Abstract
Serpentinitic sediments rich in chrysotile asbestos, from a natural landslide in the Sumas River Watershed in Washington State, are deposited on agricultural land during flooding events, creating a health concern for the rural population. The sediments have a chemical composition of high magnesium (Mg), nickel (Ni) and chromium (Cr) and high pH values compared with non-serpentinitic sediments that originate from the agricultural lowland. As the sediments are transported from the headwater to the mouth, the effects of mixing with non-serpentinitic water from agricultural drainage decrease the pH, increase the contents of organic matter, nitrate and zinc (Zn), and impact the sediments that originated from the landslide. A comparison of bed and suspended sediment showed that there is a clear seasonal effect with lower Mg and Ni values during low flow for both types of sediments. Bed sediments were consistently higher than suspended sediment in Mg and Ni. Sediments emerging from the landslide have positive zeta potentials but disperse very rapidly and become negatively charged. Once the sediment interacts with agricultural sources downstream the zeta potential becomes more negative, causing the sediment to stay suspended. It is postulated that at high pH, Mg is leached from the brucite layer of chrysotile and precipitates as Mg carbonate, which enriches the bed sediments in Mg and associated trace metal concentration.
Des sédiments serpentiniques riches en amiante chrysolite, libérés par un glissement de terrain dans le bassin versant de la rivière Sumas dans l’état de Washington, se sont déposés sur des terres agricoles suite à une inondation, prévoquant de ce fait une préoccupation de santé public. Ces sédiments sont riches en amiante et possède une concentration élevée en magnésium (Mg), nickel (Ni) et chrome (Cr) de même qu’un pH élevé comparé aux sédiments non serpentiniques de la vallée agricole. Alors que ces sédiments sont transportés des cours d’eau supérieurs vers l’embouchure, le mélange avec l’eau non serpentinique ruisselant de la zone agricole provoque une baisse du pH, augmente les concentrations en matières organiques, en azote et en zinc (Zn) et modifie le caractère des sédiments provenant du glissement de terrain. Une analyse comparative des sédiments de fond et des sédiments en suspension démontre un effet saisonnier évident car leurs concentrations en Mg et Ni diminuent durant les périodes d’étiages, pour chaque type de sédiment. Les concentrations en Mg et Ni sont systématiquement plus élevées dans les sédiments de fond par rapport aux sédiments en suspension. Les sédiments du glissement de terrain ont un potentiel zêta positif et se disperse très rapidement. Toutefois, le potentiel zêta devient négatif et les sédiments restent en suspension plus longtemps dans les zones agricoles situées en aval. L’hypothèse dégagée est que le Mg est lessivé du brucite enrobant le chrysolite, pour ensuite précipiter en carbonate de magnésium, ce qui enrichie les sédiments de fond en Mg et augmente la concentration des métaux-traces associés.
Introduction
The Sumas Mountain landslide at the headwaters of Swift Creek (48°54’54”N, 122°16’07”W) releases up to 120,000 cubic yards of excess sediment into Swift Creek each year. The slide material contains deposits of naturally occurring asbestos. Swift Creek flows into the Sumas River near Nooksack in Whatcom County, Washington, and enters into Canada near Abbotsford, BC (United States Environmental Protection Agency [USEPA] Ecology and Environment Inc. Citation2007). Mass wasting and weathering of the bedrock has resulted in serpentine-enriched sediment release (Bayer and Linneman Citation2011), and during major storm events these asbestos-rich sediments are deposited on agricultural land in the Sumas River floodplain. When dry, these sediments are prone to wind erosion, can be inhaled into the lungs and increase the risk of developing asbestos-related diseases.
The origin of the serpentine-rich sediment in Swift Creek is a large, complex landslide in the upper watershed of Sumas Mountain that became reactivated in the 1940s. Schreier and Taylor (Citation1980) report that the exposed slide material contains naturally occurring asbestos and associated metals, notably nickel (Ni), manganese (Mn), chromium (Cr) and magnesium (Mg). X-ray diffraction (XRD) analysis of the serpentine sediment detected a mix of chrysotile and lizardite derived from the landslide of altered serpentinite. Lizardite and chrysotile (magnesium silicate hydroxides) comprise two of the three more common members of the serpentine group (Januch et al. Citation2006). Other minerals reported to occurr in variable amounts include chlorite, mica, quartz, feldspar, pyroxene, amphibole, calcite, heulandite–zeolite, and chromite (USEPA Ecology and Environment Inc. Citation2006).
