Abstract
This paper compares the economic and environmental performance of a share versus a prior allocation system for managing water in Alberta’s South Saskatchewan River Basin. Currently, water is allocated on a priority basis, and moving to a share system would involve significant political and legal challenges. In the absence of water trading, both initial allocation systems result in poor economic outcomes, but the prior allocation performs particularly badly. Efficiency improves with water trading as licensees respond to opportunities to reallocate water to higher value uses. However, under prior allocation, water is more concentrated with senior licensees who capture more of the gains from trade. The share system does not result in improved environmental outcomes. Water trading results in improved instream flows as water is reallocated to upstream municipal uses, which have high return flows. The analysis suggests that improving institutions for trading water will provide better economic outcomes and environmental protection than reforming the initial allocation, and that government should focus its efforts on directly reserving water for the environment.
Cet article présente une comparaison des performances économiques et environnementales d’un système de répartition partagée par rapport à un système de répartition prioritaire pour la gestion de l’eau du bassin de la rivière Saskatchewan Sud. À l’heure actuelle, l’eau est répartie selon des priorités établies; passer à un système de répartition partagée entraînerait d’importants enjeux politiques et juridiques. En l’absence de commerce de l’eau, les deux premiers systèmes de répartition procurent de mauvais résultats économiques, mais la performance du système de répartition prioritaire est particulièrement pauvre. Le commerce de l’eau augmente l’efficacité, car les détenteurs de permis profitent de la possibilité de répartir l’eau vers des utilisations à valeur accrue. Par contre, dans le cadre de la répartition prioritaire, l’eau est plus centralisée et les principaux détenteurs de permis empochent une plus grande partie des gains. Le système partagé n’améliore pas les résultats environnementaux. Le commerce de l’eau entraîne une amélioration des débits, car l’eau est répartie à des usages municipaux en amont, lesquels ont de grands débits de retour. Selon l’analyse, l’amélioration des institutions de commerce de l’eau améliorerait davantage les résultats économiques et la protection de l’environnement qu’une réforme de la répartition initiale, et le gouvernement devrait concentrer ses efforts afin de réserver directement l’eau pour l’environnement.
Introduction
The resilience of Alberta’s water allocation system is a continuing concern in the South Saskatchewan River Basin (SSRB) where demands for water continue to rise due to expanding municipal and irrigation needs, while supply is becoming increasingly variable due to climate change (e.g. Bow River Project Research Consortium Citation2010). In 2009, during Alberta’s renewal of its Water for Life policy, the government undertook a review of the water allocation and transfer system to determine a need for reform in the context of anticipated future changes in water supply and demand conditions. Government and stakeholder reports produced during the review focused on the ability of the current priority-based water allocation system to adapt to increased variability in flows, and the potential negative impact of water transfers on instream flows and water conservation objectives in the SSRB (e.g. Alberta Water Council Citation2009; Alberta Water Research Institute Citation2009; Droitsch and Robinson Citation2009; Minister’s Advisory Group on Water Management and Allocation Citation2009). In particular, there is a perception that a share system, where water allocations are based on percentages of water available, rather than fixed volumes, is better able to manage water supply risk and is more equitable to users (Droitsch and Robinson Citation2009).
While most stakeholders support continuation of the prior allocation system in the short to medium term, there is still concern that, over the long term, Alberta’s prior allocation system will not succeed at meeting Alberta’s water management objectives (Alberta Water Research Institute Citation2009). Distributional concerns over risk sharing between licensees during shortages under the current system have led some stakeholders to recommend moving to a share system as a way to more fairly allocate risks (Droitsch and Robinson Citation2009). This paper develops a water allocation model to compare the economic and environmental performance of alternative water allocation and transfer options in the SSRB, and evaluates the need for further reform.
Water allocation in the SSRB
In Alberta, water is Crown owned and allocated under the Water Act through a license system that allows users to withdraw water from a specified source at a particular diversion point to a fixed project or facility (Alberta Citation1996). Water licenses specify an annual diversion volume and an instantaneous diversion rate as well as the purpose for the withdrawal. Each license has a priority date based on the date of the completed license application. During shortages, older senior licenses receive priority, and must be filled before junior licenses.
The SSRB includes the Bow, Red Deer, Lower South Saskatchewan and Oldman River sub-basins (Figure ). The total water available for allocation in the SSRB is constrained by the Master Agreement on Apportionment (Prairie Provinces Water Board Citation1992). Under the agreement, Alberta must pass 50% of the natural flow under normal conditions to Saskatchewan in a given year. One indicator of whether limits to allocation are being reached is the level of allocation as a percentage of median flow. This percentage shows, in an average year, how much water is allocated relative to the apportionment constraint (Alberta Environment Citation2005). While the Red Deer Basin’s allocation is only 18.4% of median flows, the Bow and Oldman River allocations, where irrigated agriculture is concentrated, are 68 and 70% of median flows, respectively. The Southern tributaries of the Oldman River are particularly strained with allocations for the St. Mary, Belly and Waterton sub-basins at 118, 80 and 75% of median flows, respectively. Over the entire SSRB, the licensed allocation represents 68% of median flows with estimated consumption at 39% of median flows (Alberta Environment Citation2005).
