Abstract
Terrestrial carbon fluxes are an important factor in regulating concentrations of atmospheric carbon dioxide (CO2). In this study, we use a coupled climate model with interactive biogeochemistry to benchmark the simulation of net primary productivity (NPP) and its response to elevated atmospheric CO2. Short-term field experiments such as Free-Air Carbon Dioxide Enrichment (FACE) studies have examined this phenomenon but it is difficult to infer trends from only a few years of field data. Here, we employ the University of Victoria's Earth System Climate Model (UVic ESCM) version 2.8 to compare simulated changes in NPP due to an elevated atmospheric CO2 concentration of 550 ppm to observed increases in NPP of 23% ±2% from four temperate forest FACE studies between 1997 and 2002. We further compare two scenarios: elevated CO2 with climate change, and elevated CO2 without climate change, the latter being consistent with FACE methodology. In the climate change scenario global terrestrial and forest-only NPP increased by 24.5% and 27.9%, respectively, while these increases were 21.0% and 17.2%, respectively, in the latitude band most representative of the location of the FACE studies. In the scenario without climate change, terrestrial and forest-only NPP increased instead by 28.3% and 30.6%, respectively, while these increases were 24.3% and 14.4%, respectively, in the FACE latitudes. This suggests that the model may underestimate temperate forest NPP increases when compared to results from temperate forest FACE studies and highlights the need for both increased experimental study of other forest biomes and further model development.
R ésumé [Traduit par la rédaction] Les flux de carbone terrestres sont un facteur important dans la régulation des concentrations de dioxyde de carbone (CO2) atmosphérique. Dans cette étude, nous utilisons un modèle climatique couplé et une biogéochimie interactive pour mettre au banc d'essai la simulation de la productivité primaire nette (PPN) et sa réponse à une concentration élevée de CO2 atmosphérique. Diverses expériences à court terme sur le terrain, comme le projet FACE (Free-Air Carbon Dioxide Enrichment), se sont penchées sur ce phénomène, mais il est difficile de dégager des tendances avec seulement quelques années de données de terrain. Ici, nous employons la version 2.8 du modèle climatique du système terrestre de l'Université de Victoria (UVic ESCM) pour comparer les changements simulés dans la PPN résultant d'une concentration de CO2 atmosphérique élevée de 550 ppm aux accroissements observés de PPN de 23 ± 2 % d'après quatre études FACE sur la forêt tempérée menées entre 1997 et 2002. Nous comparons, en outre, deux scénarios : CO2 élevé avec changement climatique et CO2 élevé sans changement climatique, ce dernier correspondant à la méthodologie FACE. Dans le scénario avec changement climatique, la PPN de la terre entière et celle de la forêt seulement se sont accrues de 24,5 % et 27,9 %, respectivement, alors que les accroissements étaient de 21,0 % et 17,2 %, respectivement, dans la bande de latitudes la plus représentative du lieu où le projet FACE s'est déroulé. Dans le scénario sans changement climatique, la PPN de la terre et celle de la forêt seulement se sont plutôt accrues de 28,3 % et 30,6 %, respectivement, alors que les accroissements étaient de 24,3 % et 14,4 %, respectivement, dans les latitudes FACE. Cela donne à penser que le modèle sous-estime peut-être les accroissements de PPN de la forêt tempérée comparativement aux résultats du projet FACE sur la forêt tempérée et met en évidence le besoin de poursuivre les études expérimentales sur d'autres biomes forestiers et de développer davantage les modèles.
1 Introduction
Continually increasing anthropogenic emissions of carbon dioxide (CO2) to the Earth's atmosphere is an issue of great concern to the contemporary world because of the significant environmental and socio-economic impacts associated with the resulting changes in global climate. Therefore, projecting the impacts of rising atmospheric CO2 concentrations ([CO2]atm) on the carbon cycle, which in turn drives changes in the global climate, is of the highest importance. Global climate models are used to project changes to biogeochemical cycling and the climate system caused by this anthropogenic forcing; given that such projections involve multiple processes and complex climate feedbacks, they possess an inherent degree of uncertainty. Consequently, it is essential that numerical climate models employed to analyze the impacts of future climate change be rigorously evaluated for their reliability and consistency with observational data.
One important impact of increasing [CO2]atm is CO2 fertilization, which is defined as the increased uptake of carbon by the terrestrial biosphere through enhanced photosynthesis in response to rising [CO2]atm (Norby et al., Citation2005). This increased uptake of carbon acts as a negative feedback in the terrestrial carbon cycle that has the potential to slow the increase in global [CO2]atm in response to anthropogenic CO2 emissions (Gregory et al., Citation2009; Matthews, Citation2007; Wullschleger et al., Citation1995). The effects of CO2 fertilization can be quantified as a change in net primary productivity (NPP), measured in g C m−2y−1 (Norby et al., Citation2005), which represents the rate of carbon fixation into organic matter (Malhi et al., Citation2002). CO2-induced changes to NPP can be observed and quantified in field studies, called Free-Air Carbon Dioxide Enrichment (FACE) studies, as well as simulated by coupled carbon-climate models. In this regard, NPP is an appropriate variable to use to benchmark such models against field measurements from FACE experiments.
One of the primary focuses of CO2 fertilization research has been forest ecosystems because these ecosystems alone contribute 70–80% of the total global terrestrial NPP (Hamilton et al., Citation2002; Karnosky, Citation2003; Matthews, Citation2007; Norby et al., Citation2005). In addition, a number of FACE studies have also been conducted in grassland ecosystems (Nowak et al., Citation2004). It has been argued that increased carbon uptake of temperate forests resulting from elevated [CO2]atm may account for a significant portion of the current imbalance in the global carbon budget which, after all known carbon sinks are subtracted from all known carbon sources, is estimated to be 2.8 Gt C y−1 (DeLucia et al., Citation2005).