An on-site survey of surface soil samples on adjacent floodplains revealed asbestos in concentrations ranging from 0.1 to 4.4% (USEPA Ecology and Environment Inc. Citation2006). There were elevated levels of chrysotile fibers in air filter samples, as well as minor amounts of amphibole fibers (actinolite or tremolite) in some of the samples (United States Department of Health Citation2006; USEPA 2006). The amount and dimensions of the asbestos fibers in the Sumas system were previously reported by Schreier (Citation1987).
Thus, the sediments are of significant health concern to the rural population, and reclamation of the sediments remains challenging. Removal and transport could result in airborne exposure of the workers, finding appropriate sites for deposition of the sediment is difficult and it is very unlikely that the landslide can be stabilized in the short run. The Swift Creek headwater area is on steeply sloping forested terrain, while the lowland in Washington State and British Columbia is intensively used for agriculture, primarily dairy and poultry production. The landslide produces a fine-textured serpentinitic asbestos-rich sediment that has a high pH, and high concentrations of Mg, Ni and Cr (Smith et al. Citation2007; Schreier and Lavkulich Citation2010). Once the sediment is transported into the lowland and influenced by agricultural inputs, the quality and physical behavior of the sediment is altered, making quantification of the sediment load challenging.
It is estimated that the total sediment load of Swift Creek is around 18,530 t/km2/yr, of which approximately 910 t/km2/yr is suspended sediment (Bayer and Linneman Citation2011). These authors report suspended sediment concentrations varying between 5 and 40 g/L. Photographs of the water in Swift Creek during high flow show a whitish-gray color of the sediment-laden stream.
Sediments in a water body can contribute to higher concentrations of bacteria, nutrients and metals in the water. These pollutants attach to sediment particles and are carried into water bodies with storm water. A complicating factor in determining suspended load is the actual particle size and density. It is commonly assumed that suspended particles are individual mineral particles, and not aggregates of smaller particles (silt and clay) that behave like larger aggregates and thus are not estimated precisely by sediment analysis. This is particularly a concern when streams have a high pH and soluble salt content, such as in the Swift Creek/Sumas River.
Along the river reach, from the landslide to the BC border, a number of factors affect the stream water quality, including dilution of the sediment by non-serpentine-affected tributary streams, and land use impacts, notably agriculture. These influences alter the water quality and undoubtedly the flocculation and dispersion characteristics of the sediment. Schreier and Lavkulich (Citation2010) report that the serpentine sediments which enter the Sumas River in British Columbia via the Swift Creek drainage have a pH above 8 at the landslide and have an electrical conductivity of around 140–270 μS/cm. The pH drops to near neutral once the stream enters BC at the USA–Canada border. The sediment near the origin of Swift Creek settles rapidly as a result of the high electrical conductivity and the concentration of basic cations in the stream water, notably Mg.
What are the major factors that influence sediment distribution within a flowing stream? One major factor is the process of coagulation, which makes colloidal particles behave like much larger particles. Coagulation is the process by which very fine solid suspensions (colloidal particles) are destabilized so that they begin to agglomerate. The charge on colloids may be positive or negative, and most colloidal particles have a negative charge. Colloidal dispersions are thermodynamically unstable. If the charge layer is removed or chemically altered, particles tend to agglomerate spontaneously. If a colloidal particle is electrically charged, it attracts oppositely charged ions and other colloidal particles. The particle and the attached ions of opposite sign form an electric double layer (Katsuhiko and Fujitani Citation2005).
A measure of the electrical attraction/repulsion among suspended sediments is the zeta potential. The zeta potential is defined as the electric potential difference between the shear planes of a colloidal particle and the bulk of the solution. The zeta potential is an indirect measure of the electrical charge of the colloidal particle and may be experimentally measured (using a microscope) by determining the velocity of a particle moving under an electric potential of known intensity (Salopek et al. Citation1992; Droste Citation1997). If the repulsive forces produced by the double layer are high, the colloidal particles are unable to agglomerate and hence coagulate. The effects of the double layer can be neutralized by increasing the ionic strength of the solution or reacting the surface with ions of opposite charge.
