Abstract
This paper demonstrates a methodology developed through applying and refining Lake Simcoe Region Conservation Authority (LSRCA) and Bradford’s (2011) framework for setting ecological flow and water level targets. Each step of the methodology is described along with an example application within Lovers Creek subwatershed. The methodology requires subwatershed objectives and habitat specialists to be defined. Study nodes are selected and the reference streamflow regime is characterized along with the hydrological alteration that has occurred, or is expected to occur under a given scenario. Potential ecological responses to the various hydrologic alterations are then identified. Methods for setting overall ecosystem health and specific ecological objective flow targets are discussed and demonstrated. The targets are then integrated into a flow regime for each study node and a process for using this information for decision-making is suggested. The targets developed using the methodology presented in this paper are mainly limited by the accuracy of the hydrologic model and the quantified flow magnitudes. Recommendations for improving these components of the assessment are made. The unique approach presented in this paper provides explicit steps for developing flow targets for subwatersheds within Southern Ontario and beyond. This research contributes toward the advancement of ecological flow assessment within Southern Ontario, which provides opportunities for enhanced protection and restoration of ecosystem health across the Province.
Cet article démontre une méthodologie développée en appliquant et raffinant la procédure de l’Office de protection de la nature de la région du lac Simcoe (OPNRLS) et de Bradford (2011) pour l’établissement de débit écologiques et d’objectifs de niveau d’eau. Chaque étape de la méthodologie est décrite avec un exemple d’application de méthodologie à travers l’OPNRLS et le sous bassin hydrographique du ruisseau Lovers. La méthodologie nécessite la définition des objectifs pour les sous bassins hydrographiques avec l’aide de spécialistes de l’habitat aquatique. Des points d’étude sont ensuite choisis et le régime hydraulique naturel est caractérisé en tenant compte des altérations hydrologiques qui ont eu lieu à chaque point. Des hypothèses sur les réactions écologiques possibles dues aux altérations hydrologiques sont alors émises pour les différents types de changements observés à chaque site. Des méthodes pour définir la santé globale de l’écosystème et pour maintenir des objectifs de débit écologique spécifique sont discutées et mises en œuvre. Ces objectifs sont ensuite intégrés dans un régime d’écoulement pour chaque point d’étude et un protocole d’utilisation de cette information pour la prise de décision est proposé. Les objectifs développés en utilisant la méthode présentée dans cet article sont principalement limités par la précision du modèle hydrologique et la magnitude des mesures de débits. Des recommandations sont ensuite formulées pour améliorer les composantes de l’évaluation. L’approche unique et les recommandations présentées dans ce document constituent des mesures explicites pour la fixation d’objectifs de débit pour les sous bassins hydrographiques du sud de l’Ontario et de l’OPNRLS. Cette recherche contribue à l’avancement des connaissances en matière d’évaluation des débits écologiques dans le sud de l’Ontario, et offre des possibilités d’amélioration de la protection et de la restauration de la santé des écosystèmes à travers la province.
Introduction
It is widely recognized that maintenance of a semblance of a river’s natural flow regime is required to sustain its natural structure and function (Poff et al. Citation1997; Bradford Citation2008). Naturally-functioning rivers are extremely important as they maintain healthy aquatic environments and promote human wellbeing through the provision of ecosystem services (Acreman and Ferguson Citation2010).
In 2008, the Government of Ontario passed The Lake Simcoe Protection Act, which was developed with the intent of protecting and restoring the health of the Lake Simcoe Watershed. The Lake Simcoe Protection Plan (LSPP) was subsequently developed to provide objectives and priorities for restoration and protection. Chapter 5 of the LSPP discusses water quantity objectives within the watershed, specifically referring to the preservation of water supply to protect aquatic ecosystems through the maintenance of ecological flow regimes (LSRCA Citation2009). Ecological flow assessment has relevance within a variety of provincial program areas in Ontario, such as Species at Risk, Source Water Protection, Ontario Low Water Response and the Permit to Take Water Program (OMNR Citation2010; AquaResource Inc. Citation2011a; Conservation Ontario, n.d).
Towards a framework for determining ecological flows and water levels in the Lake Simcoe Watershed – A guidance document (LSRCA and Bradford Citation2011) was developed to provide a framework for moving flow assessment in Ontario beyond a single (typically minimum) flow statistic to more comprehensive target regimes that capture hydrologic variability important to sustaining aquatic ecosystems (Poff et al. Citation1997). A pilot Environmental Flow Assessment (EFA) for Lovers Creek subwatershed in the Barrie/Simcoe area of the Lake Simcoe Watershed was undertaken using the framework outlined in the guidance document and the conceptual model of the Functional Unit Method (Al Zaghal Citation2010). Through implementation, the framework was refined and a methodology was developed and documented. This paper provides an overview of the EFA methodology, its applications to Lovers Creek and recommendations for future application. The framework was applied to develop both streamflow and water level targets (Beaton Citation2012), but this paper focuses on the streamflow regime.
