulte M, Bairlein F. 2013. Stopover optimization in a long-distance migrant: the role of fuel load and nocturnal take-off time in Alaskan northern wheatears (Oenanthe oenanthe). Front Zool. 10(1):26. doi:10.1186/1742-9994-10-26
- Schmaljohann H, Liechti F, Bruderer B. 2007. Songbird migration across the Sahara: the non-stop hypothesis rejected! Proc R Soc Lond B. 274(735–739). doi:10.1098/rspb.2006.0011
- Schofield LN, Deppe JL, Zenzal TJ, Ward MP, Diehl RH, Bolus RT, Moore FR. 2018. Using automated radio telemetry to quantify activity patterns of songbirds during stopover. Auk. 135(4):949–963. doi:10.1642/Auk-17-229.1
- Schwilch R, Piersma T, Holmgren NMA, Jenni L. 2002. Do migratory birds need a nap after a long non-stop flight? Ardea. 90:149–154. https://www.rug.nl/research/portal/files/3067222/2002ArdeaSchwilch.pdf
- Sih A. 1980. Optimal behavior: can foragers balance two conflicting demands? Science. 210(4473):1041–1043. doi:10.1126/science.210.4473.1041
- Sirot E. 2006. Social information, antipredatory vigilance and flight in bird flocks. Anim Behav. 72(2):373–382. doi:10.1016/j.anbehav.2005.10.028
- Smith RJ, Moore FR. 2005. Arrival timing and seasonal reproductive performance in a long-distance migratory landbird. Behav Ecol Sociobiol. 57(3):231–239. doi:10.1007/s00265-004-0855-9
- Spina F, Massi A, Montemaggiori A, Baccetti N. 1993. Spring migration across central Mediterranean: general results from the “Progetto Piccole Isole”. Vogelwarte. 37:1–94.
- Stahel CD, Megirian D, Nicol SC. 1984. Sleep and metabolic rate in the little penguin, Eudyptula minor. J Comp Physiol B. 154(5):487–494. doi:10.1007/BF02515153
- Toates FM. 1980. Animal behaviour – a systems approach. Chichester (UK): Wiley.
- Weber TP, Ens BJ, Houston AI. 1998. Optimal avian migration: a dynamic model of fuel stores and site use. Evol Ecol. 12(4):377–401. doi:10.1023/A:1006560420310
- Wellmann AE, Downs CT. 2009. A behavioural study of sleep patterns in the malachite sunbird, Cape white-eye and fan-tailed widowbird. Anim Behav. 77(1):61–66. doi:10.1016/j.anbehav.2008.09.010
- Wikelski M, Spinney L, Schelsky W, Scheuerlein A, Gwinner E. 2003. Slow pace of life in tropical sedentary birds: a common-garden experiment on four stonechat populations from different latitudes. Proc R Soc Lond B. 270(1531):2383–2388. doi:10.1098/rspb.2003.2500
- Woodworth BK, Mitchell GW, Norris DR, Francis CM, Taylor PD. 2015. Patterns and correlates of songbird movements at an ecological barrier during autumn migration assessed using landscape- and regional-scale automated radiotelemetry. Ibis. 157(2):326–339. doi:10.1111/ibi.12228