Historical evidence indicates that the metal content of the sediment along the length of the stream is advancing from the headwaters to the mouth of the Sumas (Smith et al. Citation2007; Schreier and Lavkulich Citation2010). It is postulated that, as a result of the unique charge characteristics of the serpentinic sediments, metal-containing sediments are moving in a “pulsed” manner along the reach in relation to stream discharge dynamics.
The objectives of this investigation were to: (1) determine the characteristics of the sediments in the water column and along the stream length; (2) determine flocculation/dispersion factors from the headwaters downstream to the USA–Canada border by the zeta potential index; and (3) examine the factors affecting the temporal sediment transport dynamics within the watershed.
Materials
Description of study site
Figure shows the study area and the sampling stations from the natural landslide in Whatcom County, Washington State, to the mouth of the Sumas drainage in British Columbia. Suspended and deposited sediment samples were collected along the length of the stream. Bed sediments were collected at several stations from the headwater to the mouth of the river in the mid 1990s, and during the largest storm event in December–January 2008–2009, to determine the overall sediment and water quality and to document changes over space. In 2011 a more intensive and detailed study was initiated to determine the spatial and temporal changes of bed sediments, suspended sediments and water quality, followed by additional zeta-potential analysis in 2014.
Streamflow regime
The Sumas River is a rain-fed stream that peaks during the December-to-February periods and reaches low flow conditions in late summer. The analysis of the discharge data from the Environment Canada station (08MH029) at the international border showed that there has been a significant increase in the peak flow event since 1953. What is of particular interest is the frequency of storm events above 15 m3/sec, which result in flooding of lowland areas. As shown in Figure , there has been a steady increase in such events on a decadal scale, and this indicates that the sediment transport and flooding problem is increasing. The largest flooding event occurred in January 2009 with a peak discharge of 57 m3/sec, and as documented by Schreier and Lavkulich (Citation2010), this was a classic rain-on-snow event that inundated large areas of agricultural land on both sides of the border.
Methods
Bed- and suspended sediment analysis
Bed sediments were collected in 1994, 2008, 2009, 2010, 2011, 2012 and 2014 by carefully scraping the top 2–5 cm layers at the surface in pool areas of the river by a trowel. During the intensive sampling period in 2011, bed sediments were collected at the same time as the suspended sediments.
Suspended sediment samples were collected along the Sumas River (Figure ) using a time-integrated suspended sediment sampler (Phillips et al. Citation2000). Eight sets of suspended and bed sediments were collected in 2011 at three stations (Landslide, International Border, Hougan Park) to determine the changes over time and space in the watershed. One additional set of sediments was collected in November to determine the short-distance change near the landslide from the landslide to the United Stated Geological Survey (USGS) station at South Pass Road. The length of collecting the time-integrated suspended sediment samples and the discharge characteristics during the sample collection are summarized in Table . The contents of the samplers were emptied into 4-L acid-washed plastic bottles, rinsed in river water before collection and transported to the laboratory on ice in a cooler.
Table 1. Characteristic duration and discharge during suspended sediment collection.
In the laboratory, the sediment samples were wet-sieved through stainless steel sieves to obtain a < 63 μm fraction (silt and clay particles). This size range represents the fraction of sediment that is transported in suspension, and contains the largest concentration of asbestos fibers. The sieved sediment was placed in acid-washed beakers and oven dried at 70°C overnight. An agate mortar and pestle was used to break apart large aggregates and the samples were stored in sealed labeled plastic containers. The sediments were digested and analyzed for trace metals using an inductively coupled argon emission spectrograph (Varian, Vistapro, Varian 725-ES). Samples were digested using the aqua regia method USEPA Ecology and Environment Inc. Citation1994; Chen and Ma Citation2001).
Water samples
Water samples were collected in acid-washed 250-mL plastic bottles which were thoroughly rinsed with stream water prior to taking the samples. The samples were stored on ice in a cooler during transportation to the laboratory. Temperature and specific conductivity were measured in situ using a YSI 30 conductivity meter. In the laboratory, pH was measured within 12 hours of sample collection using the Orion model 420A pH meter (Thermo Scientific, Waltham, MA). All samples were measured 3–5 times and averaged for a representative reading. The samples were filtered using Whatman #42 filter paper within 12 hours of sample collections. These filtered samples were analyzed using inductively coupled plasma – atomic emission spectroscopy (ICP-AES-Varian725-ES). Nitrogen was determined by Kjeldahl (McGill and Figueriredo 1993). Results are means of three separate analyses of each sample.