Methodology overview
There are three phases of the refined framework (Table ). The Context Setting Phase comprises five steps that serve the purpose of developing the foundational knowledge required for the flow assessment. This phase also includes the selection of analysis nodes. Most of the information required to complete these steps was available within existing documentation such as the LSRCA subwatershed plans. Expert scientific input was used to confirm and fill gaps in the available information.
Table 1. Steps of framework for ecological flows and water levels.
The Development of a Reference Regime and Hydro-ecological Analysis Phase consists of three steps that must be completed for each analysis node. The main purpose of this phase is to establish an understanding of the historic and current hydrologic regime at each node and assess the potential ecological responses to the calculated hydrologic alteration.
The final phase in the framework is the Development of the Target Regime which also consists of three steps. The purpose of Phase III is to generate a target regime that will be used to restore or protect general ecosystem health as well as specific ecological functions. This phase is also completed at each study node. The targets for overall ecosystem health are developed solely from the hydrologic statistics while targets for ecological objectives should be developed from hydrologic statistics and quantified functional flows. The targets for overall ecosystem health and the targets for the ecological objectives are then combined to create a target regime for each analysis node.
Phase I: Context setting
Define subwatershed characteristics
Information for the definition of subwatershed characteristics can be derived from existing documentation and scientific experts with local knowledge. Data and information on topography, land cover, geology, geomorphology, climate, hydrology, hydrogeology and ecology should be gathered and synthesized in this step. In addition, relevant models that have been developed for the study area should be acquired at this stage. This EFA methodology relies heavily on simulated hydrologic model output to develop target regimes. The Province of Ontario has developed sophisticated groundwater, surface water and integrated numerical models within selected conservation area subwatersheds that can be used for the development of target regimes (AquaResource Inc. Citation2011a). The methodology presented in this paper leverages the significant investment that has been made by the province to date by incorporating these numerical models in the process to develop ecological flow regimes.
Lovers Creek subwatershed is 59.5 km², accounting for approximately 2% of Lake Simcoe Watershed’s total area. The subwatershed has a gentle gradient with 70% of the area having slopes less than 5%. Steeper slopes (> 5%) are mostly located in the west central area of the subwatershed (Figure ). Subwatershed land cover consists of urban areas (24%), agriculture (46%), marsh and swamp (16%) and forest (14%) with the majority of urban areas occurring in the north end of the subwatershed near the outlet into Lake Simcoe (Figure ). The creek runs through till plain with some surficial deposits of kame or sand and gravel outwash in the areas of higher elevation which are mostly in the western section of the subwatershed. Significant groundwater upwelling, coldwater habitat and trout spawning occur along some of the western tributaries (LSRCA Citation2010, Citation2011a, Citation2011b).
Figure 2 Land cover within the contributing area of each analysis node. LOV, Lovers Creek sites; CWT, Central Western Tributary site.
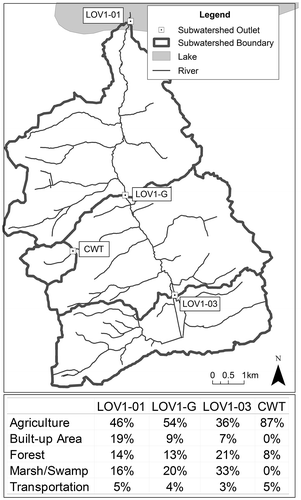
Lovers Creek Swamp is a Provincially Significant Wetland located within the subwatershed that consists of 99% swamp and 1% marsh. The wetland primarily runs along the main channel of the stream and covers an area of 6.8 km² (LSRCA Citation2010). The swamp holds a number of locally significant plant species as well as locally significant winter cover, staging and moulting habitat for waterfowl and fish staging, migration, spawning and nursery habitat (OMNR Citation1994, Citation2011).
A MIKE-SHE (DHI Citation2007) integrated hydrologic model was developed by AquaResource Inc. for the Barrie area (Figure ). The model simulates infiltration using a two-layer water balance with the Green and Ampt infiltration routine, and saturated flow is modelled using an iterative, implicit finite difference solution of the three-dimensional Darcy equation. The model has a 200-m grid resolution and has been calibrated to the Tollendale gauge at the mouth of Lovers Creek (AquaResource Inc. and Golder Associates Citation2010; AquaResource Inc. Citation2011b; LSRCA Citation2011b). The model was developed primarily for the purpose of evaluating risk to drinking water and it is acknowledged that advances are needed for application to ecological flow assessment. Nevertheless, the model was adequate to provide the streamflow and water level time series necessary to demonstrate the methodology which was the focus of the study.
When data and resources are not available to complete a full scientific assessment, it is common practice within EFA and other disciplines to use expert opinion to fill gaps (Cottingham et al. Citation2001). For the Lovers Creek EFA, the Delphi technique was used for obtaining expert input (Crance Citation1987; Pill Citation1971) to complete steps b–d of the Context Setting Phase. As recommended by Acreman and Dunbar (Citation2004), the expert scientific team chosen should consist of multidisciplinary experts with a good knowledge of the study area. The expert panel for the Lovers Creek EFA included a Senior Environmental Monitoring Scientist, Senior Fisheries Biologist, Natural Heritage Ecologist, Aquatic Ecologist and a Conservation Lands Planner from the LSRCA as well as a Fish and Wildlife Technician from the Midhurst District Ministry of Natural Resources.
