Abstract
Alternative management approaches for agricultural streams based on hydrogeomorphological principles such as a two-stage channel are promising, but need to be further tested in contexts other than the Midwestern United States. In Quebec (Canada), the presence of many deep drain outlets may limit their applicability. The objectives of this research are to (1) assess the feasibility of implementing the two-stage channel design in Quebec; (2) determine how alternative management approaches compare with traditional management from an economical point of view, and (3) evaluate the impact of modifying the drain outlet design using small horseshoe wetlands to accommodate hydrogeomorphological adjustments of the channel. A combination of case studies and numerical modelling (HEC-RAS, Hydrologic Engineering Center’s River Analysis System) was used for three study sites located in the Montérégie region (QC). Based on regional hydraulic geometry curves, additional space on each bank required for a two-stage channel ranged between 1.3 and 2.8 m for a width equal to 3 times the inset channel. Hydraulic modelling confirms previous findings of improved drainage capacity at high flow, (up to a 54% increase). Cost analysis reveals that two-stage and over-widened channels are 3 to 4 times more expensive to implement when compared to traditional trapezoidal clean-out dredging operations, mainly due to spoil removal and transportation. However, self-formed channels are less expensive since they only require additional riparian land. A crop yield analysis in the near-stream zone at three sites which are frequently flooded revealed markedly lower productivity in zones within the flooding space. The horseshoe wetland design for drain outlets could also be maintained at low cost, and would promote more heterogeneity than the traditional trapezoidal ditches. Using alternative agricultural stream management approaches based on hydrogeomorphological concepts would be feasible in the Quebec context without compromising the overall productivity of this key economic sector.
Des méthodes de gestion alternatives des cours d’eau agricoles basées sur des principes d’hydrogéomorphologie, comme les chenaux à deux niveaux, sont prometteuses mais requièrent d’être testées dans des contextes différents de ceux des états du Midwest américain. Au Québec (Canada), la présence de drains profonds pourrait limiter leur applicabilité. Les objectifs de cette recherche sont (1) d’évaluer la faisabilité d’implanter les chenaux à deux niveaux au Québec; (2) de comparer le coût de méthodes de gestion alternatives et traditionnelle et (3) de déterminer l’impact d’une modification des sorties de drain à l’aide de zones humides réduites permettant des ajustements hydrogéomorphologiques du chenal. Une combinaison d’études de cas et de modélisation numérique (HEC-RAS, Hydrologic Engineering Center’s River Analysis System) est utilisée pour trois sites d’étude en Montérégie (QC). Selon des courbes régionales de géométrie hydraulique, l’espace additionnel requis pour des chenaux à deux niveaux se situe en moyenne entre 1,3 et 2,8 m pour un chenal ayant 3 fois la largeur du chenal mineur. La modélisation confirme les résultats antérieurs sur l’amélioration de la capacité de drainage (jusqu’à 54%). L’analyse économique révèle que les chenaux à deux niveaux et sur-élargis sont 3 à 4 fois plus coûteux que le dragage traditionnel des chenaux trapézoïdaux, en grande partie en raison du coût d’enlèvement des déblais. Les chenaux auto-formés sont toutefois moins chers puisqu’ils ne nécessitent qu’un peu plus d’espace. Une analyse de la productivité à trois sites fréquemment inondés révèle des rendements nettement plus faibles dans l’espace d’inondabilité. Les sorties de drain aménagées dans des milieux humides réduits diminueraient aussi les coûts d’entretien, tout en procurant une meilleure hétérogénéité que dans les chenaux trapézoïdaux. Ces mesures alternatives de gestion des cours d’eau agricoles basées sur des concepts hydrogéomorphologiques pourraient être employées au Québec sans compromettre la productivité de ce secteur économique névralgique.
Introduction
Improving management practices for agricultural streams is increasingly recognized as critical in order to reduce agricultural nonpoint-source pollution and restore river ecosystems (D’Ambrosio et al. Citation2014; Porter et al. Citation2015). Beneficial agricultural management practices can take many forms, and are often concentrated at the field scale, for example through crop rotation, reduced tillage (Paré et al. Citation2015), livestock exclusion, riparian buffers, manure utilization (Sharpley et al. Citation2003), grassed waterways (Fiener and Auerswald Citation2003), windbreaks (Brandle et al. Citation2004), cover crops (Dabney et al. Citation2001; Snapp et al. Citation2005) and nutrient management (Uusi-Kämppä et al. Citation2000). The width of the riparian buffer, in particular, has received a lot of attention (e.g. A. N. Rousseau et al. Citation2013; Miller et al. Citation2015). In comparison, less attention has been devoted to alternative management of agricultural streams, despite their key role in transporting sediments and pollutants downstream in the drainage network.
Agricultural streams, also referred to as ditches, have often been constructed in areas where there was no organized open-channel drainage system, or modified from previously meandering streams. In Quebec, it is estimated that around 30,000 km of meandering channels were straightened in the twentieth century (Y. Rousseau and Biron Citation2009), a figure similar to the situation in Ohio (D’Ambrosio et al. Citation2014). All these channels were constructed in a similar trapezoidal shape designed to efficiently evacuate water away from the field. These straightened channels were also designed wider and deeper than nature would have intended (D’Ambrosio et al. Citation2014), resulting in problems of sedimentation at low flow and enhanced bank erosion during high-flow events (Rhoads and Herricks Citation1996; Landwehr and Rhoads Citation2003; Huang et al. Citation2010; Rhoads and Massey Citation2012). Both sedimentation and bank erosion problems lead to frequent and costly clean-out operations to ensure that subsurface drain outlets are not clogged with sediments (Rhoads and Massey Citation2012), resulting in further disturbances of these systems (Evans et al. Citation2007; Needelman et al. Citation2007).
Headwater streams are very sensitive to natural and human disturbances such as channelization, and yet their high level of hydrologic independence and ecological autonomy make them ideal sites for successful ecosystem restoration projects (Lowe and Likens Citation2005). Indeed, a recent shift has occurred – mainly in the Midwest United States – towards a better integration of fluvial processes in the design of headwater agricultural channels resulting in a more self-sustaining system and improved ecological function (Powell et al. Citation2007a, 2007b; Huang et al. Citation2010; Jayakaran et al. Citation2010; Roley, Tank, Stephen, et al. Citation2012; Roley, Tank, and Williams Citation2012). These approaches are based on some level of connectivity between the main part of the channel at the bottom of the trapezoidal shape and flat depositional benches. The latter can either be created naturally from channel with sufficient base width (over-widened or self-formed channel) or through the widening of the ditch at the elevation of the existing benches (two-stage channel) (Jayakaran et al. Citation2010). The resulting channels increase water residence during floodplain inundation, with benches harbouring herbaceous vegetation, resulting in increased nitrogen removal via denitrification (Powell and Bouchard Citation2010; Roley, Tank, Stephen, et al. Citation2012; Roley, Tank, and Williams Citation2012).