Since increasing [CO2]atm is projected to result in increasing carbon uptake by the terrestrial biosphere, the magnitude of this uptake represents a key area of uncertainty in climate model projections (Matthews, Citation2007). However, the exact nature and magnitude of the impacts of CO2 fertilization on plant physiological processes also remains unclear and is the focus of an active body of research (see reviews by Drake et al. Citation(1997); Körner Citation(2000); Karnosky Citation(2003)). CO2 enrichment studies, usually involving an artificial increase in [CO2]atm to approximately 550 parts per million (ppm), have been conducted in various ecosystems and on various vegetation types. A meta-analysis of 104 such studies conducted by Luo et al. Citation(2006) confirmed an overall increase in terrestrial carbon uptake in response to elevated [CO2]atm but this response was shown to be highly variable across ecosystems and between plant species. Studies conducted in forest ecosystems by DeLucia et al. Citation(1999), Hamilton et al. Citation(2002), Gielen et al. Citation(2005) and Norby et al. Citation(2005), under a [CO2]atm range of 550–580 ppm, showed NPP increases of 25%, 27%, 26% and 23 ±2%, respectively. In addition to showing NPP increases of 16–38% for loblolly pine plots and 14-26% for deciduous sweetgum forest plots in response to elevated [CO2]atm, an analysis conducted by DeLucia et al. Citation(2005) also demonstrated that the two species allocated the additional carbon to different tissues. Other studies have examined the role of nitrogen limitation (Luo et al., Citation2006; Wullschleger et al., Citation1995) and increased temperature (Luo, Citation2007) in CO2 fertilization but there is little consensus among the results and therefore further research is required in these areas.
A number of comparison studies have been published in the literature examining both simulated and observed increases in NPP due to elevated [CO2]atm. Matthews Citation(2007) and Hickler et al. Citation(2008) evaluated projected increases in global NPP, using the University of Victoria's Earth System Climate Model (UVic ESCM version 2.7; based on Weaver et al., Citation2001) and the Lund-Potsdam-Jena General Ecosystem Simulator (LPJ-GUESS) models, respectively, at a [CO2]atm of 550 ppm. The simulated increases in overall terrestrial NPP were 24.6% (Matthews, Citation2007) and 24.5 ±0.06% (Hickler et al., Citation2008) which agreed with the 23 ±2% increase in NPP observed by Norby et al. Citation(2005). These model results, however, are on a global scale while the FACE results of Norby et al. Citation(2005) are specific to the temperate forest biome. Hickler et al. Citation(2008) took their study one step further and analyzed the simulated NPP enhancement geographically. Results revealed that boreal forests had an NPP increase of 15.1 ±0.06%; temperate forests had an increase of 25.7 ±0.14%; and tropical forests had an increase of 35.1 ± 0.09% suggesting that FACE studies were not representative of the tropical forest or boreal forest biome (Hickler et al., Citation2008).
The purpose of this study is to expand on the work of Matthews Citation(2007) and Hickler et al. Citation(2008) by evaluating the performance of an intermediate complexity coupled climate-carbon model in simulating the effect of CO2 fertilization on forest NPP, compared to changes in NPP observed during FACE field studies in the temperate forest biome. We made a direct comparison between the observed increase in NPP and that simulated by the model in a latitudinal band that geographically matched the spatial distribution of the FACE field studies. We further examined the simulated results on a global scale in order to isolate any geographic variations in NPP enhancement and variations among different vegetation types. We determined the impact of climate change on CO2 fertilization by comparing simulations with and without the effects of climate change (i.e., changes in temperature and precipitation due to elevated CO2). Finally, we expanded our analysis to assess the range of results from other coupled climate-carbon models that participated in the Coupled Climate-Carbon Cycle Model Intercomparison Project (C4MIP) (Friedlingstein et al., Citation2006).
2 Materials and methods
a Model Description
The UVic ESCM version 2.8 is an intermediate complexity global climate model with a grid resolution of 1.8˚ latitude by 3.6˚ longitude. The climate component of the model consists of a simplified energy-moisture balance atmospheric model with dynamical feedbacks (Weaver et al., Citation2001) that is further coupled to a land-surface and dynamic-vegetation model (Meissner et al., Citation2003), a terrestrial carbon cycle model (Matthews et al., Citation2005) and an ocean carbon cycle model (Schmittner et al., Citation2008).
The dynamic Top-down Representation of Interactive Foliage and Flora Including Dynamics (TRIFFID) vegetation model simulates the terrestrial biosphere in terms of soil carbon as well as the structure and spatial coverage of the five plant functional types (PFT): broadleaf trees, needleleaf trees, C3 grasses, C4 grasses and shrubs (Cox, Citation2001). The land-surface model is a simplified single soil layer version of The Met Office Surface Exchange Scheme (MOSES2) which simulates the terrestrial carbon cycle and allows TRIFFID to use this simulated carbon availability to define vegetation distributions (Cox, Citation2001; Meissner et al., Citation2003). The effects of CO2 fertilization are calculated within this component's photosynthesis module where photosynthesis is calculated as a function of CO2, light, soil moisture, temperature and nutrients, using a leaf-level model for C3 and C4 plants (Collatz et al., Citation1991, Citation1992) with coupled photosynthesis and stomatal conductance (Cox et al., Citation1999). Using the accumulated carbon fluxes passed from MOSES2, TRIFFID updates the vegetation and soil carbon on a monthly time scale. The vegetation dynamics module in TRIFFID also updates the fractional areal coverage of each PFT based on Lotka-Volterra competition equations. Carbon is passed to a single soil carbon pool through litterfall and vegetation mortality while soil carbon decomposition is controlled by soil temperature (using a Q10 formulation) and water stress. The model, however, does not dynamically couple the terrestrial carbon and nitrogen cycles. Nitrogen availability is instead prescribed as a fixed carbon to nitrogen ratio (C:N).