The experts were asked a series of questions. Answers that occurred more frequently and answers that respondents explicitly identified as important were given greater weight. The aggregated survey responses as well as a summary of the responses and a description of how the results were going to be used were sent to the participants. The participants had an opportunity to respond, and these responses were considered with respect to the original survey and the conclusions were adjusted accordingly.
Identify goals and objectives
The general subwatershed goals and more specific objectives for the subwatershed related to the aquatic ecosystem should be determined and documented. Although a range of goals and objectives were identified for Lovers Creek, the majority of the responses were related to coldwater fish, wetland protection and maintenance of natural geomorphic functions (Table ). The framework and methodology is adaptive and iterative. If new knowledge suggests that additional functions should be integrated into the flow assessment, then this can be done when the assessment is revisited (Al Zaghal Citation2010; LSRCA and Bradford 2011).
Table 2. Translation of general goals to limiting needs (adapted from Al Zaghal 2010).
Identify habitat specialists
Habitat specialists that rely on components of the flow or water level regime to carry out specific phases of their life history should be identified and documented. Again, this information can be derived from existing literature or from experts with knowledge of the study area. The main habitat specialists identified in Lovers Creek subwatershed include coldwater fish (primarily brook trout but also mottled sculpin) as well as wetland amphibians and wetland vegetation (Table ).
Complete functional flow assessment
The functional flow assessment method based on the Functional Unit Method (Al Zaghal Citation2010) should be completed next. The Functional Unit Method (FUM) is a methodology that identified ecological functions with objectives related to fluvial geomorphology, and with potential to be used for other ecological functions (Al Zaghal Citation2010). It follows a process-based approach that is conceptually built on ecological functions, variables that limit functions, and processes governing these variables. The Flow regime provides temporal aspects to these processes. The purpose of functional assessment is to refine the scope of the study to focus on a set of key species, functions, hydrologic processes and variables that are deemed to be the most important to the system. This step leads to a realistic set of variables that can be analyzed with available resources. Additional species, functions and variables can be added in subsequent iterations of the flow assessment if necessary.
The functional flow assessment involves identifying required functions for each goal and the variables that limit those functions. The flow and non-flow related processes that govern these variables should be summarized along with the temporal aspects of the flow regime. Tables show how the functional flow assessment was compiled for Lovers Creek.
Table 3. Functional flow assessment for Lovers Creek streamflow points.
Geomorphic/hydrologic classification and selection of study nodes
Identifying study nodes is an important step in the EFA process. Study nodes are points within the subwatershed where analysis will be conducted and locations where flow regimes will be developed. Priority study node locations are areas where important ecological values exist, or did exist, and locations that have been or will be affected by land and water management decisions. It was anticipated that specific locations of ecological value, such as locations of brook trout spawning sites, were going to be identified within Lovers Creek. Instead, more general locations were identified including headwater areas, all wetlands (particularly headwater wetlands), gaining reaches and lower western tributaries.
Study nodes should capture the geomorphic and hydrologic variability of the landscape. In order to do this, a hydrologic and/or geomorphic classification of the landscape should be completed. Once the classification is completed, study nodes should be distributed across the different classes. It may then be possible to transfer flow assessment information between sites that have the same classification, reducing resources required to complete the assessment at numerous locations.
A geomorphic classification, based on slope and surficial geology, existed for Lovers Creek, and data such as stream temperature, fish and benthic invertebrate presence had been collected by the LSRCA at monitoring sites determined by the geomorphic classification. The methodology was tested for a subset of the LSRCA monitoring sites, shown as subwatershed outlets in Figure . Three of the points run along the main channel of Lovers Creek from the mouth up to the headwaters, and were chosen to gain an understanding of the difference in hydrology at different locations along the main channel. The fourth point is located at the upstream end of one of the western tributaries. This location is not an LSRCA monitoring station but was chosen as it is within an Area of Natural and Scientific Interest.
Phase II: Development of a reference regime and hydro-ecological analysis
The purpose of this phase is to establish a reference regime, calculate the hydrologic alteration and determine the potential ecological response to the hydrologic alteration at each study node. The reference regime is developed by conducting statistical analysis on the synthetic streamflow data produced from a hydrologic model of natural (or reference) conditions. The hydrologic alteration is then calculated by comparing the reference regime to the modelled current (or future) regime using software such as the Indicators of Hydrologic Alteration (IHA). Finally, the potential ecological responses to the calculated hydrologic alterations are identified using generalized relationships, expert input or results from site-specific studies (The Nature Conservancy Citation2009).
For Lovers Creek, MIKE-SHE was used to simulate daily streamflows for the natural and current conditions with the same 59 (1951–2009) meteorological inputs so human impact on the flow regime could be separated from climatic influences. In this study, the reference condition was taken to be the “pre-settlement” hydrologic condition. The condition before the majority of European settlement took place in the study area was created by removing urban and agricultural land cover from the model. Hard-copy land surveys from the early 1800s were obtained from the Natural Heritage Information Centre of the Ministry of Natural Resources to determine the historic extent of wetland and forest. IHA was then used to characterize the natural regime and to calculate hydrologic alteration at each study node (The Nature Conservancy Citation2009).