Surface charges
Surface charge was measured using a Zeta Meter Model 3.0+ (Zeta Meter Inc., USA) in Mining Engineering at the University of British Columbia. Fifty milligrams of each sample was transferred into 15 mL of distilled water. All the measurements were carried out within 30 seconds of being shaken. The applied voltage was determined based on the specific conductivity of the solution. The particle movement was observed through a microscope and the time taken for a particle to travel the distance of a micrometer was measured. To minimize error, a minimum of five particles were tracked and their average time was calculated.
Results
Water and sediment chemistry
The asbestos-rich sediments that originate from the landslide contain high Mg and Ni values, and have alkaline pH and low calcium (Ca) and zinc (Zn) values. As the water and sediments move in the downstream direction the pH declines to neutral and specific conductivity and total nitrogen in sediment increases as a result of weathering and organic-rich input from the surrounding agricultural activities. Figure shows the spatial and seasonal changes in pH and specific conductivity in the water from the headwater to the mouth. Similarly, Figure illustrates the spatial changes in total nitrogen content in bed sediment in the downstream direction, which shows the impact of sediments from agriculture. All water and sediment samples were collected between November and December 2011.
Figure 3. Spatial and seasonal differences in pH in water from the headwater landslide downstream in the Sumas River in 2011.
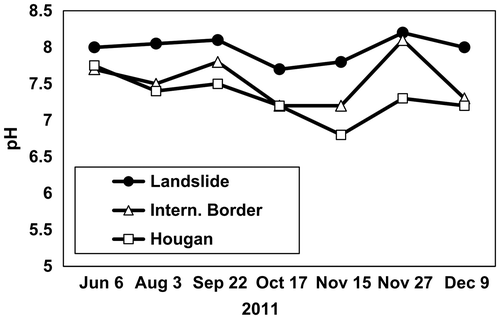
The differences in chemical characteristics of the sediments from the landslide versus those from lowland agricultural sources provide a good indication of how the sediments change as they move through the watershed. Figure shows the changes in Mg and Ca concentrations and the Ni and Zn concentrations in the < 63 μm fraction of bed sediments in December 2011, from upstream to downstream. The landslide sediments are high in Mg and Ni but low in Ca and Zn, while the sediment from agricultural sources is high in Ca and Zn, which is attributed to the presence of these metals used extensively in soil amendment and livestock feed, and which enter the stream via erosion after manure application (Smith et al. Citation2007).
Figure 5. (a) Magnesium (Mg) and calcium (Ca) values in bed sediments and (b) nickel (Ni) and zinc (Zn) values from the upstream headwaters to the mouth of the Sumas Watershed. The landslide sediments are dominated by Mg and Ni and have very low Ca values, while agricultural sediments have high Ca and Zn and low Mg and Ni values.
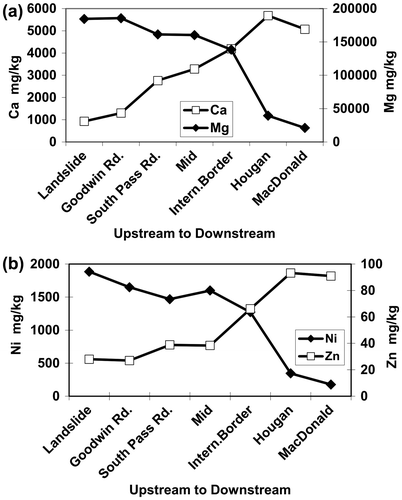
The data suggest that sediment transport occurs primarily during storm events, and as a result there are significant seasonal changes in the chemistry of the sediment. Since the sediment source at the landslide is dominated by asbestos-rich serpentinitic material, Mg and Ni concentrations are expected to be significantly higher than at the downstream stations. Comparing the seasonal amount of Mg and Ni (Figure ) at the landslide and at the international border (midpoint in the watershed and in the heart of the agricultural area) shows that both suspended and bed sediments have a significant seasonal pattern, with lower values in the summer and higher values during the high discharge periods in the winter. Of particular interest is the fact that the bed sediment samples were consistently higher in these elements than the suspended sediment samples, which suggests in situ enrichment. The difference between bed and suspended sediment is greatest in the summer low-flow period, when the landslide contribution is the lowest. There is also a roughly 20% lower concentration of Ni and Mg at the border than at the landslide. In contrast, Ca, which is not present in very high concentrations in the landslide sediments, shows a significant increase downstream, which supports the observation and provides further evidence of agricultural impacts.