b FACE Experimental Data
As seen in , we compared the model results with FACE studies from Duke University (DukeFACE), the US Department of Energy (AspenFACE), and the Oak Ridge National Laboratory (ORNL-FACE) located in Durham North Carolina (35°58'N, 79°05'W), Rhinelander Wisconsin (45°40'N, 89°37'W) and Oak Ridge Tennessee (35°54'N, 84°20'W), respectively, as well as the POP-EUROFACE experiment located in Viterbo, Italy (42°22'N, 11°48'E) (Norby et al., Citation2005). The set-up at these four temperate forest locations involved exposing the experimental forest plots to CO2 concentrations of 550 ppm, without controlling for factors such as temperature and moisture, while control plots were simultaneously exposed to ambient CO2 levels. The NPP in the four studies was calculated as annual wood, leaf, coarse root and fine root carbon increments, respectively, along with major inputs to detritus, litterfall and fine root turnover. The average change in NPP (23 ±2%) across these four FACE sites reported by Norby et al. Citation(2005), used here as the basis for comparison to model results, was based on data collected over the years 1999 to 2003 from ORNL-FACE; 1997 to 2002 from DukeFACE; 2003 Aspen data, and 2001 to 2003 Aspen-birch data from AspenFACE; and 2000 to 2001 data from POP-EUROFACE (Norby et al., Citation2005).
Fig. 1 Map depicting selected latitudinal range and the geographic location of the four FACE field studies in Norby et al. Citation(2005): (1) AspenFACE, (2) ORNL-FACE, (3) DukeFACE and (4) POP-EUROFACE.
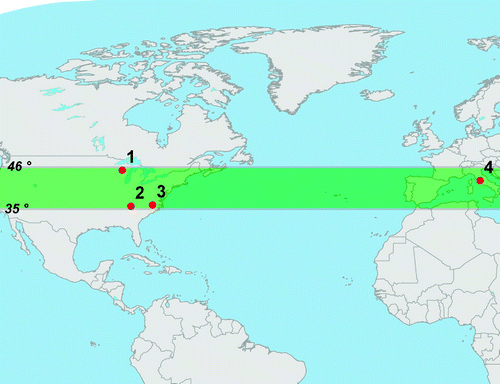
c Model Experimental Methodology
A six-year time frame from 1997 to 2002 inclusive was selected for the model simulations in this study in order to represent best the overall data collection time frame used by Norby et al. Citation(2005). The UVic ESCM was initialized to 1997 climatic conditions by beginning from a simulation equilibrated to pre-industrial climatic conditions and integrating the model forward in time to the end of 1996. Three simulations were run over the six-year period from 1997 to 2002. The first was a control simulation which was run using prescribed observed [CO2]atm data from 1997 to 2002. The second, CO2_CC, was an experimental simulation where [CO2]atm was increased to 550 ppm in 1997 and kept constant throughout the six years of the simulation. The third, CO2_NCC, was an experimental simulation with the same climate conditions as the control simulation but with carbon availability in the terrestrial model set to an atmospheric CO2 equivalent of 550 ppm, thus simulating CO2 fertilization without the additional effects of climate change from elevated CO2. The percentage increase in NPP in the experimental simulations relative to the control was calculated using the six-year average for each simulation (1997–2002).
The coarseness of the spatial model resolution and the lack of simulated forest at the geographic coordinates of some of the FACE forest plots prevented a site-level comparison between projected and observed changes in NPP. Therefore, in addition to analyzing the global model output, we isolated the latitudinal range between 35°N and 46°N in order to represent best the temperate forest region where the four Norby et al. Citation(2005) FACE studies were located (). The simulated NPP enhancement for other plant functional types (C3 grasses, C4 grasses and shrubs) was also assessed in order to identify any general trends in the response of non-forest plant functional types compared to changes in forest productivity. Finally, we compared the global scale results simulated by the UVic ESCM to the range of results available from models participating in C4MIP, by calculating the percentage increase in NPP for each model at the year in which [CO2]atm reached 550 ppm relative to NPP at present-day [CO2]atm.
3 Results
Using observed atmospheric CO2 data for the years 1997–2002 inclusive the UVic ESCM simulated a gradual increase in NPP both globally and across all tree PFTs over the course of the model run (control simulation). Global NPP increased from 98.29 g C m−2 y−1 in 1997 to 99.49 g C m−2 y−1 in 2002, representing a 1.2% increase. Globally averaged forest-only NPP increased from 281.50 g C m−2y−1 in 1997 to 286.3 g C m−2y−1 in 2002, representing a 1.7% increase while, between 35°N and 46°N, forest-only NPP increased from 143.02 g C m−2 y−1 in 1997 to 145.10 g C m−2 y−1 in 2002, representing a 1.5% increase.
shows the projected spatial distributions of NPP changes for each PFT recognized by TRIFFID, in addition to combined forests (broadleaf trees plus needleleaf trees) following an instantaneous increase in [CO2]atm to a constant level of 550 ppm in 1997 (run CO2_CC, compared to the control run). The NPP for all PFTs increased in response to elevated CO2, with the exception of C4 grasses, whose NPP decreased slightly over the six years of the simulation. The global NPP increase was clearly dominated by the response of tropical plant functional types; specifically, changes in forest NPP were largely a result of enhanced productivity by broadleaf trees in the tropics. Globally averaged NPP for all plant functional types increased by 24.5%, which is consistent with the FACE results for mid-latitude temperate forests (23 ±2% NPP increase) reported by Norby et al. Citation(2005), as well as with the 24.6% increase in overall terrestrial NPP obtained by Matthews Citation(2007) using version 2.7 of the UVic ESCM.