Characterize the natural regime
A variety of statistics were calculated to characterize the natural regime at each study node (Beaton Citation2012). A brief summary of the characterization is provided below. As would be expected, flow magnitudes are smaller for the sites further upstream along the main channel (Table ). In contrast to the sites along the main channel, the Central Western Tributary (CWT) is an ephemeral stream with a flow of 0 m3/s for over 92% of the time and a median value of 340 zero flow days. This model output is consistent with field observations at the CWT site which revealed little evidence of an active channel.
Table 4. Basic streamflow statistics for all streamflow sites. LOV, Lovers Creek sites. CWT, Central Western Tributary.
The sites along the main channel have an annual flow pattern that is typical of permanent streams in Southern Ontario with high spring flows, low summer baseflows and a small fall freshet. The Coefficient of Dispersion (CD) is calculated in IHA and is useful for describing the variability of the hydrologic statistics (The Nature Conservancy 2009):(1)
The CD (inter-annual variability) for median monthly flow is the highest in the spring for sites LOV1-01 and LOV1-G and lowest in the summer whereas the opposite is true for site LOV1-03 (Figure ). The higher inter-annual variability of summer flows at LOV1-03 may be a result of less reliable baseflows from the smaller contributing area at this site versus the other two sites further downstream. The higher inter-annual variability of the spring flows at sites LOV1-01 and LOV1G may be a result of the variability in delivery of the spring melt and precipitation caused by heterogeneous landscape characteristics, (such as slope, surficial geology and land cover) precipitation and snowpack across the larger contributing area of these sites (Maidment Citation1993).
Assessment of hydrologic alteration
It is important to understand the major disturbances within the catchment area of each study node (conversion of forest to urban and agricultural land uses) in order to qualitatively assess the hydrologic model output. The expected effects on the system’s hydrology include reduced evapotranspiration and storage, from the loss of forest vegetation, and reduced infiltration, associated with impervious surfaces. These changes generally result in increased high and flood flow magnitudes and frequencies, decreased high and flood flow durations and increased rise and fall rates associated with decreased durations (Leopold Citation1968). Changes to baseflow are expected to vary depending upon the relative changes to evapotranspiration and infiltration. The current land cover within the contributing area of each site can be seen in Figure . The majority of urbanization is located near the mouth of the subwatershed and is within the contributing area of LOV1-01 but not LOV1-G, LOV1-03 or the CWT site.
Pre-settlement and current modelled streamflows were compared using IHA to determine the degree of hydrologic alteration at all of the streamflow study nodes. As with the characterization of the natural regime, a variety of statistics were calculated to characterize the hydrologic alteration at each study site (Beaton Citation2012), but only a brief summary is provided here.
The flow duration curve (FDC) in Figure indicates that flows of all exceedance probabilities at site LOV1-G have increased, with median flow (50th percentile) increasing by 19% from pre-settlement to the current regime. The FDCs for all other sites are very similar, indicating that both the low and high flows increase at all of the sites from the pre-settlement to the current condition. These observations are consistent with the expected effects of replacing forests with urban and agricultural land. The increased baseflow may be attributed to the reduced evapotranspiration having a larger effect than the decreased infiltration associated with increased impervious surfaces.
Hypothesized ecological responses to hydrologic alteration
The purpose of this step is to hypothesize potential ecological responses to the identified hydrologic alterations. First, the ecological characteristics of each site should be described. Next, the ecological functions from the functional flow assessment that are relevant at each site should be identified based on the characteristics of each site. Following Mathews and Richter (Citation2007), an ecological model should be developed for each site based on the identified ecological functions and their temporal aspects. Finally, potential ecological responses to hydrological alteration should be discussed based on the type of hydrological alteration and the ecological characteristics of each site. The development of an ecological model for site LOV1-01 will be discussed to demonstrate the process.
It is important to note that the ecological functions that were chosen for each site in this study were based on the current condition of the subwatershed and were thus chosen to protect existing conditions. If the objective of the flow assessment is to restore sites to a given condition then the attributes of the restored condition should be used to define the ecological model.
Ecological characteristics of site LOV1-01
Over the past decade, ecological data have been collected at site LOV1-01 by the LSRCA. Using the classification system developed by Stoneman and Jones (Citation1996), the site has warm water temperatures, but coldwater fish (both brook trout and mottled sculpin) have been caught at this site. The occurrence of coldwater fish in warm water habitats suggests the presence of coldwater microhabitats such as areas with deep pools, groundwater upwelling, undercut banks and riparian or instream cover. The site has a fair index of biotic integrity, meaning that there is low to average diversity and abundance of fish (LSRCA Citation2011a). Based on a survey of the banks along a 40-m transect, there is potential for stream bank erosion.