The growing scientific evidence on the benefits of incorporating hydrogeomorphological concepts in the design and maintenance of straightened agricultural streams, in terms of both water quality and channel stability, first came from case studies and experimental work mainly in the Midwest (Powell et al. Citation2007a, 2007b; Huang et al. Citation2010; Rhoads and Massey Citation2012), but more recently also from Finland where two-stage channel design was adapted with only one side of the channel allowed to reconnect with a floodplain (Västilä and Järvelä Citation2011; Västilä et al. Citation2015). To the best of our knowledge, these hydrogeomorphological approaches have not yet been tested in Canada. In addition to climatic and potential management policy differences between Canadian provinces and states such as Ohio, Michigan and Indiana – where most of the pilot studies on two-stage channels were carried out (Powell et al. Citation2007b; Huang et al. Citation2010) – there are physical constraints that need to be taken into consideration. For example, in Quebec, most of the agricultural landscape is characterized by long and narrow fields, a French “seigneurie” inheritance. Because the most productive agricultural land is located in the flat region of the St. Lawrence Lowlands, and in order to maintain a sufficient slope for artificial tile drainage, drain outlets are often very deep, only a few centimeters above the bed. This poses a challenge for a modification of the shape of the trapezoidal channel, as drain outlets should ideally be located above the elevation of the bench (e.g. Figure ; Roley, Tank, Stephen, et al. Citation2012), which is in fact the case in Midwestern states (Rhoads and Massey Citation2012). Disconnecting drain outlets from ditches would improve water quality as nitrate and phosphorus reach streams through these subsurface drains even in the presence of a wide riparian buffer (Lemke et al. Citation2011). But, as importantly, developing alternative drain outlet designs such as small constructed horseshoe wetlands (Petersen et al. Citation1990) would provide flexibility for watercourse geomorphological adjustments and for a new dynamic equilibrium to be reached.
Figure 1. Location of the three studied watersheds – the Des Fèves, Lacolle and L’Acadie Rivers – as well as the three sites where crop productivity was assessed near the stream (sites A, B and C).
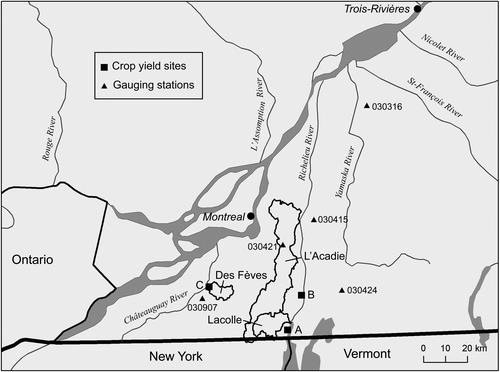
The objectives of this paper are to (1) assess the feasibility of implementing the two-stage channel design in reaches representative of the agricultural landscape of Quebec; (2) determine how alternative management approaches based on hydrogeomorphological concepts compare with traditional management from an economical point of view; and (3) evaluate the impact of modifying the drain outlet design using small horseshoe wetlands to accommodate hydrogeomorphological adjustments of the channel. These objectives are reached through a combination of case studies and numerical modelling.
Methodology
Three alternative approaches to the traditional trapezoidal ditch design are assessed in this study. The most passive approach consists in not intervening and letting the stream adjust by itself towards equilibrium (self-formed channel, which corresponds to a natural two-stage profile). The other alternatives are the two-stage channel and over-widened channel designs.
Study sites
Three agricultural watersheds in the region of Montérégie (Quebec, Canada) were studied in detailed: the Des Fèves, Lacolle and L’Acadie Rivers (Figure ). The Des Fèves River is a tributary of the Chateauguay River, while the two other rivers drain into the Richelieu River (both the Chateauguay and Richelieu are tributaries of the St. Lawrence River). The Montérégie region accounts for a little over 7000 farms, representing a quarter of all agricultural land in the province, mostly dominated by vegetables and corn and soybean crops, which respectively represent 65, 62 and 48% of provincial production (Ministère de l’Agriculture, des Pêcheries et de l’Alimentation Citation2014). Intensive channelization occurred in this agriculturally dominated region during the twentieth century. The oldest available aerial photographs (from 1930) show that the conversion of forested areas to agricultural land was already well advanced in the early twentieth century. Some historical photos show in fact fewer forested areas than today. Only a few higher order meandering rivers remained in 1930 – they were straightened later when machinery became available.
A representative study reach was chosen in each watershed for more detailed analysis: branch 53 of the Des Fèves River (hereafter referred to as Des Fèves), the cours d’eau Barrière, referred to as Lacolle, and branch C of L’Acadie River (here called L’Acadie). Criteria for site selection were the following: a lower order tributary, but with perennial flows; a channelized pre-existing stream; the presence of subsurface drainage that matched typical drainage layout in the province of Quebec; ongoing dredging activities; and openness of farmers. To ensure that each selected reach was a natural stream prior to human intervention, a detailed analysis of a high-resolution (1 m pixel size) Light Detection And Ranging (LiDAR) digital elevation model (DEM) was conducted to verify the presence of fluvial features such as depressions indicating oxbow lakes (former meander loops). This was combined with an analysis of the 1930 aerial photographs to verify the presence of unperturbed streams nearby. Table presents the main features of the three study sites. In each case, the drainage area is in the range considered suitable by Ward et al. (Citation2011) for two-stage channel designs, i.e. between 1 to 10 mi2 (2.6 to 26 km2).
Table 1. Main characteristics of the three study reaches.
An integral part of assessing the impact of implementing alternative stream management approaches is linked to the cost of the additional required riparian space which farmers typically cultivate. A crop yield analysis was thus conducted by sampling additional corn and soy field sites as (1) these crops are highly valuable and (2) they are simple to collect and analyze. The three sites are identified on Figure . Two are located near the Richelieu River (south of Lacolle, site A; and near Saint-Paul-De-L'île-Aux-Noix, site B). Both of these sites may be subject to backwater conditions from the Richelieu River during the spring. The third site is located on the north bank of the Des Fèves River, approximately 1.25 km from the junction with the Chateauguay River (site C), and is a more dynamic fluvial system, less affected by backwater effects.
LiDAR DEMs were available for all three watersheds as well as recent tile drainage plans, obtained from the agro-environmental group PleineTerre, which was a research partner in this project. These plans were georeferenced and imported in a geographic information system (GIS) database using the software ArcGIS (version 10.2) to determine their length and slope. Ortho-images from spring 2014 were also available. Combined with LiDAR DEMs, these images were used to determine the flooding space, which is part of the freedom space as defined by Biron et al. (Citation2014). Aerial photographs from 1930 were georeferenced and the streams were digitized to assess changes in planform geometry that have occurred in the last 85 years. Five gauging stations from the Centre d’Expertise Hydrique du Québec (CEHQ) are located near the study reaches (see Figure ).