Approximately 75% of water allocation in the SSRB is in the form of senior licenses for irrigation. Irrigation demand increases in dry years, intensifying low-flow scarcity issues. Municipal use is the second largest use, accounting for approximately 14% of water allocations. Municipal allocations range in seniority. Although there are some senior licenses, many municipal licenses have lower priority than irrigation district licenses, which puts municipal water use at risk during low-flow years (e.g. Droitsch and Robinson Citation2009). The third largest licensed use is for hydropower generation. TransAlta owns six large hydroelectric reservoirs in the upper Bow River, which store approximately 25% of the average annual runoff of the Bow River at Calgary (Bow River Basin Council Citation2005). Reservoirs are filled during the spring and summer, and depleted during the winter. Winter releases are particularly important for maintaining flows during winter months when flows are naturally low. Return flows also play an important role in water allocation, as they support instream flow needs and are available for re-allocation to downstream uses. Return flows vary by use, ranging from 30% of withdrawals for irrigation to 70–90% for industrial uses, and 95% for residential consumption (Bow River Basin Council Citation2005).
Instream flows are protected through license constraints on flow conditions at the point of diversion as well as through water conservation objectives (WCOs). WCOs were established under the 1999 Water Act to define the desired quantity and quality of instream flows for a basin. WCOs for the Bow, Oldman and Lower South Saskatchewan Basins were generally established at 45% of long-term natural flow. Quantities required to meet WCOs vary in amount and timing throughout the year to reflect seasonal variation in natural flows (Alberta Environment Citation2007a, Citation2007b, Citation2007c, Citation2007d). Under the Water Act (Alberta Citation1996), WCOs can be established either through setting water aside (e.g. through a Crown Reservation) or through the creation of WCO licenses with junior priority, based on the date the license is established. In the SSRB, WCOs are managed through licenses held by the Crown.
Although the amount of water allocated sometimes exceeds natural flow, many licensees do not use their full licensed allocation, which leaves sufficient water to meet current demands in average years. Nonetheless, there are still frequent deficits to junior licensees, and insufficient instream flows to meet WCOs in some reaches. Irrigation deficits are minor for current irrigated acreage, but by 2030, irrigated water use is expected to increase by more than 50% over 2005 levels (AMEC Citation2009). Climate change, along with expanded irrigation acreage, could put enormous stress on the system since water consumed per hectare would increase just as availability decreases under a warmer and, potentially, drier climate.
To improve the ability of the system to reallocate water to high value uses, the 1999 Water Act (Alberta Citation1996) enabled intra-basin water transfers under a cabinet-approved basin water management plan. Permanent and temporary transfers allow licensees to trade part or all of their licensed allocation, including priority and all other conditions, to another licensee or a new entrant. Assignments allow one licensee to temporarily transfer water to another licensee up to the amount of the buyer’s licensed allocation. Since no new water can be added to licenses through the assignment process, it is equivalent to transferring priority.
Phase 1 of the SSRB Water Management Plan established the rules for water transfers in the SSRB (Alberta Environment Citation2002). A public review of every permanent or temporary transfer is required to assess the potential for third-party effects and hydrological consequences. In addition, all permanent and temporary transfers are subject to a 10% holdback rule, which allocates water to a WCO license with the priority date of the original transferred license. To date, the amount of water restored to WCOs from transfers has been small and is not expected to be a significant factor in meeting WCOs in the SSRB (AMEC Citation2009). Transfers through assignments are more informal. Administratively, the important advantage of assignments is that there is no formal review process and no trigger for a 10% holdback. The extent and conditions under which assignments have been used in the SSRB have not been systematically tracked.
Share versus prior allocation
Share and prior allocations have different efficiency and equity implications in response to supply shortages. Prior allocations are rights to fixed volumes of water for a specific time period – for example, an annual volume per year. Each allocation has a priority date, which determines the risk to the licensee of changes in water availability as senior licensees can call on all of their water before junior licensees. Share allocations are not defined as fixed volumes. Rather, they are rights to a share of the pool of water available, and actual annual allocated volumes vary according to the amount of water available in the pool in a given year.
The merits of one system over another depend on basin characteristics. Under prior allocation, water shortages result in some licensees experiencing withdrawal interruptions. Under a share system, everyone receives less water but there are no withdrawal interruptions. Thus, the relative efficiency of the initial allocation ultimately depends on the distribution of water rights relative to water values, as well as the distribution of return flows. The initial allocation under a share system is more equitable because water is reallocated from senior licensees with full allocation to junior licensees with none. This generally results in greater economic benefits because junior licensees typically have higher marginal values for water (Burness and Quirk Citation1979, Citation1981; Bennett et al. Citation2000). However, junior licensees may have factored increased risk into their decisions and have more elastic demands and/or lower willingness to pay than senior licensees. He et al. (Citation2012) analyzed moving from prior allocation to a share allocation for agricultural licensees in the Bow River Basin. The main result is that under the share allocation, crop area could be expanded by 1–13% with 10–40% less water, resulting in a significant welfare improvement. However, the analysis did not include an assessment of impacts on non-agricultural uses and environmental flows, nor did it consider the ability of trading to achieve the same outcomes.