An ecological model was developed based on information from the functional flow assessment and the site attributes outlined above (Figure ). The ecological functions identified through the functional flow assessment included maintaining thermal refuge pools in the summer, maintaining spawning habitat and maintaining connectivity to thermal refuge areas and spawning habitat. The functions of maintaining summer thermal refuge pools as well as connectivity to summer pools are particularly important at site LOV1-01 because warm water temperatures are recorded but coldwater fish are observed at this site. Connectivity to spawning habitat will also be important since brook trout are present at this site and will need to migrate upstream to spawning habitat. The function of maintaining spawning habitat for coldwater fish is not relevant at this site since, according to the scientific experts, spawning occurs in the headwaters.
Flow-ecological response linkages
The next step is to identify the potential ecological responses to hydrological alterations. Response hypotheses can be based on scientifically derived, general relationships that have been compiled from decades of environmental flow studies, or they can be based on site-specific knowledge derived through local expert input or scientific monitoring studies (Poff et al. Citation1997). The ecological responses explored should not be limited by the important ecological functions that have been identified in Phase 1 of the EFA, but should explore a wide range of potential responses to the alteration. The hypothesized responses occur at different temporal scales and may have already occurred within the system, may be in the process of occurring or may occur in the future as a result of the hydrological alteration. It is important to be able to communicate the ecological implications of hydrologic alterations to decision-makers. As an example, a change in annual high flow frequency at site LOV1-01 was identified. The potential implications of such a change could include:
Increased erosion which may disrupt physical habitat features such as pools and riffles. This may then have an effect on the summer pool habitat available for thermal refuge at site LOV1-01 (The Nature Conservancy Citation2009).
Change in the size of streambed substrates which has subsequent effects on the use of the space by fish and benthics that prefer a given substrate type for certain phases of their life cycle (Poff et al. Citation2010).
The nutrient balance also may be affected as there may be increased flushing of nutrients downstream (Withers and Jarvie Citation2008).
Temperature spikes may occur as a result of the surface runoff coming in contact with hot pavement in the summer before entering the stream. These temperature spikes may cause stress or mortality to coldwater species in the area (Jones and Hunt Citation2009).
This step helps to identify more critical characteristics of the natural flow regime for a particular location. In circumstances where there is conflict between important ecological values and water users, more detailed work is often needed to quantify ecological responses to various hydrologic alterations. The hypothesized flow-ecological response relationships can be used to identify monitoring opportunities and guide scientific studies that test the hypotheses. A sound understanding of the relationships is the basis for the development of a target regime that can be used to guide development and restoration activities within the subwatershed.
Phase III: Development of a target regime
Two types of hydrological targets are set in this flow assessment methodology. The first set of targets is important for overall ecosystem health and help to maintain functions that are not specifically addressed in the second set of hydrological targets. The second set of hydrological targets relates to the specific ecological objectives outlined in the functional flow assessment and is intended to maintain those specific important functions.
Targets for overall ecosystem health
To maintain a broad range of ecological functions for overall ecosystem health, a wide range of hydrologic statistics should be maintained as close to their natural range of variability as possible. The IHA program calculates 33 ecologically relevant hydrologic statistics that describe different components of the hydrologic regime. Within IHA, annual or seasonal summary values (e.g. median) of each of the statistics are divided into categories based on the percentile of the value within long-term data sets. Three range of variability (RVA) categories are created: Low = 0th–33rd percentile, Mid = 33rd–66th percentile and High = 66th–100th percentile. Under the natural condition, an equal number of values falls into each category. The hydrologic alteration (HA) factor calculated in IHA is a measure of the change in the number of values that fall into each category from the pre-settlement to the altered condition (Figure ). The overall ecosystem health target is to maintain an equal distribution of the annual values across the three categories (The Nature Conservancy Citation2009).
Targets based on ecological objectives
The purpose of the ecological objective targets is to protect the required functions identified in the Context Setting Phase. This is accomplished by setting specific magnitude, frequency and duration targets for each important function. Flow magnitudes required for each function should be determined using quantitative methods such as hydraulic or habitat modelling. If data are not available to quantify the functional flow magnitudes, they can be estimated through expert input or can be set using hydrological threshold approximations.
For Lovers Creek, quantification of flow magnitudes for selected functions was attempted using the assessment tools and methods shown in Table . However, available data proved to be insufficient for this application, and magnitudes were ultimately estimated using hydrologic threshold approximations.
Table 5. Table relating flow objectives to information required for quantification of flows.
In addition to the 33 ecologically relevant statistics calculated within IHA described above, another group of statistics called Environmental Flow Components (EFCs) is available. There are five types of EFCs: extreme low flows, low flows, high flows, small floods and large floods (Figure ). Flow magnitude thresholds based on user defined discharge values, flow duration curve percentiles and/or return intervals are set to assign the bounds of the five EFC types. The frequency, duration, peak flow, timing and rise and fall rates are calculated on an annual or user-defined seasonal basis for each EFC (The Nature Conservancy Citation2009).
Figure 7 An example of Environmental Flow Component (EFC) flow ranges. The horizontal lines on the hydrograph represent thresholds that separate each EFC.