Fig. 2 Distribution of the average NPP increase between 1997 and 2002 under a [CO2]atm of 550 ppm for a) all forests, b) broadleaf trees, c) needleleaf trees, d) C3 grasses, e) C4 grasses and f) shrubs. The latitudinal band between 35°N and 46°N is indicated by the grey shaded area.
![Fig. 2 Distribution of the average NPP increase between 1997 and 2002 under a [CO2]atm of 550 ppm for a) all forests, b) broadleaf trees, c) needleleaf trees, d) C3 grasses, e) C4 grasses and f) shrubs. The latitudinal band between 35°N and 46°N is indicated by the grey shaded area.](/cms/asset/51aa5a72-2426-475f-a7a4-e3c4f6b69b67/tato_a_554784_o_f0002g.jpg)
shows the projected spatial distributions of NPP increases at 550 ppm [CO2]atm in the absence of associated climate changes from elevated CO2 (run CO2_NCC, compared to the control run). In this case also, the UVic ESCM simulated increased NPP for all PFTs except C4 grasses. The spatial pattern of NPP changes was broadly similar to the climate change simulation (CO2_CC), though NPP increases in CO2_NCC were notably larger in the tropics and lower at high latitudes. This highlights an important difference in the temperature sensitivity of vegetation at different latitudes. Tropical NPP is clearly subject to greater heat stress at higher temperatures; hence, climate warming in CO2_CC served to suppress the tropical CO2 fertilization response. By contrast, at higher latitudes, climate warming led to decreased temperature limitation of NPP, with the result that the CO2 fertilization response was larger in CO2_CC.
Fig. 3 Distribution of average NPP increases between 1997 and 2002 with carbon availability equivalent to a [CO2]atm of 550 ppm and excluding the effects of climate change for a) all forests, b) broadleaf trees, c) needleleaf trees, d) C3 grasses, e) C4 grasses and f) shrubs. The latitudinal band between 35°N and 46°N is indicated by the grey shaded area.
![Fig. 3 Distribution of average NPP increases between 1997 and 2002 with carbon availability equivalent to a [CO2]atm of 550 ppm and excluding the effects of climate change for a) all forests, b) broadleaf trees, c) needleleaf trees, d) C3 grasses, e) C4 grasses and f) shrubs. The latitudinal band between 35°N and 46°N is indicated by the grey shaded area.](/cms/asset/e2a6f731-bb4f-4e1b-8c78-790d319c2570/tato_a_554784_o_f0003g.jpg)
Spatially averaged global NPP increases due to elevated CO2 for each PFT are presented in , with and without the effects of climate change. The black horizontal lines show the range of NPP increases reported by the FACE studies of Norby et al. Citation(2005). The globally averaged NPP increase in CO2_CC (24.5%) agrees well with the observed NPP increase of 23 ±2% by Norby et al. Citation(2005), but when the effects of climate change were excluded, NPP increased by 27%, which is greater than the higher limit of this range. Similarly, the global NPP increase for forest-only PFTs was 27.9% in CO2_CC and 30.6% in CO2_NCC. This increased CO2 response in the absence of climate change was largely due to reduced heat stress in tropical broadleaf trees and C3 grasses; the NPP change for broadleaf trees increased from 32.9% in CO2_CC to 37.4% in CO2_NCC and for C3 grasses, from 29.4% to 38.3%. By contrast, needleleaf tree NPP increased by 15.6% in CO2_CC but by only 13.8% in CO2_NCC. The NPP increase for shrubs showed very little response to climate change (24.2% increase in CO2_CC and 24.8% in CO2_NCC), and the NPP of C4 grasses decreased in both cases (17.2% in CO2_CC and 20.7% in CO2_NCC). The response of C4 grasses compared to C3 grasses indicates a competitive disadvantage of C4 relative to C3 grasses at high concentrations of CO2, which is only slightly mediated by climate warming. This competitive disadvantage is consistent with differences in photosynthetic and resource use efficiency enhancements between C3 and C4 PFTs under elevated CO2 (Drake et al., Citation1997).
Fig. 4 Average percentage global NPP increase of recognized PFTs between 1997 and 2002 at a [CO2]atm of 550 ppm and the C4MIP range of model NPP responses with (black) and without (grey) climate change. The black horizontal lines represent the maxima and minima of the Norby et al. Citation(2005) NPP response range of 21.0% to 25.0%.
![Fig. 4 Average percentage global NPP increase of recognized PFTs between 1997 and 2002 at a [CO2]atm of 550 ppm and the C4MIP range of model NPP responses with (black) and without (grey) climate change. The black horizontal lines represent the maxima and minima of the Norby et al. Citation(2005) NPP response range of 21.0% to 25.0%.](/cms/asset/44a36c4b-5308-4676-9199-c6aa24c84750/tato_a_554784_o_f0004g.gif)
Also shown in , is the range of results from other coupled climate-carbon models that participated in the C4MIP study (Friedlingstein et al., Citation2006). The range for the overall terrestrial NPP increase, plotted as the “C4MIP range”, was calculated from the Friedlingstein et al. Citation(2006) coupled and uncoupled simulations, as the percentage NPP increase at the year in the transient model simulations when CO2 reached 550 ppm relative to the year when CO2 was 269 ppm. The vertical lines show the range of the results from nine inner models, with the dots (and numbers) showing the upper and lower outliers. As is clear from this analysis, there is little agreement between models as to the globally averaged NPP response to elevated CO2. In general, NPP increased more when climate change was excluded (“uncoupled” simulations) than when climate change was included (“coupled” simulations), though there were also some exceptions to this rule (see also Friedlingstein et al., Citation2006). However, as can be inferred from the analysis of the UVic ESCM model results, there is a large amount of variability amongst plant types within a given model, and it is therefore not possible to make any robust conclusions from a comparison of globally averaged model NPP increases to regionally specific forest FACE study results.