The efficiency differences driven by the initial allocation under share and prior rules disappear with additional mechanisms for managing supply risk, such as water trading as well as spot and option markets (An and Eheart Citation2006). However, the two systems may still continue to differ with respect to transactions costs. For example, Freebairn and Quiggen (Citation2006) found that a water allocation system with two priorities (high and low) outperforms a share system, because the latter requires more trades to achieve efficiency and transactions costs are a barrier to efficiently improving trades.
Methodology
SSRB water allocation model
To evaluate alternative water allocation strategies, a water allocation model was developed using Aquarius version 10 (Diaz et al. Citation2000). Aquarius is an economic-hydrologic model, which calculates the optimal allocation of water among competing users in a river basin. The SSRB is represented in Aquarius as a network of approximately 70 demand and supply nodes (Figure ). The model runs on a monthly time step and allocates water on a monthly basis to each node over a calendar year. The model includes approximately 400 decision variables corresponding to a monthly allocation for each demand node, and 900 constraints including monthly inflows and water balances at each node, reservoir storage rules, license conditions and apportionment requirements.
Figure 2. The South Saskatchewan River Basin (SSRB) in Aquarius. The SSRB is represented in Aquarius as a network of approximately 70 demand and supply nodes. This figure provides a snapshot of how the network is modeled in the SSRB with diversion points and return flows along the reach. ID: irrigation district; IFN: instream flow needs; WCO: water conservation objective.
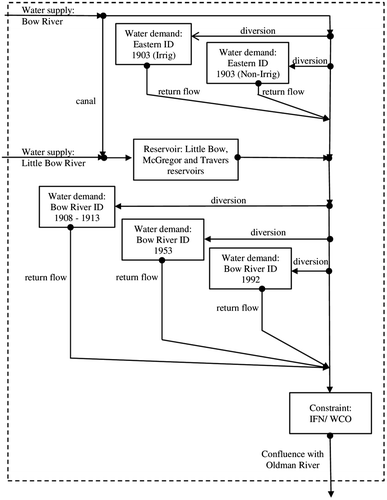
Calibration of the Aquarius model requires information on water supply and demand including: natural flows, characteristics and rules for operating regulating features such as reservoirs, licensed water uses and return flows, and water demand functions that determine the economic value of competing water uses. Physical parameters and estimates of water demand functions are drawn from Weber and Cutlac (Citation2010) and Cutlac and Horbulyk (Citation2011). Natural flow data were obtained from the Water Survey of Canada (Environment Canada Citation2010), which provided monthly inflow data for 14 headwater systems in the SSRB. In order to understand the vulnerability of the SSRB under dry conditions, the model was calibrated to the 2001 drought during which natural flows ranged from 53% to 72% of the long-term average, depending on the sub-basin (Table ).
Table 1. The Aquarius model was calibrated to the 2001 drought to understand the vulnerability of the South Saskatchewan River Basin (SSRB). Natural flows are shown as a percentage of long-term average per sub-basin.
Reservoir storage for hydropower and other uses is aggregated in nine reservoir nodes. The storage capacities of the lakes and reservoirs in the Upper Bow sub-basin including Spray, Barrier, Upper and Lower Kananaskis Lakes and Lake Minnewanka are captured by a single reservoir. Glenmore Lake stores water upstream of Calgary on the Elbow River, while the Little Bow, McGregor and Travers reservoirs are aggregated into a single reservoir that supplies water to the Bow River Irrigation District (ID). In the Oldman River, the Oldman, Pine Coulee, Payne Lake, Waterton and St. Mary reservoirs are individually modeled. In the Red Deer River, Glennifer Lake is represented. Contributions from Gull Lake and Sylvan Lake to the Red Deer River are also considered, but they have an insignificant impact on the system. Initial and final storage rules, as well as minimum and maximum storage capacity, are imposed as operational constraints for each reservoir. Final storage volumes are replenished to initial levels at the end of the year. Specific area-storage and elevation-storage curves are derived based on data from the Atlas of Alberta Lakes (Mitchell and Prepas Citation1990). Monthly net evaporation from the lakes is also taken into account (Prairie Provinces Water Board Citation1952, Citation1957).
Each water use is represented as an individual demand node drawing water from the source specified in the license, and returning a percentage of flow back to the river for reuse downstream. The model has 34 demand nodes representing 54 licenses with priority dates ranging from 1894–2001 and accounting for approximately 80% of water use in the basin. Licenses were aggregated into a single demand node if they were issued for the same use and same user, and were geographically close in terms of diversion point and seniority. Return flows from each license are treated separately to eliminate the possibility of return flows being used to fulfill more junior licenses at the same diversion point. License data were obtained from Alberta Environment’s online license viewer (Alberta Environment Citation2012), and augmented where necessary by additional information from Alberta Environment (Citation2005) and the Irrigation Water Management Study Committee (Citation2002).