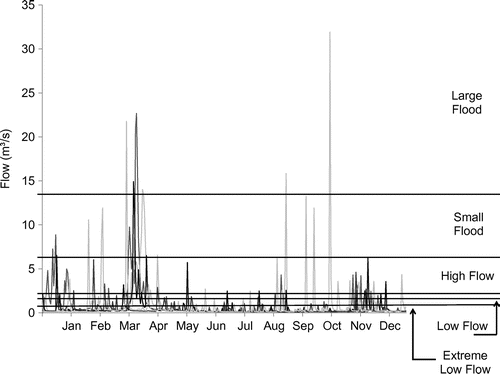
Targets were developed for the ecological functions that were identified as being important for each site within Lovers Creek according to the ecological models developed. Ecological functions were associated with an appropriate EFC according to the general functional associations of the EFCs presented in The Nature Conservancy (Citation2009). For example, The Nature Conservancy (Citation2009) associates the high flow EFC with the function of shaping the physical character of the river including the formation and maintenance of pools and riffles. So, for target setting in Lovers Creek, the high flow EFC was associated with the desired function of scouring pools to maintain pool depth for summer refugia. The association of EFC parameters to the ecological functions for site LOV1-01 can be seen in Table .
Flow targets can be categorized into one of four possible target types (Figure ). Identified functions are categorized as having either a minimum magnitude threshold or a maximum magnitude threshold. They are also categorized based on whether increasing above or decreasing below a flow threshold is desired or not desired. A Type 1 threshold is applicable to functions where it is hypothesized that a decrease in flow magnitude below a minimum threshold will have a negative impact on the ecological function. For example, maintaining summer pool depth is a minimum threshold because decreasing depth (by decreasing flow) below the minimum threshold would negatively impact the function. A Type II threshold is applicable where an increase in flow magnitude above a maximum threshold may have a negative impact on the function. Flows to scour pools have a Type III threshold whereby flows above a minimum flow threshold are desired periodically to achieve the function. However, this example highlights the importance of frequency and duration targets; flows should be below the threshold most of the time to prevent changes to channel morphology.
Figure 8 The four types of ecological targets based on the direction of flow magnitude change and whether crossing the flow threshold is desired or not desired.
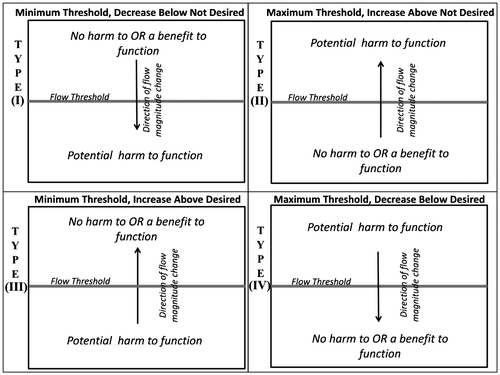
Frequency and duration targets should be set for each of the identified ecological functions at each site. For Lovers Creek, the frequency and duration targets are based on the natural frequency and duration of flows within the range of magnitudes defined by the EFC thresholds. The targets are defined by the 25th or 75th percentile of the natural range of the frequency and duration of flows. For targets where increasing above or decreasing below the threshold is desired, the 25th percentile of the median seasonal or annual frequency and duration was set as a minimum target. For functions where increasing above or decreasing below the threshold is not desired, the 75th percentile was set as a maximum target. These targets can be refined if additional research indicates that different frequencies and durations are suitable for performing the given functions. The targets for site LOV1-01 can be seen in Table . This table indicates that flows between 0.15 m3/s and 0.08 m3/s should not have a median occurrence of more than 11 times per summer, and should not have a median duration of more than 12 days or there may be negative impacts to the functions of maintaining pools for summer refugia and connectivity to thermal refuge areas at site LOV1-01.
Table 6. Flow targets for ecological objectives. EFC, Environmental Flow Component.
This EFA methodology is viewed as an ongoing process that should be iteratively reassessed and refined to produce greater confidence in the targets. When more refined flow magnitudes are calculated or decided through expert input then updated values can easily be integrated into an improved flow assessment using the techniques demonstrated in this paper.
Integration into a target regime
The combination of values derived for the overall ecosystem health targets and the specific ecological objective targets should be integrated into one target regime for each site. Land and water resource development should be managed in a manner that maintains the hydrologic statistics within the target ranges. Where this is not possible, management should attempt to direct the existing flow regime in the trajectory of the natural or reference regime. In situations where managers are restricted from making management changes that will maintain or direct the flow regime towards the natural regime, then acceptable percent deviation from the natural regime statistics should be evaluated based on an acceptable level of degradation of ecological objectives. Estimates of ecological responses to different changes in flow can be accomplished using tools such as those described above to quantify functional flows. However, identifying an acceptable level of degradation can be contentious. Further, the science and predictive models to quantify most ecological responses are still developing and it is important to recognize that determining an “acceptable” deviation remains an elusive goal. The target regime for site LOV1-01 is shown in Figure .
