Abstract
Lake Champlain and its natural outlet, the Richelieu River, underwent an historical flooding event in 2011, exceeding by a wide margin all other extreme events in over 100 years of monitoring records. In Québec, hundreds of homes were flooded for up to 2 months, requiring waves of evacuation and military involvement to support the effort. This paper examines the causes of this exceptional flood, performs a statistical analysis of important variables and compares the 2011 event to three other large floods. The results show that the return period of the 2011 spring flood exceeded 700 years and was caused by the combination of extreme precipitation over the spring season (500 years recurrence) and an important snowpack (15 years recurrence). Hydrological modeling of past extreme events over the Lake Champlain watershed indicates that liquid precipitation during snowmelt is the key factor in triggering large spring floods. The combination of the 2011 April and May precipitation with the large 2008 snowpack in a hypothetical meteorological scenario shows simulated discharges and lake levels much higher than the ones seen in 2011.
Le bassin versant du Lac Champlain et son exutoire naturel, la rivière de Richelieu, ont subi une crue exceptionnelle en 2011 qui a dépassé de loin tous les débits enregistrés depuis 100 ans. Au Québec, des centaines de maisons ont été inondées sur une période dépassant 2 mois dans certains cas, nécessitant une évacuation et l’intervention de l’armée. Ce document examine les causes de cette crue exceptionnelle, réalise une analyse statistique des variables importantes et compare la crue de 2011 avec trois autres crues majeures. Les résultats montrent que la période de retour de cette crue printanière de 2011 est supérieure à 700 ans et a été causée par la combinaison des précipitations printanières extrêmes sur la saison de printemps (récurrence de 500 ans) et une forte accumulation de neige (récurrence de 15 ans). La modélisation hydrologique des événements extrêmes passés sur le bassin versant du lac de Champlain montre que les précipitations liquides durant la fonte sont un facteur clé déclenchant les crues extrêmes. La combinaison hypothétique des précipitations d’avril et mai 2011 avec l’accumulation de neige importante de 2008 montre qu’il est possible que le lac Champlain et la rivière du Richelieu puissent atteindre un niveau et un débit bien plus élevé.
Introduction
The United Nations (UN) defines natural hazards as phenomena impacting the life of citizens, causing economic and environmental damages (UNISDR Citation2014). They can be separated into two subclasses, geophysical and meteorological hazards. Flooding is the natural hazard with the highest frequency, causing many injuries and large economic damages (Knocke and Kolivras Citation2007). Floods can be triggered in different ways. They can originate from marine submersion (e.g. the flooding in New Orleans caused by Hurricane Katrina in 2005), from flash floods (e.g. the Saguenay floods of 1996) or from spring floods (e.g. Calgary in 2013). Climate change is slowly affecting regional hydrology by changing temperature and precipitation patterns, and it is expected that flood frequency and damages will increase in the future in many regions (Milly et al. Citation2002; Hallegatte et al. Citation2013).
In the study of extreme floods, a distinction is often made between flash floods, caused by intense convective precipitation, and spring floods that result from the melting of a snowpack accumulated over several months in northern latitudes. In Nordic watersheds, most large floods happen during the spring season, when the snowpack accumulated during winter melts with the rising spring temperatures. Canada in particular has experienced a large number of extreme flooding events in the recent past. The most recent major spring flooding event happened in Alberta in 2013, where intense precipitation over the mountains resulted in a fast snowmelt and the saturation of the soil causing extreme runoff, flooding the city of Calgary (Davison and Powers Citation2013). In 2011, the Assiniboine River in Manitoba experienced record flooding due to heavy precipitation, snowmelt and high soil moisture (Environment Canada Citation2011a). In 1997, the Red River flooded a large region from Grand Forks (North Dakota, Minnesota) all the way to Winnipeg (Manitoba), causing USD 4.5 billion damages to these urban areas (Todhunter Citation2001). Spring floods are usually considered to be less life threatening than convective flash floods due to the higher duration of the flood which can dampen the peak discharge (Lawford et al. Citation1995), and because there is usually ample time to give advance warnings prior to peak discharge. On the other hand, spring floods are characterized by their large volumes and long durations and can cause serious damages which easily bear comparison with the damages induced by flash floods, as seen in the aforementioned flooding events.
Eastern Canada experienced one of its worst flooding events in history in 2011 when the Richelieu River, outlet to Lake Champlain, the sixth largest lake in North America, went above flood stage for 2 months. Lake Champlain levels shattered the previous maximum historical high, creating a flood that is a clear outlier in the 100-year historical record of Lake Chaplain levels. This record spring event flooded thousands of homes in Québec as well as in Vermont and New York on the eastern and western shores of Lake Champlain. The flood state lasted from mid-April to mid-June (Environment Canada Citation2011b) and required the evacuation of thousands of citizens. Damages were estimated at nearly USD 100 million, a very large amount for what is mostly a rural area.
Other major floods have occurred along the Richelieu River, including the 1993 and 1998 events, which were the two largest floods recorded until 2011. Both events were analyzed by Shanley and Denner (Citation1999). The maximum recorded discharge for the 2011 flood was 20% higher than both of these floods. In fact, lake levels and flow discharge exceeded those of the previous record for six consecutive weeks, which is truly outstanding considering the 100-year-long historical record of Lake Champlain levels. Saad (Citation2014) recently studied the Richelieu River and performed a sensitivity analysis of its hydrological response using various meteorological scenarios.