Each license provides data on use, point of diversion, water source, annual allocated volume, priority date and additional withdrawal conditions such as minimum flow rates. In order to examine the resilience of the current allocation system to future stresses caused by increased water use in the SSRB, it is assumed that licensed allocations are fully utilized and that water is diverted up to the fully licensed amount. This is important because it illustrates the resilience of the different allocation rules under full build-out of irrigation and municipal uses in the basin. Estimates of consumptive use and return flows associated with each license are based on Thompson (Citation1990), the Bow River Basin Council (Citation2005) and the Irrigation Water Management Study Committee (Citation2002), and range from 15–35% for agricultural irrigation water use, 90% for the cities of Calgary and Red Deer and 70% for Lethbridge and Medicine Hat.
To determine monthly water allocations, annual diversions for municipalities are distributed monthly according to reported seasonality patterns for cities (Hydroconsult EN3 Services Citation2002). Monthly irrigation diversions are distributed as 15, 22, 30, 20, 10 and 3% of the annual allocation from April to September, and 0% for the remaining fall and winter months (Cutlac Citation2005). Economic demand parameters for municipalities were obtained from Lo (Citation1995), Horbulyk and Lo (Citation1998) and Cutlac (Citation2005). Irrigation demand parameters were derived from Mahan (Citation1997) and Mahan et al. (Citation2002). Hydroelectric water use from the 11 plants located in Upper Bow is represented by a generic plant with 325-MW-installed capacity and a design discharge of 245 m3/s. Power generation is assumed to be independent of the amount of water stored in the upstream reservoir. Since hydroelectric production is exogenous in this model, demand functions for this sector are not considered.
Environmental uses are represented by two instream flow protection nodes placed at the headwater (upstream) and the mouth (downstream) of each sub-basin. Instream use nodes are specified as junior licenses – in other words, they receive the residual water after the rest of the demands are allocated. Environmental outcomes are determined by comparing water allocated to instream nodes under each allocation scenario to the minimum instream flow requirements for fish habitat (Alberta Environment Citation1984) as well as WCOs for each sub-basin (Alberta Environment Citation2007a, Citation2007b, Citation2007c, Citation2007d). Apportionment requirements are represented as constraints, which are applied at the border with Saskatchewan.
Water allocation scenarios
Four water allocation scenarios based on two initial allocations and two water transfer rules are examined. The prior and share allocation rules define the initial allocation of water and distribution of risk to licensees in a given year. Under prior allocation, water is allocated based on the application date of the license. During a water shortage, all senior licenses must be completely filled before the next license can be filled. The share allocation rule considered in this analysis is a hybrid combination of priority and share rules. Under this rule, licenses maintain their priority, but the percent reduction in volume available relative to long-term average flows is translated into an equivalent percent reduction in annual allowable withdrawals for each licensee. The 2001 percent reduction in median flows was translated into a corresponding reduction in volume for each licensee for each sub-basin according to Table . Because of sub-basin differences in changes in flows, the pattern of return flows, and the location and specific characteristics of storage, it is possible even under a share system to have some licenses that cannot be filled because instream flow constraints are binding. The hybrid share rule applied in the model allocates the pro-rated water demands based on seniority, and also shows how desirable properties of a share system could be implemented without completely eliminating priority, which could be viewed as politically infeasible.
For reallocation, both a restricted and a full trade scenario are considered. The restricted scenario represents the assignment system. Trade is restricted to transfers of water between existing licensees up to licensed allocation; licensees cannot purchase additional volume above the licensed allocation. This scenario is equivalent to trading priority, allowing senior licensees to transfer water to junior licensees that are experiencing a shortfall. The full trade scenario transfers the entire license and all of its conditions to the highest benefit use. In the model, the most important difference between these scenarios is that full trading allows users to purchase additional allocation. As shown in Johnson et al. (Citation1981) and Weber (Citation2001), full trading maximizes the value of water in a river, subject to network constraints. Therefore, the full trading scenario is also considered the optimal benchmark solution in this analysis. Note that the priority of allocation does not matter for the outcome of the trading scenario; it is only relevant for the initial allocation unless third-party constraints are binding. For the purposes of the scenario comparison, it is assumed that all physically feasible efficient trades take place. This assumes that parties are able to compensate for third-party effects, which arise due to binding flow constraints on third parties with higher priority. In reality, third-party effects may block some efficient trades. In addition, there are other administrative and transactions costs associated with water trading, such as the 10% holdback rule and the uncertainty of the review process. Therefore, the actual trading solution will lie somewhere between the assignment solution and the hypothetically optimal benchmark solution.