Settling rates of sediments
The < 63 μm sediment fraction appears to settle more rapidly near the landslide area of Swift Creek than downstream. Upon settling, a whitish-colored asbestos-rich film is precipitated on the bed sediment after a storm event. The suspended sediment samples that were collected over a 12-day period in November 2011 at 400 m, 1200 m and 2900 m below the landslide in Swift Creek ranged from no agricultural input at the uppermost station to increasing agricultural impacts downstream at the lower sampling stations. As shown in Figure , these changes in stream chemistry have a profound influence on the settling rate of the fine sediments. The < 63 μm sediment fraction, at the lowest station, remains almost completely suspended after 5 minutes in the water column, while a very large proportion of the headwater sediment has settled. This is surprising because asbestos fibers which dominate the fine sediment fraction would be expected to settle at a slower rate than the more non-fibrous mineral particles. Alvarez-Silva et al. (Citation2010) report that serpentine minerals remain suspended over a pH range of 4–8, in contrast to non-serpentine minerals, as a result of their high hydration potential.
Figure 7. Differences in settling rates of suspended sediments (< 63μm fraction) between the landslide at the Swift Creek/Sumas river confluence. Mg: magnesium; Ni: nickel.
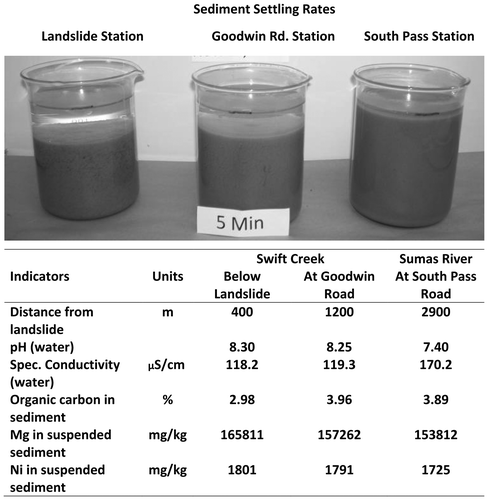
Flocculation and coagulation is a precursor to the settlement and transition of suspended sediment to bed sediment. As stated earlier, surface charge of the sediment and the chemical characteristics of the stream, particularly the ionic strength and pH, are controlling factors for sediment settling. Table presents the results of pH in stream water at the headwaters of Swift Creek and at two sampling stations downstream, as well as the surface charge of suspended and bed sediments. All samples were collected between 26 May and 26 November 2011 and an additional set was collected in March 2014.
Table 2. Changes in water pH and sediment zeta potential (mV) upstream to downstream in the Sumas River, and zeta potential of two reference minerals.
As shown in Table , the pH of the water associated with the suspended sediments is near or above 8. Of particular interest are the zeta potential values. In the headwater region the fresh suspended sediments have mainly positive zeta potential values, while the bed sediments have negative charges. All suspended and bed sediment charges below the headwater station are negative. The comparison of two standard minerals, kaolinite and chrysotile, shows that the clay mineral kaolinite is negatively charged, while chrysotile has a positive charge (Lavkulich et al. Citation2014). It is commonly held that zeta potential values greater than +10 result in dispersion (Ofir et al. Citation2007). Once the zeta potential reaches 0 to –10, flocculation and coagulation should occur, but as shown in Figure , this is not the case. The fresh sediment has a positive zeta potential but rapidly changes as the sediment adjusts to the freshwater environment, and then decreases and becomes negative in value as the sediment reacts with the dissolved cations (Mg) and dissolved carbon dioxide (CO2) in the stream. Below the landslide the pH decreases, but the flocculation and coagulation are delayed and it is assumed that the negatively charged organic matter and nitrate in the water from agricultural sources interact with the negatively charged sediments, and this might be the cause of the sediment dispersion in the lowland. The question remains: what agent in the aquatic system at the landslide causes the net negative charge (anion) to neutralize the positive charges on the chrysotile-rich sediment?