This event resulted in the US and Canadian governments asking the International Joint Commission (IJC) to reflect about possible structural and non-structural changes to mitigate the flood risk in the Richelieu River watershed (IJC Citation2013). This is not the first time the IJC has been asked to consider problems and solutions on this watershed. Studies in the 1930s and 1970s looked at options to regulate the Richelieu River flow. The first study opted for the construction of a dam with flood control gates. The Fryers dam was built, but the project was never finished due to other concerns during World War II. The second study once again considered regulating the lake level, but the initial project was again left unfinished due to environmental concerns about Lake Champlain. Consequently, the Richelieu River remains unregulated, since the flood gates at Fryers Dam were never installed (Brande and Lapping Citation1979; IJC Citation2013).
The aim of this study is to understand the causes of the 2011 extreme flooding event in the Champlain Lake–Richelieu River. This will be done through a comparison to other large historical flooding events over the same watershed by analyzing all available hydrometeorological data, and utilizing hydrological modeling results. A second objective of this study is to evaluate the likelihood of an even larger flood in the future, including the potential effects of climate change.
Study area
The Richelieu River watershed is located between Vermont and New York State on its eastern and western sides, respectively, and the Canadian province of Québec at its northern end (Figure ). Its main sub-watershed is the Champlain Lake basin, which drains 21,325 km2 of its 23,881 km2 total area. Thus, 90% of the Richelieu River Basin drainage area comes from the Lake Champlain watershed, which means that the majority of the Richelieu River flow comes from the lake outlet. The remaining 10% is mostly located downstream of the problematic flooding area; thus, flow discharge at the Lake outlet is an excellent approximation of the river discharge in flood-prone areas.
Figure 1. (a) Hydrography of the Richelieu River watershed and (b) daily mean, maximum and minimum annual flows at Fryers Dam on the Richelieu River.
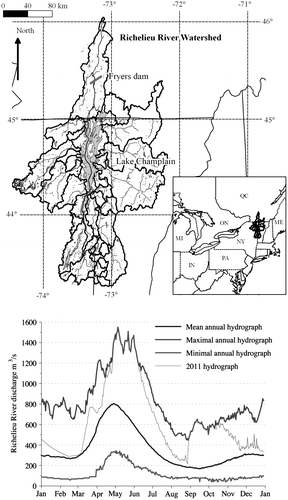
The Richelieu River watershed discharge is mainly dependent on the Lake Champlain level, with a weaker dependence on wind surge. The Lake Champlain watershed is composed of smaller sub-watersheds with surface areas varying between 115 and 2704 km2. The topography of these sub-watersheds varies greatly depending on their geographical coordinates. The Adirondacks and Green Mountains dominate the landscape on the west (maximum elevation of 1623 m) and the east (1339 m), while the northern part is a flat valley (34 m elevation at Rouses Point). To the south, the Taconic Mountains present a moderate topography. The higher elevations of the Green and Adirondack mountains allow for a significant snowpack to form in some years. Only 5.6% of the basin is occupied by developed areas, and 16% is used for agricultural purposes. The remaining areas are mostly forested land (LCPB Citation2004). More than 600,000 inhabitants occupy the US portion of the watershed (Howland et al. Citation2006), while the Canadian segment along the Richelieu River has a population of approximately 400,000 people, mostly living along the river (Hope and Bart Citation2012). The population density along the Richelieu River is high compared to the rest of the watershed, with 40% of the population within 10% of the watershed area.
Lake Champlain is a striking feature of the watershed. At 1263 km2, it occupies roughly 10% of the total surface area of the watershed. With a maximum length of 200 km in its north–south axis, it is the sixth largest lake in North America. The lake acts as a natural reservoir that damps the flood wave prior to entering the Richelieu River (Shanley and Denner Citation1999). The annual mean discharge of the Richelieu River is presented in Figure .
Dataset
River discharge data for all tributaries of Lake Champlain is from the United States Geological Survey (USGS). Flow data for the Richelieu River is from Canada’s national water survey, and for Rivière aux Brochets (a Québec affluent to Lake Champlain), the data are from the Centre d’expertise hydrique du Québec. Daily maximum and minimum temperatures and daily precipitations are from a global US gridded dataset (Maurer et al. Citation2002), improved by Livneh et al. (Citation2013). The resolution of this dataset is 1/16° and it extends from 1915 to 2011. More details can be found in Riboust and Brissette (Citationforthcoming).
The watershed mean temperature is 6°C with a mean annual precipitation of 1000 mm. Snowfall varies from 20% of annual precipitation in the valley to about 40% in the mountains (Shanley and Denner Citation1999). The mean runoff of the Richelieu River is 360 m3/s with a mean annual maximum discharge of 920 m3/s. The peak to mean average discharge ratio of 2.55 demonstrates the important role of Lake Champlain in regulating peak discharge. Peak discharge usually occurs between mid-April and early May.