The model
The Aquarius model is a non-linear programming model that optimally allocates water according to the economic demand functions at each node until marginal values are equalized or system constraints, such as reservoir operating rules or instream flow requirements, are binding. The initial allocations under the share and prior rules are not based on economic demands. To implement the initial allocation in Aquarius, surrogate demand curves are used to force the model to replicate the initial allocation (Diaz et al. Citation2000). For the prior allocation rule, the surrogate demand curves are represented by individual horizontal demand curves for each license ordered by priority date, so that the most senior licensee has the highest willingness to pay and receives water until the full license is allocated, and so on, with the most junior licensee having the lowest willingness to pay. Water is allocated to these ordered horizontal demand curves up to their initial allocation until supply constraints are binding.
The surrogate demand functions force the initial prior allocation under both the prior and share allocation rules. Once the initial allocation is determined, the quantities allocated to each licensee under each initial allocation scenario are substituted into the actual demand functions to determine the marginal value of water to each licensee and the total economic surplus generated by each scenario. In the trading scenarios, water is allocated in Aquarius according to actual water demands, until the marginal value of water is equated between demand nodes, or system constraints are binding. In the restricted trade scenario, water demands are truncated and fall to zero once the allocation reaches the licensed volume. As in the initial allocation, the marginal values of water under trading are used to examine the value of water under each of the scenarios relative to the optimal benchmark.
Results
Economic impacts
A comparison of the economic efficiency of the initial allocation rules is presented in Table . Impacts are broken down by each sub-basin (Red Deer [RDR], Bow River [BR], Oldman River [OMR], and Lower South Saskatchewan [LSSR]) as well as summarized over the entire SSRB. The first three rows of Table show the licensed allocation for each sub-basin. The remaining rows show the modeled results including the number of licenses that are fully allocated, partially allocated and unallocated under each scenario; the cut-off year after which junior licenses are unallocated; modeled withdrawals and consumptive use by sub-basin; and the economic value of each scenario as a percent of the optimal benchmark.
Table 2. Comparison of economic efficiency of the initial allocation rules. Economic benefits improve under a share rule, but the system only achieves 56% of potential economic value.
Slightly less than half (48%) of licenses receive a full allocation under the prior allocation rule, compared to 72% under the share allocation rule. Similarly, the number of partially and completely unallocated licenses falls under the share system. The reallocation takes place in the Bow and Oldman River sub-basins. At the same time, the total allocation falls under the share system, as does the total amount of water actually used. The explanation for the reduction in the total allocation and use is that larger irrigation licenses that have higher consumptive use are forced to cut back more than smaller municipal licenses. The LSSR is actually worse off under the share rule. This is because the LSSR experiences a disproportionately large reduction in water availability relative to the other sub-basins, which is applied to two senior licenses held by Medicine Hat. These licenses would have received their full allocation under prior allocation. At the same time, the only other license in the LSSR, which is also held by Medicine Hat, has a priority year of 1986 so it does not receive any compensating water under the share system. Across the SSRB as a whole, the economic value of the allocation increases from 41 to 56% under the share system due to improved risk sharing between junior and senior licensees.
While economic benefits improve under a share rule, the system still only achieves 56% of potential economic value. This can be compared to the impact of the water trading scenarios, which are presented in the last seven rows of Table . The baseline comparison for the water trading scenarios is the no-trade prior allocation rule. The numbers of buyers and sellers under each scenario are defined as licensees who end up with higher and lower water allocations, respectively, than their initial allocation under the prior allocation rule. Note that the number of buyers and sellers does not have to be equal as some sellers may reallocate water to multiple buyers. In addition, some sub-basins only have buyers, implying trade between sub-basins. Since the model considers all operational and physical constraints, any trades between sub-basins identified by the model are physically feasible. However, there may be other administrative constraints and transaction costs that create barriers to trades between sub-basins. The economic benefits from trade are calculated at a sub-basin and basin level.
Under the full trading benchmark, all licensees reallocate water, and no license is cut off from allocation. While the assignment scenario has the same number of sellers as the full trading scenario, it has fewer buyers. Generally, irrigators with senior licenses are sellers, while municipalities and irrigators with junior licenses are buyers. Trading leads to a large increase in the amount of water allocated, with an increase of 80% under the full and 60% under the restricted trade scenarios relative to the baseline. The increases in water allocation are due to increased return flows and opportunities for re-use from reallocation of licenses to the municipal sector from the irrigation sector. Less flow is allocated overall under the assignment scenario, primarily because less water is transferred to upstream municipal uses. The 23% increase in the value of water under full trading relative to restricted trading shows that the transfer of priority is not sufficient to overcome the initial misallocation of licenses under the prior allocation rule.
Table shows how water is allocated between municipal and irrigation licensees under each of the four scenarios. Table illustrates the marginal values of different uses at the solution. In general, municipal uses have a higher marginal value than agricultural uses. This phenomenon is consistent with observed data from water trades in the US, where water is typically transferred from agricultural to municipal uses (Brown Citation2006).
Table 3. Diversion and consumption by use (Mm3). This illustrates how water is allocated between municipal and irrigation licensees under each of the four scenarios. The first column under each sub-basin shows the amount of water diverted (div.), while the second column shows the amount of water consumed (con.).