Application of targets to inform decision-making
A wide variety of land and water resource management decisions can affect streamflows. A suggested framework is given below for applying the target regime to inform decisions.
It is suggested that the hydrologic model be run for various development (e.g. low impact development vs. regular development) or restoration scenarios (e.g. low impact development retrofits). Each scenario should then be compared to the pre-development or reference condition within IHA to produce a series of hydrologic alteration factors. The hydrologic alteration of the different scenarios can then be compared to determine which scenario has the highest hydrologic alteration factors and thus the highest impact on the hydrological regime. The potential benefits of restoration or impacts of development on the overall health of the system can be evaluated by inferring potential ecological responses to the hydrological alterations. The assessment of these potential benefits and impacts will provide information to decision-makers that will lead to more effective restoration and more ecologically sustainable development.
The targets could also be applied post-construction to monitor the actual effect of the restoration or development on the system. This could be accomplished by comparing gauged data at the site to the reference and pre-construction streamflows to determine if the post-construction regime is trending towards the desired regime.
It is recommended that ecological objective targets be applied to aid decision-making in a similar manner as the targets for overall ecosystem health. This can be accomplished by modelling various development or restoration scenarios, calculating the ecosystem objective statistics and comparing the statistics of the different scenarios to determine the impact or benefit of a given scenario versus the other development or restoration scenarios.
Limitations and recommendations
Within this methodology, flow targets are primarily based on hydrological model output. Therefore, the adequacy of the targets is largely dependent on the accuracy of the hydrologic model. As discussed earlier, this methodology leverages sophisticated numerical models that have been developed by the province through a peer review process using qualified modelling professionals and advanced modelling software. However, the models were primarily developed for the purpose of evaluating risk to drinking water and likely require modification to be used for EFA. The models could be enhanced by improving key input datasets, parameterization and model calibration. Model improvement has been identified as a priority for the Provincial program tasked with water budget model development (WaterBudget.ca Citation2012). In addition, model uncertainty should be evaluated, which would provide an indication of the confidence that can be placed in the defined targets.
The confidence that can be placed in flow targets also relies on the accuracy of the quantified functional flows. Again, model enhancement through improving input data and model calibration associated with the quantification of each functional flow will increase certainty of the flow targets. This methodology is also limited by the assumption that that habitat health can be used as a proxy for species health. Specifically, the assumption that the variables chosen in the functional flow assessment can be related to ecological function objectives and that maintaining a variable within a chosen range will contribute to the accomplishment of subwatershed goals is made using this methodology. Scientific studies that evaluate the link between flow-related processes, the chosen variables and ecological response will further increase the certainty associated with the flow targets.
It is important to note that several decisions needed to be made throughout the course of developing flow targets for Lovers Creek. The scientific framework discussed in this paper needs to be supported by a decision-making framework. It is recommended that a decision-making framework be developed that would define key decisions and the appropriate decision-maker in order to ensure the defensibility of the targets. Finally, the framework and methodology are hierarchical. This means that more resources should be invested in increasing certainty in areas where water resources are under stress and/or there are particularly sensitive ecosystems depending on water resources.
Conclusions
This paper provides explicit steps for developing flow targets. While the methodology demonstrated was developed for the LSRCA, it is broadly transferable and can be applied beyond southern Ontario. Applying this methodology can produce target regimes that go well beyond the singular statistics that have been commonly used for environmental flow targets in Ontario to date. Improving model accuracy and testing flow ecological response hypotheses are important to achieve greater confidence in the target regimes. Developing a decision-making framework, and integrating this with the scientific framework, is recommended to increase the likelihood of successful implementation of target regimes. Setting environmental flow targets is viewed as an iterative process where targets should continually be revisited and improved based on new tools and information. As the methods described in this paper are continually applied and the targets are continually refined, more informed, sustainable decisions will be made that will lead to the enhanced protection and restoration of aquatic ecosystems.
Acknowledgements
The authors would like to extend their thanks to the individuals who provided insights for this article. They include Dr. Douglas Joy, Dr. John FitzGibbon, Dr. Ashraf Al Zaghal, Jack Imhof, Scott Bates and the Lake Simcoe Hydrology Team. Also, thanks to Gilbert Racine and an anonymous reviewer for translating the abstract.
References
- Acreman, M.C., and M.J. Dunbar. 2004. “Defining environmental flow requirements – A review.” Hydrology and Earth System Sciences 8(5): 861–7.
- Acreman, M.C., and J.D. Ferguson. 2010. “Environmental flows and the European Water Framework Directive.” Freshwater Biology 55: 32–48.
- Al Zaghal, A. 2010. Ecological streamflows with geomorphic functions. Phd diss., University of Guelph.
- AquaResource Inc. 2011a. Water budget and water quantity risk assessment guide. Breslau: Prepared for The Ontario Ministry of Natural Resources and The Ontario Ministry of the Environment.
- AquaResource Inc. 2011b. Technical memorandum: City of Barrie Tier Three – groundwater recharge estimation using MIKE SHE. Breslau: Prepared for the Lake Simcoe Conservation Authority.