Regional curves and two-stage channel design
The two-stage channel design is based on a reference reach approach and uses regional curves of channel dimensions which are related to drainage area (Powell et al. Citation2007a). Optimal two-stage channel dimensions are 3–5 times the inset channel width at bench elevation (Powell et al. Citation2007a). Regional curves allow us to retrieve the appropriate inset channel width for any site based on the drainage area. Such curves exist for Ohio, but they needed to be adapted for Quebec’s agricultural streams. This required identifying reference reaches where no clean-out operations had been conducted, to find natural two-stage profiles. Such sites were found after consulting Municipalités Régionales de Comté (MRC)’s database where dredging clean-out operations are recorded. Bankfull geometry dimensions (width and depth) of seven small agricultural streams distributed across the Montérégie region (drainage areas ranging from 0.2 to 9.9 km2) were measured with a Spectra model LL100N precision laser level, whereas drainage areas were extracted in ArcGIS from the LiDAR DEM. A site with an intermediate drainage area (Des Fèves River main channel, 51.7 km2) was also surveyed and included in the analysis to narrow the gap between small naturalized ditches and the much bigger nearby CEHQ gauged rivers: Des Anglais River (#030907), Des Hurons River (#030415), L’Acadie River (#030421), David River (#030316) and Aux Brochets River (#030424) (see Figure ). These gauging stations have drainage areas well over the typical range for ditches (between 323 and 642 km2). However, researchers at Ohio State University who were consulted for this project often include rivers in their regional curves when they fit well in the regression (Dr. Jonathan Witter, personal communication, 20 July 2014). The bankfull discharge was considered to correspond to a 1.5-year recurrence. Additional information on the 13 sites selected for the establishment of regional curves is presented in Table .
Table 2. Detailed information on the streams used to establish regional curves.
Longitudinal and cross-sectional bathymetry surveys, as well as water surface profiles, were measured with a Juniper Systems Archer XF101 DGPS (differential global positioning system) at the three study sites (Des Fèves, Lacolle and L’Acadie). This information is required to use the Enhanced Ditch Design tools from Ohio State University (Mecklenburg and Ward Citation2004). Since the study reaches are fairly long (Table ), drainage area varies between the upstream and downstream limits. This was taken into account – unlike in the traditional trapezoidal design which uses constant dimensions for extended distances – by extracting drainage area using LiDAR DEM at approximately 500-m intervals along the reach. Based on a simplified geometry (ditch bottom- and top-widths and heights of the banks) extracted at these 500-m intervals, calculations of additional width requirements in the field were made for the different tested designs, as well as the corresponding volume of spoil needed in the case of the two-stage and over-widened channels.
Hydraulic modelling
Bathymetry data collected at the three study reaches were combined with LiDAR DEM to determine how a change in cross-sectional channel shape impacts the channel’s water evacuation capacity and field flooding using the Hydrologic Engineering Center’s River Analysis System (HEC-RAS), a one-dimensional hydraulic model (US Army Corps of Hydraulic Engineering Citationn.d.). Discharge was measured at the Lacolle and Des Fèves sites by installing a thin-plated 90° V-notch weir and measuring water level with a pressure transducer (Solinst LTC Levelogger Junior). Measured discharges were obtained from the following relation (Rantz Citation1982): (1)
where Q is discharge (m3/s) and h is the measured height (m) of water passing through the V-notch. A rating curve was established between discharge obtained from (1) and water level measured with a second pressure transducer located approximately 500 m downstream of the V-notch weir. These precise water levels associated with a range of discharges were used to calibrate and validate the HEC-RAS model based on different roughness coefficients (Manning’s n). A first estimate of Manning’s n was obtained from field observations by assessing the various components of roughness (grain size, presence of obstacles, variable cross-sectional dimensions, vegetation), which was later adjusted to correspond to the measured flow stage (Table ). Note that in contrast with more complex fluvial environments (i.e. where floodplains are highly connected to the stream), channelized streams tend to present rather uniform condition of roughness, which is why a constant value of Manning’s n was used for different flow stages at each site. This is in agreement with other studies in simple channels where roughness value is only modified for overbank flow (e.g. Fry et al. Citation2012).
Table 3. Four levels of discharge used in hydraulic modelling, along with the calibrated Manning’s n.
Four levels of flow were tested with HEC-RAS: low, medium, high and very high (Table ). The very high flow corresponds to the maximum in-channel discharge, i.e. the drainage capacity when the trapezoidal channel is completely full. Note that using the term “bankfull discharge” would be confusing as it typically corresponds to a 1.5–2-year recurrence interval, whereas human-made channels are designed to withhold much higher flows in order to limit flooding in agricultural fields (Kallio Citation2010). The very high discharge was estimated with HEC-RAS by progressively raising the discharge until the water surface corresponded to the top of the trapezoidal channel. The high level corresponds to a 10-year recurrence interval discharge. This was computed based on a Weibull distribution for the 42-year record of the nearest CEHQ gauging station (Des Anglais River). The drainage area method was then used to determine the corresponding discharge for the three study sites, i.e.: (2)
where Qditch is the scaled discharge of the gauging station (Qstation) using a ratio of the drainage areas of the study site (DAditch) and the gauged watercourse (DAstation). Medium- and low-flow discharges were selected according to field measurements for the two sites equipped with a V-notch weir. The corresponding discharges for the third site (L’Acadie River) were computed using the drainage area ratio method. Note that the roughness coefficient obtained by calibration for the medium flow was applied to high and very high flow stages since no direct measurements of flow level for these low-frequency events could be taken during the course of this project.
The hydraulic model was used to test the impact of different stream naturalization approaches (two-stage, over-widened, self-forming channels) on flow stage. These approaches are expected to affect the Manning’s n value due to lower dredging recurrence resulting in vegetation development and some channel sinuosity. A sensitivity analysis of Manning’s n values was conducted in HEC-RAS to measure the impact on water level of increasing resistance.
The hydraulic model was also used to test the impact on water level of modifying the drainage outlet with horseshoe (pocket) wetlands (Figure A). This was accomplished by using additional cross-sections to represent a sudden widening of the trapezoidal channel (Figure B). Ideally, a two-dimensional modelling approach would be needed to assess the impact of horseshoe wetlands on flow dynamics (e.g. recirculation flow) but this was not possible in this study due to time constraints.
Economic analysis
The difference in implementation cost between the three alternative approaches (two-stage, over-widened and self-formed channels) and the trapezoidal ditch design needed to be estimated in the Quebec context. For the most passive of these alternatives, i.e. self-formed, which involves not intervening and letting the stream adjust towards equilibrium, the two major costs consist in land acquisition and tile drainage outlet stabilization as bank erosion will enlarge the stream corridor with time. An estimate for land acquisition was calculated using an average agricultural land price of $19,387 per hectare (all values given in 2015 CAD$) for western Montérégie (Financière Agricole du Québec [FADQ] Citation2014) and multiplying it with the additional area required for every study site (based on a minimum width of 3 times the inset channel width; Powell et al. Citation2007a). The other two alternatives require additional construction costs, which include obtaining permits and designing construction plans. Based on PleineTerre’s estimates for several streams in the MRC Jardins-de-Napierville, this was estimated at $4.5/m.
The construction costs of the two-stage channel design in the Midwest vary from $33 per linear meter to $218/m (Powell et al. Citation2007b). There are no readily available figures for the cost of traditional ditch dredging operations in the province of Quebec, but based on informal discussion with biologists working for the Ministry of Forests, Fauna and Parks, it averages around $25/m, which is the value applied for the traditional approach calculation in this study. The high variability for two-stage channels is mainly linked to the overall amount of earth to be removed but also whether or not the dug materials are to be transported away from the adjacent field. Trapezoidal ditch clean-out operations typically leave the dug earth atop the banks, and producers eventually spread the material over their field as most of the dug sediment is sourced from the top of the fields (i.e. fertile soil). However, mineral horizons cannot simply be pushed over fields as this would considerably weaken crop yields.