In , we show NPP increases for each PFT but averaged only over the latitudes between 35°N and 46°N (indicated by the shaded region on , and 3), selected to best represent the location of the forest FACE experiments. For this region also, NPP values averaged across all PFTs were consistent with the Norby et al. Citation(2005) FACE data (21% increase in CO2_CC and 24.5% increase in CO2_NCC). However, forest-only NPP increased by only 17.2% in CO2_CC and by 14.4% in CO2_NCC; in this case, NPP increases were notably smaller than the FACE data range. As in the case of the global averages, broadleaf tree NPP increased less in CO2_CC (24.5%) than in CO2_NCC (26.2%), whereas the opposite was true for needleleaf trees (16.2% increase in CO2_CC and 13.1% increase in CO2_NCC). Similar differences between the two simulations were seen for other PFTs at the latitudinal scale.
Fig. 5 Average percentage NPP increase of recognized plant functional types between 1997 and 2002 at a [CO2]atm of 550 ppm with (black) and without (grey) climate change but only between 35°N and 46°N latitude (1997–2002). The black horizontal lines represent the Norby et al. Citation(2005) NPP response range of 21.0% to 25.0%.
![Fig. 5 Average percentage NPP increase of recognized plant functional types between 1997 and 2002 at a [CO2]atm of 550 ppm with (black) and without (grey) climate change but only between 35°N and 46°N latitude (1997–2002). The black horizontal lines represent the Norby et al. Citation(2005) NPP response range of 21.0% to 25.0%.](/cms/asset/3bc6f6c3-746d-4a8e-9659-63d5aa6d669e/tato_a_554784_o_f0005g.gif)
4 Discussion
Our comparative analysis of the four FACE field studies by Norby et al. Citation(2005) and the simulated FACE studies conducted using the UVic ESCM has yielded three main findings. First, results suggest that when considering the simulated forest NPP response in the region consistent with the FACE experimental data, the model underestimated the CO2 fertilization response of temperate forests. Second, the effects of climate change, including elevated temperature and changes in precipitation patterns, led to a suppressed NPP response for broadleaf trees and C3 grasses (and for tropical productivity generally) but an enhanced NPP response for needleleaf trees (and high-latitude productivity). Globally, including climate change led to a reduced NPP response to elevated CO2, but in the temperate forest latitude band, including climate change led to an enhanced response. Third, the differences in NPP enhancement between broadleaf (tropical) trees and needleleaf (temperate/boreal) trees suggest that despite the large expanse of the temperate forest across continents, the temperate forest FACE results alone are not an appropriate metric with which to assess global-scale NPP increases from coupled climate-carbon models.
In general, the geographic distributions of the different PFTs such as broadleaf trees and needleleaf trees simulated by the UVic ESCM were consistent with known global distributions of forested regions (Meissner et al., Citation2003). Simulated needleleaf NPP was highest in the mid-latitude forests of North America and Asia, with a noticeable distribution also in the northern latitude boreal forests. Broadleaf trees dominated tropical forests and the model projected very little broadleaf tree distribution in temperate and high-latitude regions. It is possible that our conclusion that the model under-projects the CO2 fertilization response was influenced by the model's imperfect balance of needleleaf and broadleaf trees in the specific latitudinal band that we selected to match the location of the FACE experiments. However, we did test the sensitivity of our results to the selected region by analyzing several other geographic regions, including all extra-tropical areas in each hemisphere as well as individual continents. Despite the sparse spatial distribution of broadleaf trees compared to needleleaf trees simulated in these regions, the contribution of broadleaf trees to the projected increase in NPP varied considerably between regions, with broadleaf trees contributing only up to 19.1% of the increase in extra-tropical forests of the northern hemisphere but up to 90.6% of the increase in the extra-tropical forests of the southern hemisphere. Nevertheless, the overall NPP response of extra-tropical forests was consistently lower than the 23 ±2% increase reported by Norby et al. Citation(2005), suggesting that our conclusion is not an artifact of the specific mix of simulated forest cover within the geographic region that we selected. In addition to this bias in the PFT balance, the lack of additional anthropogenic influences, such as anthropogenic nitrogen, as well as a highly simplified representation of several key vegetative demographic processes (e.g., seed advection, seed mixing and sapling survival) may also have contributed to the model's under-projection of the forest CO2 fertilization response. Such factors can affect both predicted vegetation community structure and the response of ecosystems to climate change (Fisher et al., Citation2010). These processes, however, are the focus of ongoing model development and are beyond the scope of this study.
A major limitation of many forest FACE field studies is the omission of the effects of CO2-induced climate change in the experimental set-up because artificial changes are not made to climate variables such as temperature and precipitation when CO2 levels are increased in the experimental vegetation plots (Nowak et al., 2003). As we have shown here, temperature increases can have an important influence on the CO2 fertilization response in the model; additionally, both the magnitude and sign of the effect are dependent on vegetation type and latitude. Tropical broadleaf tree and C3 grass NPP were negatively affected by temperature increases, whereas mid- and high-latitude needleleaf forest NPP values were enhanced by warmer temperatures. Globally, NPP increases due to CO2 fertilization were smaller when climate change was included, both in the UVic ESCM projections of CO2-induced NPP change and in the analysis of the transient C4MIP simulations (Friedlingstein et al., Citation2006); this is consistent with previous studies which have shown that the sensitivity of tropical NPP to high temperatures is a key determinant of net terrestrial carbon uptake in response to elevated CO2 and climate changes over the next century (Matthews et al., Citation2007; Raddatz et al., Citation2007). However, the opposite was true in mid-latitude forests, where higher temperatures led to a larger NPP increase; this indicates that the CO2 fertilization response inferred from temperate forest FACE studies may have a very different sensitivity to temperature increases than that of globally averaged NPP.