Method
Hydrologic modelling of the watershed
The watershed was modelled by combining a semi-distributed hydrological model of Lake Champlain inflows with a reservoir mass conservation model (Equation 1). The outflow to the Richelieu River was modelled as an empirical function of the lake level based on historical observations (Equation 2) with a simple mass equation approach, explained with the following equations:(1)
(2)
The daily variation of water volume depends on natural inflows from all sub-watersheds Qin and direct precipitation over the lake QP, from which the daily evaporation QE (estimated by the Hamon method) and the daily volume of water outflows to the Richelieu River Qout must be subtracted. The Hamon method is a simple method for calculating daily lake evaporation that has the significant advantage of needing very few meteorological inputs. The Hamon method uses mean air temperature and daytime length as inputs.
The HSAMI (hydrological model from Hydro-Québec) was used (Fortin Citation2000) for calculating the inflows to Lake Champlain. HSAMI is Hydro-Québec’s main lumped conceptual model, which is used operationally to forecast inflows on a daily basis on more than 100 Québec watersheds (Chen et al. Citation2011). HSAMI is a 23-parameter model, of which 10 account for snow processes. Model inputs are daily minimum and maximum temperature and precipitation. Snowmelt is based on a degree-day approach. It has been used in many forecasting projects as well as to assess climate change impacts, quantify regional climate model uncertainty, analyze optimization algorithms and quantify hydrological model uncertainty (e.g. Minville et al. Citation2008; Boyer et al. Citation2010; Poulin et al. Citation2011; Arsenault et al. Citation2014). It was also used to evaluate climate change impacts on the Richelieu River watershed in a previous study (Riboust and Brissette, Citationforthcoming). For the present study, a semi-distributed approach was used in order to calibrate the lumped model on each sub-watershed using the Nash Sutcliff efficiency criterion. Lake Champlain tributaries were separated into 14 distinct gauged watersheds. The calibration was performed independently to account for physiographical differences between watersheds. For the ungauged watersheds, the flow was estimated using a space proximity regionalization approach (Arsenault and Brissette Citation2014). More details about the modeling of the hydrology of the Richelieu River can be found in Riboust and Brissette (Citationforthcoming).
Flood and frequency analysis
In order to better understand the 2011 flood, a statistical analysis was performed on all the pertinent meteorological and hydrologic data. Temperature, precipitation, natural inflows to Lake Champlain, Lake Champlain level, Richelieu River discharge and snowpack were retained for this analysis. For the snowpack, since there are no adequate observation databases going back in time, modeled snowpack was used as a proxy. The period from 1930 to 2011 was chosen for this analysis. A frequency analysis was performed on annual maximum lake level, total spring precipitation, mean spring temperature and maximum annual snowpack snow-water equivalent (SWE). The daily evolution of all important variables (i.e. lake level, river discharge, snowpack SWE, precipitation, inflows to the lake) was graphed for all four chosen flooding events.
To better outline the exceptional nature of the 2011 flood, a detailed comparison was made with the floods of 1993, 1998 and 2008. Earlier floods were not retained as there are too many differences in the available hydrometeorological data to ensure an adequate comparison. The 1993 flood was the largest on record prior to 2011. The 1993 and 1998 floods were evaluated by Shanley and Denner (Citation1999), but they did not perform any statistical analysis. The 2008 flood was retained not because of its magnitude, but rather because an exceptionally thick snowpack covered most of the watershed that year.
A frequency analysis was first performed on historical Lake Champlain levels. Lake levels were chosen over flow discharge in the Richelieu River because of their much longer record, dating back to 1907. Since river discharge is nearly perfectly correlated with lake levels (r2 = 0.97) based on the 1937–2011 daily data analysis of Riboust and Brissette (Citationforthcoming), each can be used as a proxy for the other. Wind surge, which can be rather large due to the long fetch of the lake, adds some noise to the relationship. Because of the lake’s configuration as a deep valley between the Adirondack and Green Mountains, winds come mainly from the southwest and northwest (Myer and Gruendling Citation1979). Wind surge has no impact on flood volume or duration but it can affect peak discharge into the Richelieu River, negatively or positively depending on wind direction. However, since high winds (which cause large surges) have a typical sub-daily duration (Baird and Associates Coastal Engineers Ltd. Citation2000), and considering that the analysis was performed on a daily basis, the effect of wind surge was not taken into account in this study. This is reasonable since Lake Champlain flooding stages are typically exceeded over several weeks in the case of large floods. The generalized extreme value and Pearson III distributions are often used for maximum annual lake level studies (Woodbury and Padmanabhan Citation1989; Jöhnk et al. Citation2004), but, in this case, the normal distribution was found to be best. Figure a shows the fitted distribution as well as the 95% confidence intervals. The choice of the normal distribution over the Gumbel and Pearson III distributions was confirmed using the Akaike information criterion (91 for normal, 115 for Gumbel and 92 for Pearson III distributions). The normal distribution has been shown to be adequate in the case of floods dampened by a large reservoir (Rousselle et al. Citation1990). While the normal distribution was the best, the use of either the Gumbel or Pearson III distributions had minor impacts on the results, which is not surprising considering the length of the data record. Frequency analysis was performed using Matlab programs developed in house and tested against commercial software packages. Several distributions (normal, log-normal, Gumbel, Pearson III, log-Pearson III) were tested in all cases to select the most appropriate one.