Based on a minimal lateral space requirement of 3 times the inset channel width, and taking into consideration the fact that the L’Acadie site has deep organic deposits (compared to mineral layers for the other two sites), construction cost was estimated based on an hourly rate of $170/hour ($1360 for an 8 hour-day) and 300 m/day. For the mineral horizons of the Des Fèves and Lacolle sites, transportation of the material had to be included. Since soil compaction must be avoided, trucks are loaded to half (~15 tons) of their capacity, at an average rate of $10/ton. Lastly, subsurface drainage outlets will need to be adjusted to the new channel width. Based on Quebec’s Ministry of Agriculture estimate for the cost of materials (riprap and geotextile) and labour (mechanical shovel and supervision), this amounts to around $800 per outlet. More details on the economic analysis are presented in Paradis (Citation2015).
According to Bernhardt et al. (Citation2005), the largest expenditures in restoration projects relate to land acquisition. Since all the alternatives based on hydrogeomorphological concepts (two-stage, over-wide and self-formed channels) are wider than trapezoidal channels, an assessment of the value of cultivated riparian land was undertaken to estimate a fair compensation price. Crop productivity was therefore assessed along selected agricultural streams (sites A, B, C; Figure ). It is hypothesized that lower yields are found near streams because these zones are regularly flooded or affected by bank erosion. If this is the case, it can help convince producers to concede riparian land at more affordable prices. For the first year, crop yield was estimated for corn fields for three cross-sections 20 m apart, at three distance intervals from the stream (0–10 m, 10–20 m and 20–30 m), where 0 m corresponds to the limit of the cultivated land, normally 1 m away from the ledge of the bank (i.e. the minimum vegetated buffer strip width requirements for agricultural streams in Quebec. Based on results from the first year which showed lower-than-average yields even at the farthest distance, two sample distances of 30–40 m and 40–50 m were added for the second year to encompass sections with normal yield production. Two of the three cornfields were changed to soy production in that second year. The three sites were characterized by a flat topography, with an elevation above stream bank of less than 0.85 m. It was also assumed that soil compaction was similar between the sites since farmers were using identical ploughing methods. Nevertheless, caution is required in interpreting these crop yield results since other factors such as water table height in root zone or soil compaction could affect yields.
Results
Regional curves and channel dimensions
From the regional curves for the inset channel width (Winset) and depth (D) (Figure ) the following relationships were obtained:(3)
(4)
Figure 3. (A) Diagram showing the dimensions of two-stage channels; (B) regional curves of bankfull channel geometry (Montérégie) for the inset width (Winset in A) and depth.
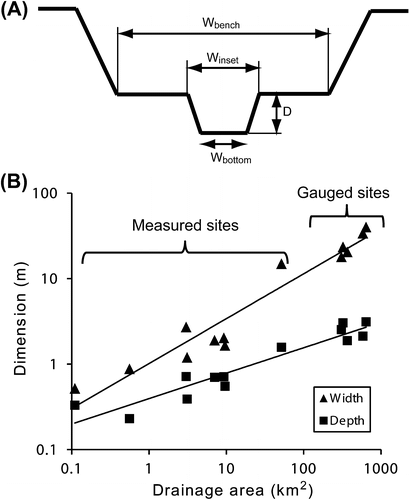
These values are in the same range as those obtained in the St-Joseph River and Loramie Creek (Ohio), where the exponent values for width were 0.47 and 0.40, respectively, and 0.30 and 0.29 for depth, also with high R2 ranging between 0.90 and 0.97 (Powell et al. Citation2007b). Similarities in regional curves between Quebec and Ohio agricultural streams are not surprising when considering the surface deposits of the two regions. Indeed, nearly all of the selected sites in Quebec are located within the Champlain’s sea maximal extent where clay deposits are a common feature (Gadd Citation1988). Extensive clay deposits are also a dominant physiographic unit in Northwestern Ohio, in the region of the Maumee Lake Plains, which comprises among other sub-units the Paulding clay plains (Brockman Citation2007).
Based on Equation (3), the inset channel widths for the Des Fèves, Lacolle and L’Acadie study sites are 1.9, 2.9 and 1.7 m, respectively. The existing channel widths, which should ideally be 3 to 5 times the regional curve values for the inset channel, are in fact very close to the theoretical inset channel width for the three study sites (Table ). Therefore, additional space is required for naturalization processes to occur (Figure ). The increased width requirements for each site are presented in Table , along with the corresponding volume of spoil. For a width equal to 3 times the inset channel, the average additional width ranges from 1.3 to 2.8 m for the three sites, whereas the average additional width is comprised between 2.6 and 5.6 m when using 5 times the inset channel (Table ). The minimal values of additional width were found in the upstream reaches, where drainage area is smaller. This highlights one of the problems with the traditional design of ditches, i.e. they do not follow hydraulic geometry concepts, where width and depth should increase from upstream to downstream. Instead, current designs in Quebec use a constant trapezoidal shape for extensive distances (as some of the straightened channels can be several kilometers in length).
Figure 4. Visual representation of a two-stage channel design (3 and 5 times the inset channel width) over an actual cross-section of branch 53 of the Des Fèves River, also showing the location of the deep subsurface drainage outlet measured in the field (the inset channel width is 1.62 m).
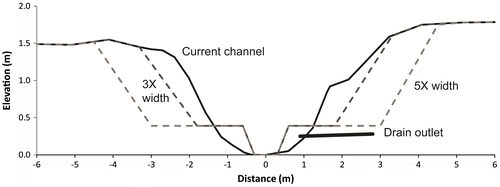
Table 4. Additional width required for each bank and corresponding volume of spoil to be removed for two-stage and over-wide ditch designs.
Hydraulic modelling
Figure presents HEC-RAS modelling results for the Lacolle site. Not surprisingly, the flow stage increases with a two-stage channel design for the low and medium discharge values since flow is then concentrated in the inset channel. However, a decrease in flow stage occurs for the two highest discharges, averaging around 5.5% for the 3×-width design, and 15% for the 5×-width design. Consequently, the drainage capacity of the two-stage channels, i.e. the maximum discharge contained within the largest trapezoidal channel, increases for all three sites by about 13% for the 3× configuration, and by 48% for the 5× configuration (Table ).
Figure 5. Hydrologic Engineering Center's River Analysis System (HEC-RAS) output for (A) the actual channel configuration of the Lacolle site, (B) the corresponding two-stage profile with 3 times the inset channel width, and (C) the corresponding two-stage profile with 5 times the inset channel width compared to the floodplain width. Values on the right in B and C correspond to the difference in flow stage compared to the actual channel for the four discharge values.
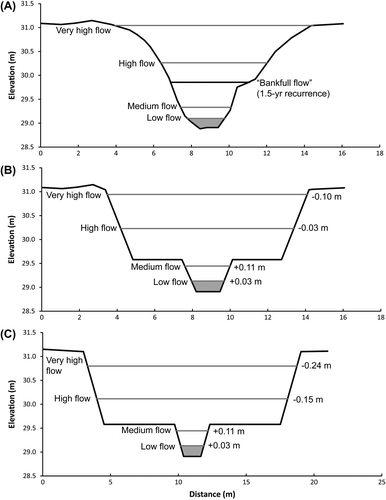
Table 5. Effect of the two-stage profiles on the maximum in-stream discharge (drainage capacity).