In addition to different temperature sensitivities, different plant types in our simulations had very different responses to elevated CO2 alone. Notably, tropical broadleaf trees had a much higher response to CO2 fertilization than did temperate/boreal needleleaf trees. This is broadly consistent with the result reported by Hickler et al. Citation(2008) who argued that temperate forest FACE experiments are not consistent with either tropical or boreal forest ecosystem responses to elevated CO2 in a study using an offline version of the LPJ-GUESS model. Despite Hickler et al.'s use of a very different model and experimental set-up, as well as the omission of the effects of CO2-induced climate change, there are several important similarities between the results we present here and those of Hickler et al. Citation(2008). Notably, both models simulated global broadleaf tree/tropical forest NPP increases greater than 32% and global needleleaf tree/boreal forest NPP increases of less than 16%, which fall above and below the FACE field results, respectively. Both models also agree that global forest NPP is projected to increase by at least 24.5% at a [CO2]atm of 550 ppm. However, Hickler et al. Citation(2008) reported that the LPJ-GUESS temperate forest response was consistent with the FACE data, whereas in our study, the CO2 fertilization effect in extra-tropical forests is consistently weaker than that found by Norby et al. Citation(2005). Despite this difference, the NPP increases simulated by the UVic ESCM support the general conclusion of Hickler et al. Citation(2008) that temperate forest FACE results are not representative of all forest regimes and cannot therefore be applied globally.
As we have begun to outline here, experimental FACE studies, like models, are subject to their own limitations and uncertainties. This stems not only from technological limitations in creating controlled microclimates on the scale of the FACE studies but also gaps in our knowledge of plant physiological responses to high CO2 concentrations. For example, CO2-induced stimulation of photosynthetic rates may not be directly linked to NPP (Körner, Citation2003); trees growing in natural settings may not be carbon limited since they are presently growing at CO2 levels that are approximately double what they were 18 000 years ago (Neftel et al., Citation1988; Gedalof and Berg, Citation2010); and gradual long-term increases in [CO2]atm may have different impacts than short-term step increases (Körner, Citation2003). Nevertheless, these uncertainties are the focus of an active body of research and FACE studies currently remain one of the best ways to examine CO2-induced changes in real-world ecosystems.
It is also worth noting that while we have focused here on the modelled forest CO2 fertilization response, there is also a rich body of literature on the response of grassland ecosystems to elevated CO2. Grassland FACE studies frequently use higher [CO2]atm than do the forest FACE studies that we have discussed here and are typically multifactor experiments which include nutrient availability and species richness in addition to elevated CO2. The closest match that we found to the experimental protocol used by Norby et al. Citation(2005) is the study by Reich et al. Citation(2001), who reported an increase in average total biomass of 27.0% in a grassland FACE study conducted at doubled present-day [CO2]atm using experimental plots which included C3 grasses, C4 grasses, C3 legumes and C3 non-legumes. In our simulations the largest biomass increase simulated by grass plant functional types was a 12.3% increase in average global biomass for C3 grasses in the CO2_NCC simulation. While not a perfect comparison, these results add some additional support to our conclusion that the UVic ESCM specifically, and climate-carbon models more generally, may underestimate increased carbon uptake under elevated CO2 conditions.
5 Conclusions
CO2 fertilization is recognized as an integral part of the terrestrial carbon cycle and an important factor in determining future [CO2]atm in a world of ever increasing anthropogenic CO2 emissions. Many studies have analyzed the impacts of CO2 fertilization on terrestrial vegetation, such as the enhancement of net primary productivity (NPP), through both FACE field studies and modelling studies (DeLucia et al., Citation1999; Cramer et al., Citation2001; Hamilton et al., Citation2002; Nowak et al., Citation2004; Gielen et al., Citation2005). Our research has expanded on previous modelling studies by comparing the simulated temperate forest NPP enhancement in a coupled climate-carbon model to experimental data from forest FACE field studies (Norby et al., Citation2005). We used two model scenarios to compare the effect of elevated [CO2]atm without climate change to the effect of elevated [CO2]atm with climate change. We further compared the model's global-scale forest NPP response with the response simulated in temperate, tropical and boreal forest biomes.
By conducting a simulated FACE study using the climate-carbon model and comparing the resulting model projections of forest NPP increases to the average NPP enhancement of 23 ±2% reported by Norby et al. Citation(2005), we have shown that the model results are not consistent with the observed response of temperate forest NPP in either the CO2_CC or the CO2_NCC model scenarios. Specifically, we have shown that when the closest possible comparison of the model results to the FACE data is performed, the model consistently simulated less NPP increase from CO2 fertilization than was reported by Norby et al. Citation(2005). At a global scale, climate change tended to suppress the response of NPP to elevated CO2, which can also be seen in the results from other coupled climate-carbon models (Friedlingstein et al., Citation2006). However, this global signal was dominated by the response of tropical forest NPP in the model, whereas forest NPP at temperate and high latitudes increased more when climate warming was included in the simulations. In general, there was a wide range of responses associated with different PFTs in the model. The divergent CO2 fertilization response of tropical forests compared to forests at temperate and high latitudes supports the growing body of evidence in the literature that FACE studies, currently limited to the temperate forest biome, are not representative of tropical and boreal forest biomes (e.g., Hickler et al., Citation2008). Based on our analysis of the response of different vegetation types and geographic regions to elevated CO2, we suggest that validating models using global variables will mask important geographic variations in NPP enhancement and temperature stress, variations in the responses between vegetation types to CO2 fertilization and any changes these variations may exhibit through time.