Figure 2. Frequency analysis – experimental non-exceedence probability against the best-fit distribution for (a) lake levels (normal distribution), (b) April–May total precipitation (Gumbel distribution), (c) April mean temperature (normal distribution) and (d) mean annual maximum simulated snowpack (Gumbel distribution). All values are watershed-averaged, with the exception of lake levels.
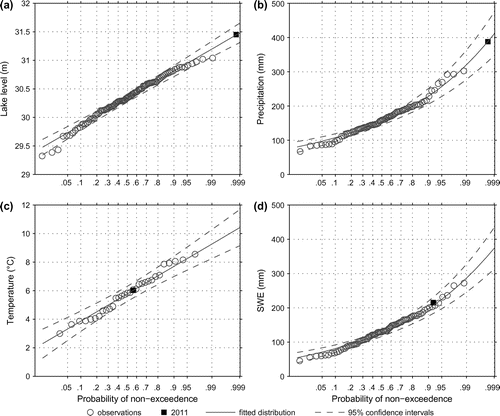
Precipitation during the flooding season was analyzed monthly between March and June, and seasonally from March to May and April to May. Frequency analyses were performed on monthly and seasonal precipitation totals. The Gumbel distribution was used with these data, as was done in many other precipitation studies (e.g. Fowler and Kilsby Citation2003). This fit and the confidence intervals are shown in Figure b. Temperatures were analyzed monthly from February to May also using a normal distribution. Results for the month of April are shown in Figure c.
Snowpack was analyzed using the annual maximum SWE averaged over the watershed. This parameter is an indicator of the water volume stored in the watershed and subsequently released during spring snowmelt. As mentioned earlier, in the absence of reliable snow data measurements, the modeled SWE from the HSAMI hydrology model was used. While there are valid reasons to question the accuracy of absolute values of SWE from the model, relative values from year to year should be reliable. The Gumbel distribution was chosen as the best distribution for SWE, as shown in Figure d, and was found to be the best compared to the other four distributions. This is consistent with other studies such as that of Graybeal and Leathers (Citation2006).
Results
Hydrology and reservoir model
The modeling of the Lake Champlain watershed yielded good results. Nash-Sutcliffe Efficiency (NSE) criterion values of 0.85 and 0.82 were obtained for daily lake levels river discharge, respectively. Figure presents the results for Lake Champlain levels. The 2011 flood peak was very well simulated, but globally, the simulated spring flood levels are slightly underestimated although flood volumes are well represented.
Flood frequency analysis
Lake level
Table presents the return period of all four flooding events for both observed and simulated lake levels using the combined hydrology-reservoir model. Modelled and observed lake levels are very close for the 2011 and 2008 floods, whereas the 1993 and 1998 floods are underestimated, as seen in Figure and Table . The exceptional nature of the 2011 flood is quite clear with 700+ and 1000+ return periods for the observed and simulated data, respectively.
Table 1. Lake levels associated with the 1993, 1998, 2008 and 2011 floods, with their corresponding returning periods.
Maximum snowpack
The maximum SWE occurred during winter 2008 with a watershed-averaged mean SWE of 261 mm. This corresponds to a 56-year return period. In comparison, the 2011 snowpack was 20% smaller, but nevertheless had an estimated 15-year return period. By itself, however, the 2011 snowpack is unlikely to be large enough to have been the sole cause of the exceptional flood. As is often the case for large floods, other compounding factors are clearly in play. The simulation results of the snow water equivalent are presented in Figure , and Table presents the average maximum SWE as simulated by the hydrology model for all four flooding events.
Table 2. Annual maximum snowpack averaged on the watershed associated with the 1993, 1998, 2008 and 2011 floods, with their corresponding return periods.
Temperature
Relevant spring and late-winter temperature information with respect to the four studied flooding events are presented in Table . All floods are mostly characterized by spring temperatures above the climatological average. May 2011, for example, was nearly 2°C higher than the average. In particular, 2008 winter and spring temperatures are much warmer than normal. High February and March temperatures may favour an earlier and faster snowmelt, which was a major component of the 1998 flood.
Table 3. Monthly mean temperatures from February to June for 1993, 1998, 2008 and 2011 floods, with their corresponding return periods.
Rainfall
The 2008 and 1998 precipitations were below the average in the spring flood season, as shown in Table , which presents monthly and seasonal liquid precipitation values for all four flooding events. Exceptional precipitations were recorded in June 1998, but this did not contribute to the spring flood since they occurred after snowmelt was finished. The seasonal precipitations in 1993 were closer to the average, but the monthly study showed that the amount of April precipitation was above the average. The 2011 spring precipitations were more than twice the average for each month. In particular, April and May rainfall amounts were very high, with a return period evaluated to be over 200 years in April and over 100 years in May. The analysis of seasonal precipitation for the months of April and May showed that the corresponding return period is greater than 500 years, with total precipitation being equal to 2.4 times the climatological average. This amount of rainfall is exceptional and it alone could explain most of the flooding.
Table 4. Monthly and seasonal liquid precipitation over the watershed for the 1993, 1998, 2008 and 2011 floods, with the corresponding return periods.