Table 4. Monthly marginal values for selected uses by scenario (CAD$2013). By showing the marginal values of different uses at the solution, it is found that municipal uses have a higher marginal value than agricultural uses. This Alberta phenomenon is consistent with water trades in the US where water is typically transferred from agricultural to municipal uses (Brown Citation2006).
The increase in the total economic value of the share system relative to the prior allocation system is explained by the reallocation of water from the agricultural to the higher value municipal sector whose allocation of water increases from 66 dam3 to 213 dam3. Demand from the City of Calgary in the Bow River Basin is a significant factor in the value differences between alternative scenarios. In the Bow, municipal uses account for 21% of licensed allocation, but only 2% of consumption because of high return flows. This can be compared to irrigation, which accounts for 79% of the licensed allocation and 51% of consumption. Under the prior allocation system, the low-flow scenario results in the irrigation sector receiving 99% of the water due to seniority and the size of the licenses. Municipalities receive only 1% of allocated water. This explains the very high marginal value of water ($2800 per dam3) for Calgary under the prior allocation scenario, since it only has one license of vintage 1895 that can be satisfied.
The municipal allocation of water increases from 66 dam3 (10% of the licensed allocation) under prior allocation to 531 dam3 (84% of the licensed allocation) under restricted trading. Municipalities, such as the City of Calgary, receive less water under the assignment system than is economically optimal, while smaller municipalities have marginal values that are competitive with the irrigation sector and therefore do not use their full allocation. In addition, the total amount of water available for use is less under restricted trading because of the high return flow values for the City of Calgary.
With full trading, municipal allocation increases to 1514 dam3, 2.4 times the licensed allocation and 23 times the initial prior allocation. Nonetheless, only 182 dam3 of water is consumed by municipal uses, which is only a fraction (12%) of the 1519 dam3 consumed in total under the full trade scenario. The City of Calgary receives 58% of the allocated water, and the marginal value of water drops to $8–11 per dam3. The low marginal value of water for the City of Calgary is because Aquarius equalizes the social marginal value of water allocation. This accounts for both Calgary’s direct marginal value of water use as well as the all-downstream values from re-use of return flows from Calgary. The model subsidizes Calgary’s water use since the water can be reused downstream, leading to spatially differentiated prices at the optimum. This is equivalent to the optimal pricing results, which are shown to be supported by a water market in Weber (Citation2001) and Murphy et al. (Citation2009). Finally, note that there is less variation in marginal water values as restrictions are relaxed on the initial allocation, moving from the prior allocation through the share, restricted trading and full trading scenarios. Continued price differentials after full trading reflect the implicit subsidization of upstream water users with high return flows. Price differentials are also caused by system constraints resulting from infrastructure operating rules and return flow patterns.
Environmental impacts
The impact of the initial allocation as well as trading on environmental constraints is shown in Table . WCOs and instream flow needs (IFNs) for the Bow, Oldman and Red Deer Rivers are calculated. The WCOs for the sub-basins, specified in the SSRB Water Management Plan, are 45% of long-term natural flow (Alberta Environment Citation2007a, Citation2007b, Citation2007c, Citation2007d). IFNs are based on 1984 requirements to protect fish habitat (Alberta Environment Citation1984). IFNs allocate more water than WCOs during the winter months. In particular, IFNs demand larger amounts of water from August to April and, in some cases, 100% of the natural flows. WCOs reflect the natural seasonal changes in water availability, peaking in the spring and summer months.
Table 5. Violation of instream flow needs (IFN) and water conservation objective (WCO) constraints per allocation scenario.
Table shows the impact of each allocation scenario on WCOs and IFNs. The ability of the system to meet WCOs and instream flow requirements is indicated by the number of months in which these requirements are violated for both the source and the mouth of each sub-basin.
The environmental impacts of the different policies are mixed. The prior allocation scenario performs the worst, resulting in 14 violations of WCO constraints and 30 violations of IFN constraints across the three sub-basins. Moving to the share system does not improve the situation for WCOs; however, the number of violations of IFN constraints decreases by 23% to 23. The restricted trading and full trading scenarios perform best at achieving WCOs, with the total number of violations falling from 14 to 11 and 10, respectively. It is interesting to note that the distribution of WCO violations between sub-basins differs between the two trading scenarios. The trading scenarios are better than the share system at achieving WCOs because they allocate more water to municipal uses, which increases the amount of flows in the system through return flows. Therefore, even though final consumption is higher than under the share system, these systems allocate more water to the environment because they maximize return flows through moving water to municipal uses. In terms of IFNs, the restricted trade scenario fails nearly as frequently as the initial allocation, resulting in 29 constraint violations. The results for IFNs under full trading are very similar to those under the share system, with 24 violations in total (a reduction in 20% compared to the prior allocation scenario).