Acknowledgements
This study was funded by HDM's Nouveaux Chercheurs grant from the Fonds Québécois de la Recherche sur la Nature et la Technologie, the Natural Sciences and Engineering Research Council of Canada and the Canadian Foundation for Climate and Atmospheric Sciences.
References
- Collatz , G. J. , Ball , J. T. , Grivet , C. and Berry , J. A. 1991 . Physiological and environmental–regulation of stomatal conductance, photosynthesis and transpiration - A model that includes a laminar boundary-layer . Agric. For. Meteorol. , 54 : 107 – 136 .
- Collatz , G. J. , Ribas-Carbo , M. and Berry , J. A. 1992 . Coupled photosynthesis-stomatal conductance model for leaves of C4 plants . Aust. J. Plant Physiol. , 19 : 519 – 538 .
- Cox , P. M. 2001 . Description of the “TRIFFID” dynamic global vegetation model (Hadley Centre Technical Note 24), Bracknell, UK: Met Office
- Cox , P. M. , Betts , R. A. , Bunton , C. B. , Essery , R. L.H. , Rowntree , P. R. and Smith , J. 1999 . The impact of new land surface physics on the GCM simulation of climate and climate sensitivity . Clim. Dyn. , 15 : 183 – 203 .
- Cramer , W. , Bondeau , A. , Woodward , F. I. , Prentice , I. C. , Betts , R. A. , Brovkin , V. , Cox , P. M. , Fisher , V. , Foley , J. A. , Friend , A. D. , Kucharik , C. , Lomas , M. R. , Ramankutty , N. , Sitch , S. , Smith , B. , White , A. and Young-Molling , C. 2001 . Global response of terrestrial ecosystem structure and function to CO2 and climate change: Results from six dynamic global vegetation models . Glob. Change Biol. , 7 ( 4 ) : 357 – 373 .
- Delucia , E. H. , Hamilton , J. G. , Naidu , S. L. , Thomas , R. B. , Andrews , J. A. , Finzi , A. , Lavine , M. , Matamala , R. , Mohan , J. E. , Hendrey , G. R. and Schlesinger , W. H. 1999 . Net primary production of a forest ecosystem with experimental CO2 enrichment . Science , 284 ( 5417 ) : 1177 – 1179 .
- Delucia , E. H. , Moore , D. J. and Norby , R. J. 2005 . Contrasting responses of forest ecosystems to rising atmospheric CO2: Implications for the global C cycle . Global Biogeochem. Cy. , 19 ( 3 ) GB3006, doi: 10.1029/2004GB002346
- Drake , B. G. , Gonzàlez-Meler , M. A. and Long , S. P. 1997 . More efficient plants: A consequence of rising atmospheric CO2? . Annu. Rev. Plant Physiol. Plant Mol. Biol. , 48 ( 1 ) : 609 – 639 .
- Fisher , R. , Mcdowell , N. , Purves , D. , Moorcroft , P. , Sitch , S. , Cox , P. , Huntingford , C. , Meir , P. and Woodward , F. I. 2010 . Assessing uncertainties in a second-generation dynamic vegetation model caused by ecological scale limitations . New Phytol. , 187 ( 3 ) : 666 – 681 .
- Friedlingstein , P. , Cox , P. , Betts , R. , Bopp , L. , von Bloh , W. , Brovkin , V. , Cadule , P. , Doney , S. , Eby , M. , Fung , I. , Bala , G. , John , J. , Jones , C. , Joos , F. , Kato , T. , Kawamiya , M. , Knorr , W. , Lindsay , K. , Matthews , H. D. , Raddatz , T. , Rayner , P. , Reik , C. , Roeckner , E. , Schnitzler , K.-G. , Schnur , R. , Strassmann , K. , Weaver , A. J. , Yoshikawa , C. and Zeng , N. 2006 . Climate-carbon cycle feedback analysis: Results from the C4MIP model intercomparison . J. Clim. , 19 ( 14 ) : 3337 – 3353 .
- Gedalof , Z. and Berg , A. A. 2010 . Tree ring evidence for limited direct CO2 fertilization of forests over the 20th century . Global Biogeochem. Cy. , 24 GB3027, doi:10.1029/2009GB003699
- Gielen , B. , Calfapietra , C. , Lukac , M. , Wittig , V. E. , De Angelis , P. , Janssens , I. A. , Moscatelli , M. C. , Grego , S. , Cotrufo , M. F. , Godbold , D. L. , Hoosbeek , M. R. , Long , S. P. , Miglietta , F. , Polle , A. , Bernacchi , C. J. , Davey , P. A. , Ceulemans , R. and Scarascia-Mugnozza , G. E. 2005 . Net carbon storage in a poplar plantation (POPFACE) after three years of free-air CO2 enrichment . Tree Physiol. , 25 ( 11 ) : 1399 – 1408 .
- Gregory , J. M. , Jones , C. D. , Cadule , P. and Friedlingstein , P. 2009 . Quantifying carbon cycle feedbacks . J. Clim. , 22 ( 19 ) : 5232 – 5250 .
- Hamilton , J. G. , Delucia , E. H. , George , K. , Naidu , S. L. , Finzi , A. C. and Schlesinger , W. H. 2002 . Forest carbon balance under elevated CO2 . Oecologia , 131 ( 2 ) : 250 – 260 .