Analysis of past floods
The daily evolution of all relevant variables is presented in Figure for all four selected floods. This figure is then used in conjunction with the statistical analysis to gain a better understanding of floods in the Lake Champlain and Richelieu River watershed.
Figure 5. Graphical analysis of (a) 1993, (b) 1998, (c) 2008 and (d) 2011 spring floods, considering lake levels (O. for observed, S. for simulated), Richelieu River discharges (O. for observed, S. for simulated), lake input, precipitation and snow accumulation over the watershed.
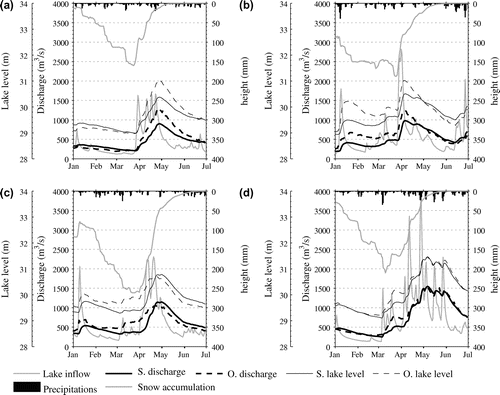
The 1998 and 1993 floods
During the 1998 event, Lake Champlain reached a level of 31 m on 5 April, the same level reached on 27 April 1993. The maximum level recorded prior to the 2011 flood was 31 m. Although they were of the same magnitude, the two floods had quite different triggering mechanisms. The snowpack over the Richelieu River watershed was much higher in 1998, but spring precipitations were much more important in 1993. This indicates that both snow cover and spring precipitation can play important roles in triggering large flooding events over this watershed. Temperatures do not seem to play a major role, which is not altogether surprising considering the size of the watershed and the long duration of a typical flood. Additional details about the 1993 and 1998 floods can be found in Shanley and Denner (Citation1999).
The 2008 flood
The 2008 flood is particularly interesting due to the thick snowpack accumulated over the watershed. The snowpack reached its maximum on 25 March with an SWE of 262 mm, which is 50 mm larger than its maximum in 2011. Shortly after reaching its 2008 peak, snowmelt started and lasted for a little more than a month. The snowmelt happened relatively quickly due to high April temperatures, which were 2.6°C higher than the climatic normal. The lake level reached 30.7 m on 22 April, corresponding to a Richelieu River peak flow of 1060 m3/s. Despite having by far the largest snowpack, lake levels this year did not reach the magnitude of the other floods because the 2008 spring precipitations were much smaller than average. April–May precipitations totaled 118 mm, considerably below the 165-mm average. The 2008 and 1998 floods share similar characteristics with respect to the rapidly disappearing snowpack, but without the additional compounding factor of high spring precipitation.
The 2011 flood
The magnitude of the 2011 spring flood broke all past records, with streamflows in the Richelieu River that reached 1550 m3/s, 20% higher than the previous record of 1260 m3/s established during the 1993 flood. Lake Champlain levels peaked at 31.5 m, a full 0.5 m higher than the preceding record. This 0.5-m difference in lake level corresponds to an additional volume of 560 million cubic meters.
Following a late December warm spell, the early January snowpack over the watershed was thin, with a little less than 30 mm (SWE). Lake levels were high at 29.7 m. From that point on, lake levels gradually decreased until the onset of the spring flood, when levels were normal for that time of the year. A few snow storms during the month of January brought the SWE to a value of 80 mm. At that time of the year, there were no precursor signals for a high spring flood.
In early March, the lake level reached its minimum level of 29.2 m, slightly higher than the average minimum lake level (28.9 m). The SWE increased rapidly in February and March, reaching 210 mm, which is a 15-year record snowpack.
On 6 March 2011, 35 mm of rain fell in 24 hours. This was followed by a few days of above-freezing temperatures, causing the SWE to fall to 165 mm by mid-March. The lake level rose to 30.1 m and stayed around that level until early April, following colder temperatures that maintained the snow cover.
From early April onwards, snow cover gradually decreased, providing Lake Champlain with continuous inflows. Snowmelt was accompanied by large rainfalls throughout April. Snowmelt provided approximately 130 mm (SWE) of water, and precipitation added another 173 mm, which is 2.5 times the average amount for April. The combination of snowmelt and heavy rainfall resulted in rapidly increasing lake levels and discharge in the Richelieu River. Lake Champlain reached the 30.5-m flood level threshold (Facey et al. Citation2012) on 14 April. This flood level was to be exceeded for the next 64 consecutive days.
At the beginning of May, less than 10% of the maximal SWE remained on the watershed. The spring flood peak occurred on 6 May following 70 mm of rainfall between 25 and 28 April, and an additional 46 mm between 3 and 5 May. In about 1 month, the lake level rose from 30 m to 31.5 m. The month of May saw 214 mm of rain fall on the watershed (2.3 times the average May precipitation). June precipitation was close to normal and the lake level finally dropped under the flood stage on 17 June.