With reduced maintenance for a two-stage channel design, more vegetation is expected on the benches and banks, resulting in a possible increase in the Manning’s n roughness value and decreased drainage capacity (Helmiö Citation2002). A sensitivity analysis was therefore conducted for the Lacolle site to assess how an increase in roughness could affect drainage capacity (Table ). With increasing roughness, maximum in-stream flow stage is rapidly reached in the case of the 3×-width design, but less so in the case of the wider channel (5×). Lower water levels for a larger vegetated floodplain in compound channels were also observed by Helmiö (Citation2002) and would suggest that a design closer to 5 rather than 3 times the inset channel width is preferable. It is obviously difficult to quantify precisely the impact of vegetation growth related to lack of channel maintenance as this will clearly evolve with time. Furthermore, reduced velocities due to the increase in vegetation will likely have an effect on sediment deposition, which would need to be assessed through long-term monitoring. Since there are many similarities between Midwest and Quebec agricultural channels, as revealed by the regional curve analysis, it would be possible to follow the geomorphological adjustment of some Midwestern two-stage ditches which have been constructed over a decade ago, for example the Crommer ditch (Michigan) and a tributary to Bull creek (Ohio) which were built in 2003 and 2001, respectively (Powell et al. Citation2007b).
Table 6. Sensitivity analysis of water stage for the very high flow discharge of the Lacolle site (6.5 m3/s) when increasing Manning’s n.
Constructing a two-stage channel creates a problem with deep drain outlets which are characteristic of Quebec’s agricultural landscape. As illustrated in Figure , deep outlets would exit in one of the benches, which is clearly problematic for drainage. One option could be to prolong the outlet in the inset channel. However, considering the problem of nitrate and phosphorus pollution due to drains exiting directly in the stream (Lemke et al. Citation2011), a more sustainable option would be to modify the drain outlet zone to create horseshoe (or pocket) wetlands (Figure A). This solution has the additional advantage of modifying the dredging maintenance operation from a linear process to a punctuated one, i.e. sediments only need to be removed from small pockets instead of from entire reaches.
Hydraulic modelling based on the planform geometry modifications illustrated in Figure B reveals that the hydraulic influence of a 3 × 3 m horseshoe wetland on a 6-m wide trapezoidal ditch would be small overall (Table ). There would be a slight increase in stage with reduced velocity for both low flow (0.2 m3/s) and high flow (4 m3/s) tested events (Table ). Local channel widening causes a considerable reduction in velocity as the wetted perimeter increases sharply. It also explains the slight increase in stage (due to a change in the balance between kinetic and potential energy). Since the horseshoe wetland design has not yet been implemented, it is not possible to validate these simulated results at this stage. One of the main concerns with this technique is accelerated sediment deposition in the wetland and thus a more frequent need to dredge. The rate of sediment accumulation was not estimated here. This would require two- or three-dimensional morphodynamic modelling to properly account for the recirculation effect created by the wetland.
Table 7. Flow characteristics before and after integration of a 3 × 3 m horseshoe wetland on a 6-m wide ditch.
Economic analysis
The additional land required for a two-stage channel design is often perceived as the largest expenditure, limiting the chances of this approach being socially accepted. For the 3×-width design, this represents areas of 0.85, 2.22 and 0.15 ha for the sites of Des Fèves, Lacolle and L’Acadie, respectively. At an approximate land value of $20,000/ha in the Montérégie area (FADQ Citation2014), this translates into over $40,000 for the Lacolle site. When adding to this value the costs for permits, plans and site surveillance, the 3× two-stage or over-widened channels throughout the study reaches amount to $29,339, $60,724 and $6,108 for the Des Fèves, Lacolle and L’Acadie sites, respectively. The subsurface drainage outlet adjustment, fertile and mineral soil removal, and mineral horizon transportation costs are estimated at $140,255, $505,200 and $11,680 for the 3× two-stage design, and at $182,583, $737,320 and $11,680 for the over-wide design (for Des Fèves, Lacolle and L’Acadie sites, respectively). Therefore, with the exception of the L’Acadie site which is characterized by organic soils, land acquisition is only around 10% of the total cost of two-stage and over-wide channels (25% for the L’Acadie site).
Table presents a cost comparison between the traditional trapezoidal channel and the three alternate approaches tested here. It is interesting to note that the same high variability of costs mentioned by Powell et al. (Citation2007a) for the two-stage channel design is observed in this analysis (between $37 and $144/m). Even if some factors are difficult to quantify precisely (e.g. distance of transportation of mineral spoil), two broad conclusions can be drawn with confidence about the two-stage and over-wide ditch designs: (1) cost varies greatly according to factors such as drainage area and soil type; and (2) these alternatives will always be more expensive to implement than the traditional ditch dredging operations, especially in mineral soil areas. However, comparing the traditional dredging approach with two-stage or over-wide channels is questionable since one is a maintenance operation (traditional ditch dredging) and the others require the design of a new channel. Strictly speaking, the comparison should be made with the creation of new traditional ditches, but they have not been constructed in over 30 years. Furthermore, it is important not to limit the analysis to the additional cost highlighted in Table , but to also consider that a two-stage profile is expected to greatly reduce the need for recurrent maintenance (Powell et al. Citation2007a), thus reducing cost in the long term. For example, assuming a maintenance recurrence of 50 years for alternative management options and a current average recurrence for dredging between 15–20 years, there would normally be two or three dredging operations conducted during that time period (assuming agricultural practices remain the same). This converts into a cost of $50–75/m, which narrows considerably the gap between the cost of conventional and alternative approaches.
Table 8. Comparison of total cost for a traditional dredging approach, self-formed channel approach, two-stage channel and over-widened channel designs.
One other interesting finding of Table is the very low cost of the self-formed stream – a passive approach to stream management which does not receive as much attention as the two-stage channel design despite providing the same end result with minimal human intervention. A major reason for this is the relatively low cost of land acquisition in comparison with other expenses. The land acquisition cost may be even further reduced when considering spatial variability in crop yields in the critical riparian zone (see below), making self-formed channels as well as other management approaches requiring more space to rivers (e.g. wetland preservation [Mann et al. Citation2014]; freedom space for rivers [Biron et al. Citation2014]) even more appealing.
The two sites near the Richelieu River (sites A and B; Figure ) have crop productivity in the riparian zone which is clearly very affected by the different flood levels reached by the Richelieu. In 2013, peak discharge was 0.27 m lower than in 2014. At site A, the maize productivity in the first interval (0–10 m from the stream) was close to 0 in 2013, and reached levels of around 1 ton/ha in the 10–20-m interval, and only around 3 tons/ha in the 20–30-m zone. In comparison, the regional reference maize yield for insurance in that region is 9.27 tons/ha (FADQ Citation2014). In 2014, the severe spring flood discouraged the farmer from attempting sowing in the flood space. At site B, the flooding space extends about 14 m away from the stream. There was a marked increase in maize crop productivity in 2013 at distances above the flooding space limit, jumping from 4.7 tons/ha in the 0–10-m zone to about 8.4 tons/ha in the 10–20-m interval, remaining at this value for the 20–30-m zone. In 2014, soy crops did not reveal such a strong relationship, although soy productivity at cross-section 3 nearly doubled outside the flooding space (from 1.28 to 2.41 tons/ha).