At the landslide source sediment settles rapidly but as the sediments come into contact with the stream water, the zeta potential changes from positive to negative, and during this transition sediments settle rapidly. Magnesium decreases in the sediment but increases in the water column immediately below the landslide, probably the result of the reaction of Mg with the bicarbonate (HCO3–) dissolved in the high-pH waters. Holmes et al. (Citation2012) reported similar processes in suspended sediment at the headwaters of Swift Creek. X-ray diffraction did not show Mg carbonate in the suspended sediment but bed sediment settled on the side of the streambed fizzed when hydrochloric acid (HCl) was applied. This confirms that Mg precipitation occurs. Thus, the dynamics of zeta potential is highly complex and the interactions between different water sources, and the interactions with stream velocity, need to be assessed further in order to get a more distinctive appreciation of the dynamics of sediment transport in these systems.
The seasonal differences in the Mg content in the streamwater at the landslide and at the international border are shown in Figure . The highest Mg concentrations in water at the landslide occur during late summer while, in contrast, at the international border the opposite pattern is observed. The Mg content in the water increases in the three stations below the landslide, but then gets diluted by additional water from the lowland area. This provides additional evidence of Mg leaching from the brucite layer when asbestos-rich sediment enters the upper part of the stream.
Figure 8. (a) Spatial differences in magnesium (Mg) in streamwater in the downstream direction and (b) seasonal differences in nickel (Ni) at the landslide and at the international border during 2011.
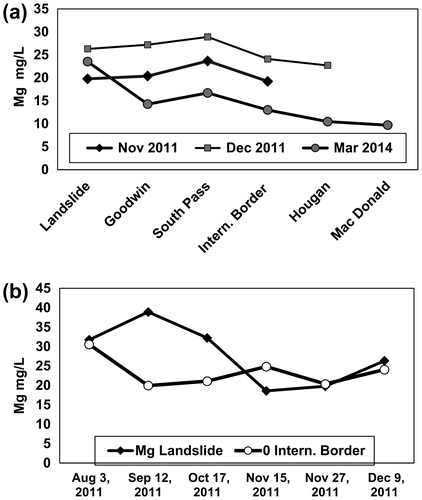
These findings are in agreement with results reported by Seshan (Citation1983), who states that the surface properties of chrysotile asbestos are affected by acids and exposure to water, and in particular the surface potential (zeta potential) changes from positive to negative. The explanation given is that the surface of the chrysotile becomes more “silica-like” as the Mg is lost from the surface. The changes in the surface chemistry of chrysotile have been documented in laboratory studies by Lavkulich et al. (Citation2014), who showed that as Mg is leached from the surface, the silicon (Si) structure is exposed and becomes negatively charged. It is the excess Mg at the surface that is the cause of the initial positive zeta potential. Thus as the chrysotile-rich serpentine sediment from the landslide enters the aquatic environment, the surfaces react, Mg is leached from the surface and the zeta potential becomes negative, thus allowing for rapid flocculation, coagulation and deposition of the sediment. Once the sediment reaches the high-organic and nitrogen-rich aquatic environment in the agricultural lowland, the sediment remains suspended for considerable time.
Evidence of pulsing effect in sediment transport
As a result of the differences in settling rates, there is strong evidence that the suspended sediment does not move through the entire river system during a single storm event but rather moves in a pulsed manner of suspension and re-deposition. In 2009, the river at the international border reached the highest measured discharge level since 1953 of 57 m3/sec. We collected a bed sediment sample in December before the peak of the storm, in February after storm recession and again in June 2009. To this we added summer bed sediment data for 1994, 2008, 2010 and 2011, which were re-analyzed with the same analytical techniques. The results (Figure ) show a clear pulsing effect during the 2009 storm event. The pulsing is posited to be flow related due to the charge changes in the headwaters, suggesting that the sediment settles and is re-suspended during subsequent storms.
Figure 9. Evidence of pulsed movement in sediments with a focus on (a) magnesium (Mg) and (b) nickel (Ni) in bed sediments before and after the largest storm event in 52 years during the winter of 2008–2009.