Precipitations were truly exceptional during spring 2011, recording a total of 442 mm for March, April and May, more than twice the 198-mm average. This amounts to a 400-year return period for March to May total precipitation. This large amount, combined with a larger-than-average snowpack (15-year return period) resulted in this extreme event with a return period exceeding 700 years.
Another extreme event also happened later in 2011, when remnants of hurricane Irene reached the Lake Champlain watershed and dumped 120 mm of rainfall over 3 days, including 80 mm in a single day. The lake level went up abruptly by nearly 1 m, just below flood levels. However, severe damages were observed in many tributaries, and massive amounts of sediments were brought to the lake. In some Vermont and New York portions of the Lake Champlain watershed, damages following Irene were larger than those that occurred during the spring flood. On the other hand, damages in Québec were limited.
Discussion
Because Lake Champlain acts as a reservoir and damps the incoming flow, the typical spring flood lasts several weeks on the Richelieu River. Flood stages on the river are directly related to lake levels. The analysis performed here outlines that extreme floods on the Lake Champlain/Richelieu River may have multiple origins. The 2011 exceptional flood was triggered by the intense precipitations (500-year return period) that fell basin-wide in April and May, during the snowmelt period. The massive amount of rainfall (500-year return period) occurred on top of a relatively thick 15-year snowpack and resulted in the historical 2011 flood. In comparison, the 2008 snowpack was much larger than in 2011 (56-year return period), but the spring flood was mostly due to snowmelt with only average precipitations during the snowmelt period. The 2011 spring temperatures were only slightly above average and did not appear to have played any important role in the flooding. In comparison, 1998 and 2008 temperatures were much higher.
Theoretically, as is the case for all extreme events, it is possible for an even larger flood than 2011 to occur. To get an idea of this possibility, the 2008 snowpack (56-year period) was coupled to the 2011 spring conditions within the hydrological modelling system to create an even greater theoretical flood. In order to do so, the meteorological data from 2008 were taken until 26 March, and then from 27 March until the end of the year, the hydrological system was driven using the 2011 meteorological conditions.
The resulting snowpack and lake levels are the same as in 2008 until the end of March. From that point onwards, the main difference in lake levels and discharge in the Richelieu River stems from the additional snowmelt induced by the thicker snowpack, to which was added the 2011 precipitation. The results are shown in Figure . Although lake levels have a similar pattern to those of the 2011 flood, the amplitude is much larger, which increases the Richelieu River discharge by an additional 170 m3/s (an 11% increase) up to 1720 m3/s. This places the return period of the theoretical flood at well above 1000 years. Nevertheless, this is a relatively mild increase in peak discharge, indicating that precipitations were clearly the key factor in the 2011 flood, as outlined by the return period of individual components. The theoretical flood should also be quite clearly well below the probable maximum flood (PMF). To our knowledge, no PMF has ever been estimated over the Lake Champlain watershed, but the maximization of the probable maximum precipitation coupled with a 100-year snowpack would result in a much larger flood. As is the case with any extreme event nowadays, the media was quick to raise the spectre of climate change to explain the 2011 flood. However, the 2014 climate change impact study over the Lake Champlain watershed yielded no clear answer as to future trends in flooding probabilities. These results predicted a thinner snowpack with an increase in liquid precipitation during spring, offsetting one another with respect to flooding. All results were characterized by a large uncertainty envelope (Riboust and Brissette Citationforthcoming). However large, the 2011 flood was well within the natural variability expected in the current climate.
Figure 6. Graphical analysis of the theoretical flood created by mixing 2008 winter meteorological data with 2011 spring data.
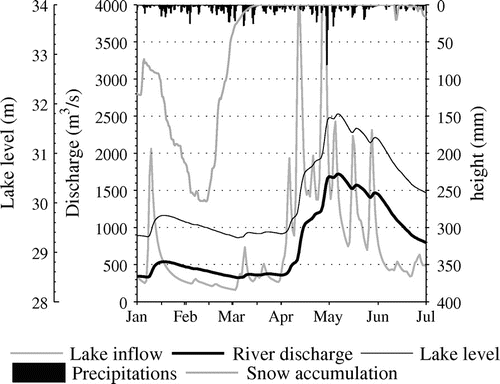
Uncertainties
As with many hydrological and earth sciences studies, this analysis is subject to multiple uncertainties. First of all, there are uncertainties concerning the hydrological modelling of the watershed. Even if the 2011 flood is well represented, the majority of simulated spring floods were underestimated when compared to the observed discharge, explaining the difference in the results of estimating the return period between observed and simulated lake levels. The use of a conceptual hydrology model may have limitations for the representation of extreme events. It would be interesting to use a physically based, fully distributed model in order to gain a better understanding of the physical processes during flooding events. To investigate the sensitivity of the semi-lumped approach, lake levels were also reconstructed using a fully lumped approach for the Lake Champlain watershed. The results were nearly identical, which indicates that sub-watersheds’ physiographical features are very similar. An investigation of modeled processes at the sub-watershed scale as done by Shanley and Denner (Citation1999) did not provide useful new information.