Site C, covering a former meander of the straightened Des Fèves River, is representative of a larger, more dynamic fluvial system. It exhibited the strongest and clearest relationship between crop productivity and distance to the stream for both years. Since the 2013 data do not cover the zone beyond flooding space, only 2014 results are presented in Figure , which highlights a linear increase in maize productivity with distance. On average, yields in the 40–50-m interval (outside the flooding space) are 67% higher than those between 0 and 40 m, with only very small variation between cross-sections, in contrast with the other two sites which showed more variability. Cross-sections 1 and 2 outside the flooding space are the only ones to feature maize yields above the regional reference yields for insurance.
Discussion
The case studies analyzed in this research revealed many similarities with previous findings on two-stage channels documented in the Midwest, in terms of both regional curves and hydraulic impact. Since research on alternative approaches for agricultural drainage is considerably more advanced in the Midwest than in Quebec and other Canadian provinces, these strong analogies will favour fast progress in the implementation of these concepts, at least where the political and social willingness to move away from traditional trapezoidal ditches is present.
Our hydraulic modelling results clearly reveal that two-stage profiles do not undermine the drainage capacity of channelized streams. On the contrary, drainage is often improved for very high-flow events, concurring with other studies conducted in the Midwest (e.g. Powell et al. Citation2007a; Jayakaran et al. Citation2010). The potential for reduced velocities at high flow due to increased momentum transfer through the cross-section (Myers Citation1978; Knight and Hamed Citation1984) and increased vegetation on the banks and benches is also beneficial for reducing bank erosion and suspended load in these channels (Krider et al. Citation2014; Wang et al. Citation2014). At the other end of the flow spectrum, low water flows tend to have a higher stage when benches are incorporated as they narrow the width of the bottom of the ditch. This may result in a more suitable habitat for several water fauna species (Rhoads et al. Citation2003; Rhoads and Massey Citation2012). A natural two-stage profile is also likely more resilient as it can help attenuate the effects of increased extreme weather events, both dry and wet, which are expected to affect several regions of the world including Quebec (Ouranos Citation2014). Another major benefit of improving hydraulics is the potential gain in crop yield for flood-prone fields.
The main downside to the hydraulics of the two-stage channel is caused by one of its major benefits: reduced maintenance. This can translate into a long-term increase in roughness as vegetation expands, reducing drainage compared to the initial trapezoidal ditch model. Since no implemented two-stage channel in the Midwest is older than 15 years, it is hard to estimate the timeframe for this to happen. However, many naturally formed two-stage ditches have prevailed for several decades without significant maintenance and still feature predominantly grassed banks and benches. Furthermore, the sensitivity analysis presented in this study reveals that roughness would need to be increased markedly to result in reduced drainage capacity, particularly for a wider (5×-width) configuration (Manning’s n up to 0.07; Table ). An investigation of the vegetation succession in those systems would, however, be needed to better predict how these channels will evolve in the long term.
Although agricultural streams in Quebec are in many ways similar to those of the Midwest, the depth of drain outlets seems to be a unique feature in Quebec, likely due to the very different cadastral layout inherited from the French seigneurie system, with long and narrow fields. In addition, Ohio drainage systems are aligned with the width, whereas in Quebec they follow the length (Paradis Citation2015). At the Des Fèves site, 19% of the drain outlets were below the calculated level of the two-stage channel bench, whereas this was the case for 1/3 of the outlets at the Lacolle site (Paradis Citation2015). The peculiarity of Quebec’s agricultural landscape requires a novel solution to ensure that alternative ditch management approaches are not in conflict with drain outlets. The proposed approach of horseshoe (or pocket) wetland (Figure A) seems promising; however, it has not been tested yet and its potential thus remains speculative.
Consulted farmers were in fact quite enthusiastic about the pocket wetland concept, particularly because it would give them control over the management of their subsurface drainage outlets. It is also a fairly inexpensive approach, which was estimated at around $2100 per drain for the Des Fèves and Lacolle sites. When divided by the study reach length, this corresponds only to about $4/m (compared to $25/m for standard dredging). However, many consulted farmers raised concerns about the bank erosion potential risk during high-magnitude events. Another risk is that massive sediment deposition would occur in these miniature wetlands because of the abrupt widening of the channel, resulting in drain clogging. Finally, issues were raised concerning the modification of the shape of the field which will be cumbersome for heavy machinery circulation. The degree of disturbance varies according to the location of the outlet: in most situations it is located at the corner of a field which is already hard to reach for machinery, while drains in the middle of the field are rarer and mostly present for very wide fields. Further work is needed before considering any widespread implementation of the horseshoe wetland approach. This should focus on two-dimensional morphodynamic modelling (to assess the impact of the recirculation zone within the wetland and overall sediment dynamics) and a first experimental site to collect data which could be used to calibrate and validate a model. This work would also help determine in which cases horseshoe wetlands would not be applicable, and develop other drain outlet solutions for these situations.
The economic analysis reveals that on average, two-stage and over-wide channel total implementation cost is around $80/m and $105/m, respectively, although there was a lot of variability between sites which mainly depended on the volume of mineral soil horizon removal and transportation (Table ). Clearly, this high cost will constitute a major obstacle to modify agricultural stream management approach. However, the current environmental management paradigm of including ecological services in economic analysis could improve the social acceptability of the aforementioned alternatives. For example, one of the most important benefits associated with these floodplain-integrated alternatives is the marked reduction of nitrate concentrations in water which improves downstream water quality and aquatic habitat (Roley, Tank, Stephen, et al. Citation2012; Roley, Tank, and Williams Citation2012). As nitrate plays a key role in algal blooms and other environmental problems such as hypoxia in the St. Lawrence estuary (Thibodeau et al. Citation2006) and several smaller estuaries of Prince Edward Island (Danielescu and MacQuarrie Citation2011), it appears increasingly essential in future studies to quantify these ecological benefits if a long-term sustainable vision of agricultural stream management is to be developed. Alternatives based on hydrogeomorphological concepts such as two-stage channels also require far less interventions in the long run, which needs to be factored in when assessing costs and benefits of different approaches. In fact, the more passive “self-forming” channel approach, which only requires additional space along the streams, is by far the cheapest ($12/m on average; Table ). Since crop yields were shown to be markedly lower in these near-stream zones (Figure ), letting agricultural ditches adjust to form natural benches with a sinuous smaller inset channel should be the preferred option. This is in many ways similar to the concept of freedom space developed for larger rivers (Biron et al. Citation2014).
Conclusion
This study has examined the perspectives, problems and prospects of integrating hydrogeomorphological concepts in management approaches of lowland agricultural streams using three study sites located in the St. Lawrence Lowlands in Quebec. Results show many similarities with case studies from the Midwestern United States, indicating a high potential for application of more sustainable approaches such as two-stage, over-widened or self-formed channels in Quebec. The additional space required for a two-stage channel ranged on average between 1.3 and 2.8 m from each bank for the three study sites when using a design based on 3 times the inset channel width, and from 2.6 to 5.6 m when using 5 times the inset channel width (Table ). Hydraulic modelling using HEC-RAS confirmed previous findings of improved drainage capacity for high-flow events, with up to a 54% increase in maximum discharge. A cost analysis reveals that two-stage and over-widened channels are 3 to 4 times more expensive to implement when compared to traditional trapezoidal clean-out dredging operations, mainly due to spoil removal and transportation. However, self-formed channels are markedly less expensive (about half the cost of clean-out dredging) since they only require additional riparian land.