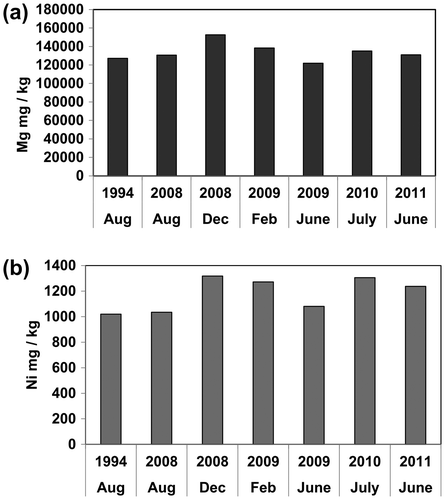
The December 2008 sample was taken at the beginning of the storm event, which peaked at 57 m3/sec on 9 January 2009. All other bed sediments were collected during the summer period after the high winter discharge.
The winter period which preceded the 1994 sediment sampling had the lowest mean monthly discharge and the lowest maximum storm peak (12.5 m3/sec) recorded. Similarly, the winter peak event before the 2008 sampling period had a moderate mean monthly discharge and a low maximum peak flow of 16.3 m3/sec. In contrast, the 2009 summer samples in 2009, 2010 and 2011 had high monthly discharge and maximum peak flows of 57 m3/sec, 40 m3/sec and 31 m3/sec, respectively. The annual discharge events over these 5 different years are provided in Figure , which shows that the 2008–2009 and 2010–2011 discharge events produced the highest winter discharge, resulting in higher Mg and Ni values in sediment at the international border station.
Discussion and conclusion
The asbestos-rich sediments from the Swift Creek landslide exhibit very challenging behavior because they settle rapidly immediately below the landslide area and leave a white film of asbestos-rich fibers at the surface. As the sediment is transported downstream it is impacted by the chemistry of the water and sediments from agricultural sources, which have higher electrical conductivity, lower pH and higher organic matter and nitrogen content. Since suspended sediments at the headwaters of Swift Creek rapidly change from positive to negative zeta potential, they settle rapidly and it is suggested that the reason for this change is that the Mg-rich outer layer of the chrysotile fibers is leached in the aquatic system. This charge relationship becomes more dynamic as Mg carbonates are formed and as tributary organic-rich water enters the stream. These interactions are likely responsible for the pulsed manner of flocculation and re-suspension. As the negatively charged suspended sediment moves downstream, the pH drops and the more negatively charged organic matter and nitrate likely interact with the negatively charged sediments to result in re-suspension. This partially explains the unusual changes in the settling rates of these sediments.
It is postulated that at high pH, Mg from the brucite layer of chrysotile is leached and is precipitated as Mg carbonate, which enriches the bed sediments that were found to be higher in Mg than the suspended sediment throughout the stream system. There is also a distinct seasonal pattern of Mg in the water, bed and suspended sediment. At the international border, the water and the sediment samples had lower values in late summer, during low streamflow conditions. However, at the landslide source, the Mg content in the water reaches the highest values during the summer. It is suggested that during low summer flow, bed sediments near the landslide undergo alternating aerobic and anaerobic conditions that could accelerate reaction with dissolved bicarbonate ions.
There is convincing evidence that Mg and Ni in the sediments can be used as tracers for the origin of asbestos-rich source material. Both elements exhibit a steady decline in concentration in the downstream direction. In contrast, Ca and Zn, which are low in the landslide material, can be used as tracers for the sediments originating from the agricultural sediments. Both show increasing values in the downstream direction.
Finally, there is evidence that the landslide sediments move through the watershed in a pulsed manner of suspension, deposition and re-suspension during storm events. The largest storm event generated a significant short-term increase in Ni and Mg values in bed sediments before and after these storm events. There is further evidence to suggest that the proportion of the sediment that originated from the landslide has increased at the international border region over the past 18 years, but the pulsing pattern of sediment transport makes such assessment challenging.
A historic assessment of peak flow at the international border shows that high storm events with high discharge show an increasing trend since 1953, which suggests that the problems associated with sediment deposition on lowland agricultural sites are increasing, particularly since it appears unlikely that the landslide can be stabilized in the near future.
Acknowledgements
The zero-point charge analysis was conducted in the Mining and Mineral Processing Laboratory at UBC by Beini Xu.
References
- Alvarez-Silva, M. M., A. Mirnezami, A. Uribe-Salas, and J. A. Finch. 2010. Point of zero charge, isoelectric point and aggregation of phyllosilicate minerals. Canadian Metallurgical Quarterly 49: 405–410.
- Bayer, T. M., and S. Linneman. 2011. The nature and transport of the fine grain component of Swift Creek landslide, Northwest Washington. Earth Surface Processes and Landforms 36: 624–642.