As usual, frequency analysis is very dependent on the length of the data record. This is the reason why lake levels were chosen rather than using the discharge data. While the length of the data record is outstanding for Lake Champlain levels (at least by North American standards), the evaluation of the return period for the 2011 event remains uncertain. Finally, the same uncertainty applies to all return period values for precipitation and temperature. The choice of the best fitting distribution is also a source of uncertainty. In particular, Lake Champlain levels were relatively difficult to fit using standard distributions. This could be due to the fact that the difference between the lowest and highest lake elevations is only about 3 m. Nonetheless, all points lie within the 90% confidence interval, and the computed return periods are fairly robust all the way to the 100-year value considering the length of the data record.
Conclusion
The aim of this work was to analyze and to better understand the leading causes that brought the 2011 catastrophic spring flood along the Richelieu River and Lake Champlain. Using a hydrological model calibrated and validated over the studied watershed, as well as a reservoir model, a graphical and a frequency analysis were performed on all relevant hydrometeorological variables. The results of these analyses showed that the 2011 flood had an estimated return period of longer than 700 years. This exceptional flood was caused by extreme precipitations over the spring season. April and May total precipitations had a return period larger than 500 years. The maximum snowpack had a rather large return period (15 years) but was nevertheless smaller than the 2008 snowpack, which was the main cause of the 2008 flooding event. For better insights into the 2011 flood, the extreme April and May weather data was combined with the 2008 snowpack, resulting in a peak flood 11% higher than in 2011, with a return period larger than 1000 years. All in all, the analysis indicates that the main factor behind the 2011 flood was the precipitation during the months of April and May. While it was the largest flood on record, the analysis also showed that it was well within the expected natural variability, and that even larger flooding events are possible, with or without climate change.
Acknowledgements
The authors want to thank Ed Maurer and Ben Livneh for providing the 2011 observed meteorological gridded dataset used in this study. They would also like to thank the USGS and Environment Canada for lake levels and Richelieu River flow datasets.
References
- Arsenault, R., and F. Brissette. 2014. Continuous streamflow prediction in ungauged basins: The effects of equifinality and parameter set selection on uncertainty in regionalization approaches. Water Resources Research 50: 6135–6153. doi:10.1002/2013WR014898.
- Arsenault, R., A. Poulin, P. Côté, and F. Brissette. 2014. Comparison of stochastic optimization algorithms in hydrological model calibration. Journal of Hydrologic Engineering 19: 1374–1384.
- W.F. Baird and Associates Coastal Engineers Ltd. 2000. Review of Lake Winnipeg water level reporting procedures. Prepared for Lake Winnipeg Shoreline Erosion Advisory Group, January 2000, Ottawa, 85 pp.
- Boyer, C., D. Chaumont, I. Chartier, and A. G. Roy. 2010. Impact of climate change on the hydrology of St. Lawrence tributaries. Journal of Hydrology 384(1–2): 65–83. http://linkinghub.elsevier.com/retrieve/pii/S002216941000034X.
- Brande, J., and M. Lapping. 1979. Exchanging information across boundaries: The Richelieu–Champlain experience. Canadian Water Resources Journal 4(4): 39–50. http://dx.doi.org/10.4296/cwrj0404039.
- Chen, J., F. P. Brissette, and R. Leconte. 2011. Uncertainty of downscaling method in quantifying the impact of climate change on hydrology. Journal of Hydrology 401(3–4): 190–202. http://linkinghub.elsevier.com/retrieve/pii/S0022169411001351.
- Davison, J., and L. Powers. 2013. Why Alberta’s floods hit so hard and fast. CBC News. http://www.cbc.ca/news/canada/calgary/why-alberta-s-floods-hit-so-hard-and-fast-1.1328991 (accessed April, 2014).
- Environment Canada. 2011a. Canada’s top ten weather stories for 2011: Historic flood fights in the west. http://ec.gc.ca/meteo-weather/default.asp?lang=En&n=0397DE72-1 (accessed April, 2014).
- Environment Canada. 2011b. Canada’s top ten weather stories for 2011: Richelieu Flooding…Quebec’s longest-lived disaster. http://www.ec.gc.ca/meteo-weather/default.asp?lang=En&n=D7CF8BE3-1 (accessed April, 2014).
- Facey, D. E., J. E. Marsden, T. B. Mihuc, and E. A. Howe. 2012. Lake Champlain 2010: A summary of recent research and monitoring initiatives. Journal of Great Lakes Research 38: 1–5.
- Fortin, V. 2000. Le modèle Météo-Apport HSAMI: Historique, théorie et application. Institut de recherche d’Hydro-Québec.
- Fowler, H. J., and C. G. Kilsby. 2003. A regional frequency analysis of United Kingdom extreme rainfall from 1961 to 2000. International Journal of Climatology 23(11): 1313–1334. http://doi.wiley.com/10.1002/joc.943.
- Graybeal, D., and D. Leathers. 2006. Snowmelt-related flood risk in Appalachia: First estimates from a historical snow climatology. Journal of Applied Meteorology & Climatology 45: 178–193. http://journals.ametsoc.org/doi/abs/10.1175/JAM2330.1.
- Hallegatte, S., C. Green, R. J. Nicholls, and J. Corfee-Morlot. 2013. Future flood losses in major coastal cities. Nature Climate Change 3(9): 802–806. http://www.nature.com/doifinder/10.1038/nclimate1979.