- AquaResource Inc. and Golder Associates. 2010. City of Barrie Tier Three water budget and local area risk assessment conceptual understanding memorandum. Breslau: Prepared for The Ontario Ministry of Natural Resources and The Ontario Ministry of the Environment.
- Beaton, A. 2012. Testing and refining a unique approach for setting environmental flow and water level targets for a southern Ontario subwatershed. Master’s thesis, University of Guelph.
- Bradford, A. 2008. “An ecological flow assessment framework: Building a bridge to implementation in Canada.” Canadian Water Resource Journal 33(3): 215–32.
- Cottingham, P., G. Howell, P. Bennett, J. Boehm, J. Doolan, G. Hannan, J.B. Harris, et al. 2001. Perspectives on the scientific panel approach to determining environmental flows for south-eastern Australian rivers. Melbourne: Department of Natural Resources and Environment.
- Crance, J.H. 1987. Guidelines for using the Delphi technique to develop habitat suitability index curves. Fort Collins: US Fish and Wildlife Service Biological Report.
- Danish Hydraulic Institute (DHI). 2007. MIKE-SHE user manual. Volume 1: User guide. 2007 edition ed. Horsholm: Danish Hydraulic Institute.
- Grand River Conservation Authority (GRCA). n.d. Establishing environmental flow requirements for selected streams in the Grand River watershed – Pilot project. Cambridge: Prepared for Conservation Ontario.
- Jones, M.P., and W.F. Hunt. 2009. “Bioretention impact on runoff temperature in trout sensitive waters.” Journal of Environmental Engineering 135: 577–86.
- Lake Simcoe Region Conservation Authority (LSRCA). 2009. Lake Simcoe protection plan. Newmarket: Lake Simcoe Region Conservation Authority.
- Lake Simcoe Region Conservation Authority (LSRCA). 2010. South Georgian Bay-West Lake Simcoe study area Tier Two water budget and stress assessment. Newmarket: Lake Simcoe Region Conservation Authority.
- Lake Simcoe Region Conservation Authority (LSRCA). 2011a. Draft Lovers Creek subwatershed plan. Newmarket: Lake Simcoe Region Conservation Authority.
- Lake Simcoe Region Conservation Authority (LSRCA). 2011b. Draft city of Barrie Tier Three water budget and local area risk assessment conceptual understanding memorandum. Newmarket: Lake Simcoe Region Conservation Authority.
- Lake Simcoe Region Conservation Authority (LSRCA) and Bradford, A. 2011. Towards a framework for determining ecological flows in the Lake Simcoe Watershed – A guidance document. Newmarket: Lake Simcoe Region Conservation Authority.
- Leopold, L.B. 1968. Hydrology for urban land planning – A guidebook on the hydrologic effects of urban land use. Washington, DC: Department of the Interior.
- Maidment, D.R. 1993. Handbook of hydrology. New York: McGraw-Hill.
- Mathews, R., and B.D. Richter. 2007. “Application of the indicators of hydrological alteration software in environmental flow setting.” Journal of the American Water Resources Association 43(6): 1400–13.
- Ontario Ministry of Natural Resources (OMNR). 1994. Ontario wetland evaluation system southern manual. Peterborough: Ontario Ministry of Natural Resources.
- Ontario Ministry of Natural Resources (OMNR). 2010. Ontario low water response. Peterborough: Ontario Ministry of Natural Resources.
- Ontario Ministry of Natural Resources (OMNR). 2011. Lovers Creek wetland evaluation file. Midhurst: Ontario Ministry of Natural Resources.
- Pill, J. 1971. “The Delphi method: Substance, context, a critique and an annotated bibliography.” Socio-Economic Planning Science 5: 57–71.
- Poff, N.L., J.D. Allan, M.B. Bain, J.R. Karr, K.L. Prestegaard, B.D. Richter, R.E. Sparks, et al. 1997. “The natural flow regime: A paradigm for river conservation and restoration.” BioScience 47: 769–84.
- Poff, N.L., B.D. Richter, A.H. Arthington, S.E. Bunn, R.J. Naiman, E. Kendy, M. Acreman, et al. 2010. “The ecological limits of hydrologic alteration (ELOHA): A new framework for developing regional environmental flow standards.” Freshwater Biology 55: 147–70.
- Raleigh, R.F. 1982. Habitat suitability index models: Brook trout. Fort Collins: US Fish and Wildlife Service.
- Scott, W.B., and E.J. Crossman. 1985. Freshwater fishes of Canada. Ottawa: Fisheries Research Board of Canada.
- Stoneman, C.L., and M.L. Jones. 1996. “A simple method to classify stream stability with single observations of daily maximum water and air temperatures.” North American Journal of Fisheries Management 16: 728–37.
- The Nature Conservancy. 2009. Indicators of hydrologic alteration user’s manual version 7.1. Arlington: The Nature Conservancy.
- WaterBudget.ca. 2012. Ontario water budget program. http://www.waterbudget.ca (accessed December, 2012).
- Withers, P.J.A., and H.P. Jarvie. 2008. “Delivery and cycling of phosphorus in rivers: A review.” Science of the Total Environment 400: 379–95.