A crop yield analysis in the near-stream zone at three sites which are frequently flooded revealed markedly lower values in zones that are within the flooding space, which varied between about 15 and 40 m from the stream at the three sites. A more systematic investigation of crop yield in near-stream zones, including sites that are less frequently flooded, would be particularly useful, as land productivity is very often the main reason why farmers are reluctant to accept novel management approaches based on natural fluvial processes which inevitably require more space around streams.
The deep drain outlets often encountered in Quebec are another potential problem for the implementation of alternate management approaches. A novel design is proposed here, with horseshoe (pocket) wetlands which could easily be maintained at low cost, and which would promote more heterogeneity than the traditional trapezoidal ditches do.
Considering the high level of impairment of rivers in most agricultural watersheds and the necessity to increase the resilience of fluvial systems with the expected increase in extreme events associated with climate change, the development of alternative management approaches for agricultural streams based on hydrogeomorphological concepts should be a high priority for all levels of government. This study shows that it is possible to do so without compromising the overall productivity of this key economic sector, although a reassessment of alternative stream designs would likely be required if climate change resulted in a markedly different hydrological regime.
Acknowledgements
This project was funded by the Fonds de Recherche du Québec – Nature et Technologies (FRQNT), the Natural Sciences and Engineering Research Council of Canada (NSERC), and Pleine Terre through a BMP scholarship to A. Paradis. P. M. Biron also acknowledges support from the NSERC Discovery grant program. Thanks to Samuel Comtois from PleineTerre, and to Jon Witter, Jessica D’Ambrosio, Mike Brooker, and Professors Andrew Ward and Larry Brown from Ohio State University’s Department of Food, Agricultural and Biological Engineering. The comments of two anonymous reviewers on a previous version of this manuscript are greatly appreciated.
References
- Bernhardt, E. S., M. Palmer, J. D. Allan, G. Alexander, K. Barnas, S. Brooks, and E. Sudduth. 2005. Synthesizing US river restoration efforts. Science 308 (5722): 636–637.
- Biron, P. M., T. Buffin-Bélanger, M. Larocque, G. Choné, C.-A. Cloutier, M.-A. Ouellet, S. Demers, T. Olsen, C. Desjarlais, and J. Eyquem. 2014. Freedom space for rivers: A sustainable management approach to enhance river resilience. Environmental Management 54 (5): 1056–1073.
- Brandle, J. R., L. Hodges, and X. H. Zhou. 2004. Windbreaks in North American agricultural systems. In New vistas in agroforestry, 65–78. The Netherlands: Springer. Kluwer Academic Publishers.
- Brockman, C. S. 2007. Physiographic regions of Ohio. Ohio DNR – Division of Geological Survey. Online map. http://www.people.iup.edu/kpatrick/Great%20Lakes/Ohio%20Physiography.pdf (accessed January, 2015).
- Dabney, S. M., J. A. Delgado, and D. W. Reeves. 2001. Using winter cover crops to improve soil and water quality. Communications in Soil Science and Plant Analysis 32 (7–8): 1221–1250.
- D’Ambrosio, J. L., L. R. Williams, M. G. Williams, J. D. Witter, and A. D. Ward. 2014. Geomorphology, habitat, and spatial location influences on fish and macroinvertebrate communities in modified channels of an agriculturally dominated watershed in Ohio, USA. Ecological Engineering 68: 32–46.
- Danielescu, S., and K. T. MacQuarrie. 2011. Nitrogen loadings to two small estuaries, Prince Edward Island, Canada: A 2-year investigation of precipitation, surface water and groundwater contributions. Hydrological Processes 25 (6): 945–957.
- Evans, R. O., K. L. Bass, M. R. Burchell, R. D. Hinson, R. Johnson, and M. Doxey. 2007. Management alternatives to enhance water quality and ecological function of channelized streams and drainage canals. Journal of Soil and Water Conservation 62 (4): 308–320.
- Fiener, P., and K. Auerswald. 2003. Effectiveness of grassed waterways in reducing runoff and sediment delivery from agricultural watersheds. Journal of Environmental Quality 32 (3): 927–936.
- Financière Agricole du Québec (FADQ). 2014. Rendements de référence 2014 en assurance récolte. Direction de l’assurance récolte. http://www.fadq.qc.ca/statistiques_et_taux/statistiques/assurance_recolte/rendements_de_reference.html (accessed February, 2015).
- Fry, B. E., A. Ward, and K. W. King. 2012. The frequency of channel-forming discharges in a tributary of Upper Big Walnut Creek, Ohio. Journal of Soil and Water Conservation 67 (3): 173–182.
- Gadd, N. R. 1988. The Late Quaternary development of the Champlain Sea basin. Proceedings of a symposium held in Ottawa in may 1986. Geological Association of Canada Special Paper 35. 312 pp.
- Helmiö, T. 2002. Unsteady 1D flow model of compound channel with vegetated floodplains. Journal of Hydrology 269: 89–99.
- Huang, J.-C., W. J. Mitsch, and A. D. Ward. 2010. Design of experimental streams for simulating headwater stream restoration. Journal of the American Water Resources Association 46 (5): 957–971.
- Jayakaran, A. D., D. E. Mecklenburg, J. D. Witter, A. D. Ward, G. E. Powell. 2010. Fluvial processes in agricultural ditches in the North Central Region of the United States and implications for their management. In Agricultural drainage ditches: Mitigation wetlands for the 21st century, ed. M. T. Moore and R. Kröger, 195–222. Kerala, India: Research Signpost.
- Kallio, S. E. 2010. Determining the bankfull discharge exceedance potential of agricultural ditches in Ohio. Unpublished MSc thesis, The Ohio State University.
- Knight, D. W., and M. E. Hamed. 1984. Boundary shear in symmetrical compound channels. Journal of Hydraulic Engineering 110 (10): 1412–1430.
- Krider, L., G. Kramer, B. Hansen, J. Magner, L. Lahti, B. DeZiel, L. Zhang, et al. 2014. Cedar River alternative ditch designs: The assessment of a self‐sustaining ditch design in Mower County, Minnesota. Minnesota Pollution Control Agency Final Report. https://wiki.umn.edu/pub/Wilson/DownloadReports/AlternativeDitchDesign_EPA319_FinalReport.pdf (accessed July, 2015).
- Landwehr, K. L., and B. L. Rhoads. 2003. Depositional response of a headwater stream to channelization, East Central Illinois, USA. River Research and Applications 19(1): 77–100.
- Lemke, A. M., K. G. Kirkham, T. T. Lindenbaum, M. E. Herbert, T. H. Tear, W. L. Perry, and J. R. Herkert. 2011. Evaluating agricultural best management practices in tile-drained subwatersheds of the Mackinaw River. Illinois. Journal of Environmental Quality 40 (4): 1215–1228.
- Lowe, W. H., and G. E. Likens. 2005. Moving headwater streams to the head of the class. BioScience 55 (3): 196–197.
- Mann, J., C. Grant, and S. Kulshreshtha. 2014. Economics of a pricing mechanism to compensate rural land owners for preserving wetlands. Canadian Water Resources Journal 39(4): 462–471.