- Hope, A., and R. Bart. 2012. Evaluation of a regionalization approach for daily flow duration curves in central and southern California watersheds. JAWRA Journal of the American Water Resources Association 48(1): 123–133.
- Howland, W. G., B. Gruessner, M. Lescaze, and M. Stickney. 2006. Lake Champlain experience and lessons learned brief. Report from project Towards a lake basin management initiative and a contribution to the Third World Water Forum: Sharing experiences and early lessons in GEF and non-GEF lake basin management projects. http://www.ais.unwater.org/ais/aiscm/getprojectdoc.php?docid=1088
- International Joint Comission (IJC). 2013. The identification of measures to mitigate flooding and the impacts of flooding of Lake Champlain and Richelieu River. http://www.ijc.org/files/publications/Final_PoS_LakeChamplain-RichelieuRiver.pdf
- Jöhnk, K. D., D. Straile, and W. Ostendorp. 2004. Water level variability and trends in Lake Constance in the light of the 1999 centennial flood. Limnologica 21: 15–21.
- Knocke, E. T., and K. N. Kolivras. 2007. Flash flood awareness in southwest Virginia. Risk Analysis: An Official Publication of the Society for Risk Analysis 27: 155–169.
- Lake Champlain Basin Program (LCBP). 2004. The Lake Champlain Basin atlas: Online version. http://atlas.lcbp.org/HTML/intro.htm (accessed August, 2014).
- Lawford, R.G., T. D. Prowse, W. D. Hogg, A. A. Warkentin and P. J. Pilon. 1995. Hydrometeorological aspects of flood hazards in Canada. Atmosphere–Ocean 33(2): 303–328. http://www.tandfonline.com/doi/abs/10.1080/07055900.1995.9649535.
- Livneh, B., E. A. Rosenberg, C. Lin, B. Nijssen, V. Mishra, K. M. Andreadis, E. P. Maurer, and D. P. Lettenmaier. 2013. A long-term hydrologically based dataset of land surface fluxes and states for the conterminous United States: Update and extensions*. Journal of Climate 26(23): 9384–9392. http://dx.doi.org/10.1175/JCLI-D-12-00508.1.
- Maurer, E. P., A. W. Wood, J. C. Adam, D. P. Lettenmaier, and B. Nijssen. 2002. A long-term hydrologically based dataset of land surface fluxes and states for the conterminous United States*. Journal of Climate 15(22): 3237–3251. http://dx.doi.org/10.1175/1520-0442(2002)015<3237:ALTHBD>2.0.CO;2.
- Milly, P. C. D., R. T. Wetherald, K. A. Dunne, and T. L. Delworth. 2002. Increasing risk of great floods in a changing climate. Nature 415(6871): 514–517. http://www.ncbi.nlm.nih.gov/pubmed/11823857.
- Minville, M., F. Brissette, and R. Leconte. 2008. Uncertainty of the impact of climate change on the hydrology of a Nordic watershed. Journal of Hydrology 358(1–2): 70–83.
- Myer, G. E., and Gruendling, G. K. 1979. Limnology of Lake Champlain. New England River Basins Commission: Lake Champlain Basin Study.
- Poulin, A., F. Brissette, R. Leconte, R. Arsenault, and J.-S. Malo. 2011. Uncertainty of hydrological modelling in climate change impact studies in a Canadian, snow-dominated river basin. Journal of Hydrology 409(3–4): 626–636. http://linkinghub.elsevier.com/retrieve/pii/S0022169411006135.
- Riboust, P., and F. Brissette. Forthcoming. Climate change impacts and uncertainties on spring flooding of Lake Champlain and the Richelieu River. Journal of the American Water Resources Association, under review.
- Rousselle, J., W. E. Watt, K. W. Lathem, C. R. Neill, and T. L. Richards. 1990. Hydrologie des crues au Canada: Guide de planification et de conception. Ottawa: Conseil National de Recherches Canada et Comité Associé D’hydrologie.
- Saad, C. 2014. Analyse hydrométéorologique multivariée et modélisation déterministe des crues de la rivière Richelieu, Québec. M.Sc. thesis, Institut National de la Recherche Scientifique – Centre Eau Terre Environnement, Quebec City, Canada, 133 pp.
- Shanley, J. B., and J. C. Denner. 1999. The hydrology of the Lake Champlain Basin. In Lake Champlain in transition: From research toward restoration, 1: 41–66. Washington, DC: AGU. http://dx.doi.org/10.1029/WS001p0041.
- Todhunter, P. 2001. A hydroclimatological analysis of the Red River of the North snowmelt flood catastrophe of 1991. JAWRA: Journal of the American Water Resources Association 37(5). http://onlinelibrary.wiley.com/doi/10.1111/j.1752-1688.2001.tb03637.x/abstract.
- United Nations Office for Disaster Risk Reduction (UNISDR). 2014. Terminology on disater risk reduction. http://www.unisdr.org/we/inform/terminology (accessed April, 2014).
- Woodbury, L., and G. Padmanabhan. 1989. Estimating terminal lake level frequencies. Journal of Water Resources Planning and Management 115(3): 321–337. http://ascelibrary.org/doi/abs/10.1061/(ASCE)0733-9496(1989)115:3(321).