Conclusions
A share allocation system has been advanced as a mechanism to better manage risks of water shortage to junior licensees and the environment in the SSRB. While some literature suggests that there could be improved economic and environmental outcomes under a share allocation system, moving to such a system would require a significant shift in the way water rights are allocated, and would be politically challenging. Economic and environmental outcomes of different water allocation systems are compared under a low flow regime. The initial distribution of water under both the prior and share allocation rules is shown to be economically inefficient; the redistribution of water from senior to junior licensees under the share system only increases the value of water to 56% of its potential value in the system. The assignment system performs substantially better than the share system at managing risk to junior licensees, as shown by the increase in the value of water to 77% of potential. Still, the inefficiencies of the initial prior allocation persist. Moving to a share system also does not lead to an improvement in meeting environmental objectives. This is because, under a share rule, water goes first to junior irrigation and municipal licenses before being allocated to WCOs, resulting in no change to environmental outcomes.
The results suggest that both environmental and economic objectives would be better served by improving institutions for water trading. Under full trading, more water is allocated to municipal licensees with higher marginal values and higher return flows, which can support environmental objectives. The current structure of Alberta’s water market is inefficient. The uncertain review process and holdback rules on water transfers are impediments to trade, which ultimately do not improve environmental outcomes. Furthermore, there are no formal institutions to match buyers and sellers or track market prices, resulting in high search and negotiation costs. Defining transferable portions of licenses in terms of consumptive uses and/or creating markets for return flows would reduce the potential for third-party constraints to impede efficient trades.
The analysis highlights the equity implications of the current initial allocation, which forces junior licensees to contract with senior licensees. The concentration of senior licenses in irrigation districts may create market power. In addition, the current allocation provides an opportunity to raise fears about junior municipal licensees being held hostage to water market prices in times of shortage. The equity implications could be addressed by moving to a share-based system; however, this would still not eliminate the need for a water market to improve efficiency and environmental outcomes. Finally, the results do not imply that environmental flows are adequately protected under any of the above regimes, but rather that a share system per se is unlikely to address environmental concerns. The key challenge facing environmental uses is the number of more senior licenses ahead of WCO licenses in the queue. This analysis suggests that environmental objectives will best be achieved not by changing the allocation system, but by using direct mechanisms to restore water to meet WCOs including Crown reserves, reverse auction buy-backs, and provide opportunities for third parties such as environmental trusts to purchase or lease water for the environment.
References
- Alberta. 1996. Water Act. Revised statues of Alberta 2000. Chapter W-3. Edmonton, AB: Queen’s Printer.
- Alberta Environment. 1984. South Saskatchewan River Basin planning program – Fishery component – Desired flows and scenario evaluation. Water Resources Management Services. Edmonton, AB: Alberta Environment.
- Alberta Environment. 2002. South Saskatchewan River Basin water management plan, Phase 1. Water allocation transfers. Edmonton, AB: Alberta Environment.
- Alberta Environment. 2005. South Saskatchewan River Basin water allocation. May 2003, Revised January 2005. Edmonton,AB. Alberta Environment.
- Alberta Environment. 2007a. Establishment of Bow River sub-basin water conservation objectives. Edmonton, Alberta: Alberta Environment.
- Alberta Environment. 2007b. Establishment of Oldman River sub-basin water conservation objectives. Edmonton, AB: Alberta Environment.
- Alberta Environment. 2007c. Establishment of Red Deer River sub-basin water conservation objectives. Edmonton, AB: Alberta Environment.
- Alberta Environment. 2007d. Establishment of South Saskatchewan River sub-basin water conservation objectives. Edmonton, AB: Alberta Environment.
- Alberta Environment. 2012. Water allocation licence viewer search system. http://ssrb.environment.alberta.ca/licence_viewer.html (accessed October, 2012).
- Alberta Water Council. 2009. Recommendations for improving Alberta’s water allocation transfer system. Edmonton, AB: Alberta Water Council.
- Alberta Water Research Institute. 2009. Towards sustainability. Phase I: Ideas and opportunities for improving water allocation and management in Alberta. Edmonton, AB: Alberta Water Research Institute.
- AMEC. 2009. South Saskatchewan River Basin in Alberta: Water supply study. Lethbridge, AB: Alberta Agriculture and Rural Development.
- An, H., and J. W. Eheart. 2006. Evaluation of programs for regulating withdrawal of surface water under the riparian legal system. Journal of Water Resources Planning and Management 132(5): 385–394.10.1061/(ASCE)0733-9496(2006)132:5(385)
- Bennett, L. L., C. W. Howe, and J. Shope. 2000. The interstate river compact as a water allocation mechanism: Efficiency aspects. American Journal of Agricultural Economics 82(4): 1006–1015.10.1111/ajae.2000.82.issue-4
- Bow River Basin Council. 2005. Nurture, renew, protect: A report on the state of the Bow River Basin. Calgary, AB: Bow River Basin Council.
- Bow River Project Research Consortium. 2010. Bow River project: Final report. Edmonton, AB: Alberta Water Research Institute.
- Brown, T. C. 2006. Trends in water market activity and price in the western United States. Water Resources Research 42: W09402. doi:10.1029/2005WR004180.