- Ministère de l’Agriculture, des Pêcheries et de l’Alimentation (MAPAQ). 2014. Portrait agroalimentaire: La Montérégie. Directions régionales de la Montérégie, secteurs est et ouest. http://www.mapaq.gouv.qc.ca/fr/Publications/Portrait_agroalimentaire_monteregie.pdf (accessed January, 2015).
- Mecklenburg, D. E., and A. D. Ward. 2004. STREAM modules: Spreadsheet tools for river evaluation, assessment and monitoring. ASAE Publication ( 701P0904) St. Joseph, Michigan.
- Miller, J. J., T. Curtis, D. S. Chanasyk, and S. Reedyk. 2015. Influence of mowing and narrow grass buffer widths on reductions in sediment, nutrients, and bacteria in surface runoff. Canadian Journal of Soil Science 95 (2): 139–151.
- Myers, W. R. C. 1978. Momentum transfer in a compound channel. Journal of Hydraulic Research 16 (2): 139–150.
- Needelman, B., P. Kleinman, J. Strock, and A. Allen. 2007. Improved management of agricultural drainage ditches for water quality protection: An overview. Journal of Soil and Water Conservation 62 (4): 171–178.
- Ouranos. 2014. Vers l’adaptation. Synthèse des connaissances sur les changements climatiques au Québec – Partie 1: Évolution climatique au Québec. Édition 2014. Montréal, Québec: Ouranos.
- Paradis, A. 2015. Integrating hydrogeomorphological concepts in management approaches of lowland agricultural streams in Quebec: Perspectives, problems and prospects. Unpublished MSc thesis, Concordia University.
- Paré, M. C., J. Lafond, and D. Pageau. 2015. Best management practices in northern agriculture: A twelve-year rotation and soil tillage study in Saguenay–Lac-Saint-Jean. Soil & Tillage Research 150: 83–92.
- Petersen, R. C., L. B. M. Petersen, and J. O. Lacoursière. 1990. Restoration of lowland streams: The building block model. Vatten 46 (4): 244–249.
- Porter, P. A., R. B. Mitchell, and K. J. Moore. 2015. Reducing hypoxia in the Gulf of Mexico: Reimagining a more resilient agricultural landscape in the Mississippi River Watershed. Journal of Soil and Water Conservation 70 (3): 63A–68A.
- Powell, G. E., and V. Bouchard. 2010. Is denitrification enhanced by the development of natural fluvial morphology in agricultural headwater ditches? Journal of the North American Benthological Society 29 (2): 761–772.
- Powell, G. E., A. D. Ward, D. E. Mecklenburg, and A. D. Jayakaran. 2007a. Two-stage channel systems: Part 1, a practical approach for sizing agricultural ditches. Journal of Soil and Water Conservation 62 (4): 277–286.
- Powell, G. E., A. D. Ward, D. E. Mecklenburg, and A. D. Jayakaran. 2007b. Two-stage channel systems: Part 2, case studies. Journal of Soil and Water Conservation 62 (4): 286–296.
- Rantz, S. E. 1982. Measurement and computation of streamflow: Volume 2 – computation of discharge. United States Geological Survey Water Supply Paper 2175. 118 pp. U.S. Government Printing Office Washington, D.C.
- Rhoads, B. L., and E. E. Herricks. 1996. Naturalization of headwater streams in Illinois. In River channel restoration, ed. A. Brookes and D. Shields, 331–367. UK: John Wiley & Sons. Wiley, Chichester
- Rhoads, B. L., and K. D. Massey. 2012. Flow structure and channel change in a sinuous grass-lined stream within an agricultural drainage ditch: Implications for ditch stability and aquatic habitat. River Research and Applications 28: 39–52.
- Rhoads, B. L., J. S. Schwartz, and S. Porter. 2003. Stream geomorphology, bank vegetation, and three-dimensional habitat hydraulics for fish in Midwestern agricultural streams. Water Resources Research 39(8): 1218. doi: 10.1029/2003WR002294
- Roley, S. S., J. L. Tank, M. L. Stephen, L. T. Johnson, J. J. Beaulieu, and J. D. Witter. 2012. Floodplain restoration enhances denitrification and reach-scale nitrogen removal in an agricultural stream. Ecological Applications 22 (1): 281–297.
- Roley, S. S., J. L. Tank, and M. A. Williams. 2012. Hydrologic connectivity increases denitrification in the hyporheic zone and restored floodplains of an agricultural stream. Journal of Geophysical Research: Biogeosciences 117 (G3). doi: 10.1029/2012JG001950
- Rousseau, A. N., S. Savary, D. W. Hallema, S. J. Gumiere, and E. Foulon. 2013. Modeling the effects of agricultural BMPs on sediments, nutrients, and water quality of the Beaurivage River watershed (Quebec, Canada). Canadian Water Resources Journal 38 (2): 99–120.
- Rousseau, Y., and P. M. Biron. 2009. Geomorphological impacts of channel straightening in an agricultural watershed, Southwestern Québec. The Northeastern Geographer 1: 91–113.
- Sharpley, A. N., J. L. Weld, D. B. Beegle, P. J. Kleinman, W. J. Gburek, P. A. Moore, and G. Mullins. 2003. Development of phosphorus indices for nutrient management planning strategies in the United States. Journal of Soil and Water Conservation 58 (3): 137–152.
- Snapp, S. S., S. M. Swinton, R. Labarta, D. Mutch, J. R. Black, R. Leep, J. Nyiraneza, and K. O’Neil. 2005. Evaluating cover crops for benefits, costs and performance within cropping system niches. Agronomy Journal 97 (1): 322–332.
- Thibodeau, B., A. de Vernal, and A. Mucci. 2006. Recent eutrophication and consequent hypoxia in the bottom waters of the Lower St. Lawrence Estuary: Micropaleontological and geochemical evidence. Marine Geology 231(1): 37–50.
- US Army Corps of Hydraulic Engineers. n.d. HEC-RAS. http://www.hec.usace.army.mi (accessed January, 2015).
- Uusi-Kämppä, J., B. Braskerud, H. Jansson, N. Syversen, and R. Uusitalo. 2000. Buffer zones and constructed wetlands as filters for agricultural phosphorus. Journal of Environmental Quality 29: 151–158.
- Västilä, K., and J. Järvelä. 2011. Environmentally preferable two-stage drainage channels: Considerations for cohesive sediments and conveyance. International Journal of River Basin Management 9 (3–4): 171–180.
- Västilä, K., J. Järvelä, and H. Koivusalo. 2015. Flow–vegetation–sediment interaction in a cohesive compound channel. Journal of Hydraulic Engineering 4015034 (11). doi: 10.1061/(ASCE)HY.1943-7900.0001058
- Ward, A. D., G. E. Powell, A. D. Jayakaran, J. E. Witter, J. L. D'Ambrosio, and D. Mecklenburg. 2011. Selecting and sizing a two-stage channel system in an agricultural landscape. Madison, WI, USA. http://greatlakeswater.uwex.edu/sites/default/files/library/outreach-and-education/sizing-fv.pdf (accessed January, 2015).
- Wang, W., W.-X. Huai, and M. Gao. 2014. Numerical investigation of flow through vegetated multi-stage compound channel. Journal of Hydrodynamics 26 (3): 467–473.