- Hickler , T. , Smith , B. , Prentice , I. C. , Mjofors , K. , Miller , P. , Arneth , A. and Skyes , T. 2008 . CO2 fertilization in temperate FACE experiments not representative of boreal and tropical forests . Glob. Change Biol. , 14 ( 7 ) : 1531 – 1542 .
- Karnosky , D. F. 2003 . Impacts of elevated atmospheric CO2 on forest trees and forest ecosystems: Knowledge gaps . Environ. Int. , 29 ( 2–3 ) : 161 – 169 .
- Körner , C. 2000 . Biosphere responses to CO2 enrichment . Ecol. Appl. , 10 ( 6 ) : 1590 – 1619 .
- Körner , C. 2003 . Carbon limitation in trees . J. Ecol. , 91 ( 1 ) : 4 – 17 .
- Luo , Y. 2007 . Terrestrial carbon-cycle feedback to climate warming . Ann. Rev. Ecol. Evol. and Syst. , 38 : 683 – 712 .
- Luo , Y. Q. , Hui , D. F. and Zhang , D. Q. 2006 . Elevated CO2 stimulates net accumulations of carbon and nitrogen in land ecosystems: A meta-analysis . Ecology , 87 ( 1 ) : 53 – 63 .
- Malhi , Y. , Meir , P. and Brown , S. 2002 . Forests, carbon and global climate . Philos. T. Roy. Soc. A. , 360 : 1567 – 1591 .
- Matthews , H. D. 2007 . Implications of CO2 fertilization for future climate change in a coupled climate-carbon model . Glob. Change Biol. , 13 ( 5 ) : 1068 – 1078 .
- Matthews , H. D. , Weaver , A. J. and Meissner , K. J. 2005 . Terrestrial carbon cycle dynamics under recent and future climate change . J. Clim. , 18 : 1609 – 1628 .
- Matthews , H. D. , Eby , M. , Ewen , T. , Friedlingstein , P. and Hawkins , B. J. 2007 . What determines the magnitude of carbon cycle-climate feedbacks? . Global Biogeochem. Cy. , 21 GB2012, doi:10.1029/2006GB002733
- Meissner , K. J. , Weaver , A. J. , Matthews , H. D. and Cox , P. M. 2003 . The role of land-surface dynamics in glacial inception: a study with the UVic Earth System Climate Model . Clim. Dynam. , 21 : 515 – 537 .
- Neftel , A. , Oeschger , H. , Staffelbach , T. and Stauffer , B. 1988 . CO2 record in the Byrd ice core 50,000–5,000 years BP . Nature , 331 ( 6157 ) : 609 – 611 .
- Norby , R. J. , Delucia , E. H. , Gielen , B. , Calfapietra , C. , Giardina , C. P. , King , J. S. , Ledford , J. , McCarthy , H. R. , Moore , D. J.P. , Ceulemans , R. , De Angelis , P. , Finzi , A. C. , Karnosky , D. F. , Kubiske , M. E. , Lukac , M. , Pregitzer , K. S. , Scarascia-Mugnozza , G. E. , Schlesinger , W. H. and Oren , R. 2005 . Forest response to elevated CO2 is conserved across a broad range of productivity . P. Natl. Acad. Sci. USA , 102 ( 50 ) : 18052 – 18056 .
- Nowak , R. S. , Ellsworth , D. S. and Smith , S. D. 2004 . Functional responses of plants to elevated atmospheric CO2 – Do photosynthetic and productivity data from FACE experiments support early predictions? . New Phytol. , 162 ( 2 ) : 253 – 280 .
- Raddatz , T. J. , Reick , C. H. , Knorr , J. , Kattge , J. , Roeckner , E. , Schnur , R. , Schnitzler , K.-G. , Wetzel , P. and Jungclaus , J. 2007 . Will the tropical land biosphere dominate the climate-carbon cycle feedback during the twenty-first century? . Clim. Dyn. , 29 : 565 – 574 .
- Reich , P. B. , Tilman , D. , Craine , J. , Ellsworth , D. , Tjoelker , M. G. , Knops , J. , Wedin , D. , Naeem , S. , Bahauddin , D. , Goth , J. , Bengtson , W. and Lee , T. D. 2001 . Do species and functional groups differ in acquisition and use of C, N and water under varying atmospheric CO2 and N availability regimes? A field test with 16 grassland species . New Phytol. , 150 : 435 – 448 .
- Schmittner , A. , Oschlies , A. , Matthews , H. D. and Galbraith , E. D. 2008 . Future changes in climate, ocean circulation, ecosystems, and biogeochemical cycling simulated for a business-as-usual CO2 emission scenario until year 4000 AD . Global Biogeochem. Cy. , 22 ( 1 ) GB002953, doi:10.1029/2007GB002953
- Weaver , A. J. , Eby , M. , Wiebe , E. C. , Bitz , C. M. , Duffy , P. B. , Ewen , T. L. , Fanning , A. F. , Holland , M. M. , MacFadyen , A. , Matthews , H. D. , Meissner , K. J. , Saenko , O. , Schmittner , A. , Wang , H. and Yoshimori , M. 2001 . The UVic Earth System Climate Model: Model description, climatology and applications to past, present and future climates . Atmosphere-Ocean , 39 : 361 – 428 .
- Wullschleger , S. D. , Post , W. M. and King , A. W. 1995 . “ On the potential for a CO2 fertilization effect in forests: Estimates of the biotic growth factor based on 58 controlled-exposure studies ” . In Biotic Feedbacks in the Global Climate System (pp. 85–107). New York, U.S.A: Oxford University Press.