- Chen, M., and I. O. Ma. 2001. Comparison of three aqua regia digestion methods for twenty Florida soils. Soil Science Society of America Journal 65: 491–499.
- Droste, R. L. 1997. Theory and practice of water and wastewater treatment. New York: Wiley & Sons.
- Holmes, E. P., J. C. Wilson, H. Schreier, and L. M. Lavkulich. 2012. Processes affecting surface and chemical properties of chrysotile: Implications for reclamation of asbestos in the natural environment. Canadian Journal of Soil Science 92: 229–242.
- Januch, J., D. Frank, and L. Edmond. 2006. Office of Environmental Assessment, EPA Region 10, Memorandum to Denise Baker, Site Assessment Manager, Office of Environmental Clean-up, EPA Region 10, Regarding Trip Report and X-ray Diffraction Analysis of Samples Collected 6 April 2006, Project Code ESD-122A, Swift Creek Asbestos Project.
- Katsuhiko, I., and H. Fujitani. 2005. Charge characteristics and related dispersion/flocculation behavior of soil colloids as the cause of turbidity. Colloids and Surfaces 265: 55–63.
- Lavkulich, L. M., H. Schreier, and J. Wilson. 2014. Effects of natural acids on surface properties of asbestos minerals and kaolinite. Journal of Environmental Science & Health A 49: 617–624. doi:10.1080/10934529.2014.865401.
- McGill, W. B., and C. T. Figueiredo. 1993. Total nitrogen. In Soil sampling and method of analysis, ed. M. R. Carter, 201–211. Boca Raton, FL: Canadian Society of Soil Science and Lewis Press.
- Ofir, E., Y. Oren, and A. Adin. 2007. Electro-flocculation: The effect of zeta-potential on particle size. Desalinization 204: 33–38.
- Phillips, J. M., A. Russell, and D. E. Walling. 2000. Time integrated sampling of fluvial suspended sediment: A simple method for small catchments. Hydrological Processes 14: 2589–2602.
- Salopek, B., D. Krasic, and S. Filipova. 1992. Measurement and application of zeta-potential. Rudarsko-Geolosko-Naftni Zbornik 4: 147–151.
- Schreier, H. 1987. Asbestos fibres introduce trace metals into streamwater and sediments. Environmental Pollution 43: 229–242.
- Schreier, H., and L.M. Lavkulich. 2010. Spatial and historic changes in sediments in the Sumas River Watershed affected by an Asbestos rich landslide 1993–2009. Summary Report for US-EPA, Seattle, WA, 31 March 2010, 14 pp.
- Schreier, H., and J.Taylor. 1980. Asbestos fibres in receiving waters. Environment Canada IWD Technical Bulletin #117: 1–19.
- Seshan, K. 1983. How are the physical and chemical properties of chrysotile asbestos altered by a 10-year residence in water and up to 5 days in simulated stomach acid? Environmental Health Perspectives 53: 143–148.
- Smith, I., K. J. Hall, L. M. Lavkulich, and H. Schreier. 2007. Trace metal concentrations in an intensively used agricultural watershed in BC. Journal of the American Water Resources Association 43(6): 1455–1467.
- United States Department of Health. 2006. Swift Creek sediment asbestos, Whatcom County, Washington. Report, US Department of Health and Human Services, Atlanta, GA, 32 pp. http://www.atsdr.cdc.gov/HAC/PHA/SwiftCreekSedimentAsbestos/SwiftCreekHC033006.pdf (accessed November, 2014).
- United States Environmental Protection Agency (USEPA). 1994. Method 200.2; Sample preparation procedure for spectrochemical determination of total recoverable elements. Rev. 2.8. USEPA.
- United States Environmental Protection Agency (USEPA) Ecology and Environment Inc. 2006. Swift Creek asbestos integrated assessment, final report, Everson, Washington. Prepared for the USEPA, Contract Number EP-S7-06-02, Seattle, WA.
- United States Environmental Protection Agency (USEPA) Ecology and Environment Inc. 2007. Summary report of EPA activities Swift Creek asbestos site Whatcom County, Washington. USEPA, Region 10, Seattle, WA. http://yosemite.epa.gov/R10/CLEANUP.NSF/sites/sumasmtndocs/$FILE/ATTTAMDX.pdf/Final+Report.pdf (accessed November, 2014).