- Burness, H. S., and J. P. Quirk. 1979. Appropriative water rights and the efficient allocation of resources. The American Economic Review 69: 25–37.
- Burness, H. S., and J. P. Quirk. 1981. Water law, water transfers, and economic efficiency: The Colorado River. Journal of Law and Economics 23: 111–134.
- Cutlac, M. 2005. Economics models of surface water allocation. MA thesis, University of Calgary.
- Cutlac, M., and T. Horbulyk. 2011. Optimal water allocation under short-run water scarcity in the South Saskatchewan River Basin. Journal of Water Resources Planning and Management 137(1): 92–100.10.1061/(ASCE)WR.1943-5452.0000092
- Diaz, G., T. C. Brown, and O. Sveinsson. 2000. Aquarius: A modeling system for river basin allocation. General technical report RM-GTR-299. Forest Services. Rocky Mountain Forest and Range Experiment Station. Fort Collins, CO: US Department of Agriculture.
- Droitsch, D., and B. Robinson. 2009. Share the water: Building a secure water future for Alberta. Canmore, AB: Water Matters Society of Alberta and Ecojustice.
- Environment Canada. 2010. Water Survey of Canada – HYDAT. Archived hydrometric data. http://www.wsc.ec.gc.ca/applications/H2O/index-eng.cfm (accessed November, 2010).
- Freebairn, J., and J. Quiggin. 2006. Water rights for variable supply. The Australian Journal of Agricultural and Resource Economics 50: 295–312.10.1111/ajar.2006.50.issue-3
- He, L., T. Horbulyk, A. M. Kamar, D. G. Le Roy, and K. K. Klein. 2012. Proportional water sharing vs. seniority-based allocation in the Bow River Basin of southern Alberta. Agricultural Water Management 104: 21–31.10.1016/j.agwat.2011.11.008
- Horbulyk, T. M., and L. Lo. 1998. Welfare gains from potential water markets in Alberta, Canada. In Markets for water: Potential and performance, eds. K. W. Easter, M. W. Rosegrant and A. Dinar, 241–258. Boston, MA: Kluwer Academics.10.1007/b102479
- Hydroconsult EN3 Services. 2002. South Saskatchewan River Basin non-irrigation water use forecasts. Edmonton, AB: Alberta Environment.
- Irrigation Water Management Study Committee. 2002. South Saskatchewan River Basin: Irrigation in the 21st Century. Volume 1: Summary Report. Alberta Irrigation Projects Association.
- Johnson, R. N., N. Gisser, and M. Werner. 1981. The definition of a surface water right and transferability. Journal of Law Economics 24: 273–288.10.1086/jle.1981.24.issue-2
- Lo, L. J. 1995. Water scarcity and the potential gains from water trading in Southern Alberta. MA thesis, University of Calgary.
- Mahan, R. C. 1997. Efficient allocation of surface water resources in southern Alberta. MA thesis, University of Calgary.
- Mahan, R. C., T. M. Horbulyk, and J. Rowse. 2002. Market mechanisms and the efficient allocation of surface water resources in southern Alberta. Socio-Economic Planning Science 36(1): 25–49.10.1016/S0038-0121(01)00013-1
- Minister’s Advisory Group on Water Management and Allocation. 2009. Recommendations for improving Alberta’s water management and allocation. Edmonton, AB: Minister’s Advisory Group on Water Management and Allocation.
- Mitchell, P., and E. Prepas. 1990. Atlas of Alberta lakes. Edmonton, AB: University of Alberta Press.
- Murphy, J. J., A. Dinar, R. E. Howitt, S. J. Rassenti, V. L. Smith, and M. Weinberg. 2009. The design of water markets when instream flows have value. Journal of Environmental Management 90: 1089–1096.10.1016/j.jenvman.2008.04.001
- Prairie Provinces Water Board. 1952. Evaporation from lakes and reservoirs on the Canadian Prairies: A study based on thirty years of meteorological records. Report no. 5. Regina, SK: Prairie Provinces Water Board.
- Prairie Provinces Water Board. 1957. Precipitation and streamflow in the mountain and foothills region of the Saskatchewan River Basin. Report no. 6. Regina, SK: Prairie Provinces Water Board.
- Prairie Provinces Water Board. 1992. The 1969 Master Agreement on Apportionment and bylaws, rules and procedures. Regina, SK: Prairie Provinces Water Board.
- Thompson, J. 1990. Economic factors in river management: River conservation and management. In Flowing to the future: Proceedings of the Alberta’s Rivers Conference. University of Calgary, May 11–13, 1989, eds. C. Bradley, A. A. Einsiedel, T. Pirch, and K. Van Tighen, 51–62. Edmonton, AB: University of Alberta.
- Weber, M. 2001. Markets for water rights under environmental constraints. Journal of Environmental Economics and Management 42: 53–64.10.1006/jeem.2000.1149
- Weber, M., and M. Cutlac. 2010. Economic evaluation of water allocation strategies for the South Saskatchewan River Basin. Edmonton, AB: Alberta Environment.