Abstract
A rainfall simulator was used to evaluate the effects of rainfall intensity and row spacing on Phakopsora pachyrhizi urediniospore wet deposition at different depths within a soybean canopy. Soybean field sections of 19 and 76 cm row spacing were drill planted on two separate dates in Quincy, FL, USA. Urediniospore injected simulated rainfalls of 45 or 85 mm h−1 were conducted within the field sections and urediniospore deposition was quantified in the canopy using a detached leaflet bioassay. The proportion of wet deposited urediniospores retained within the soybean canopy, estimated by uredinia density, was observed to significantly (P < 0.05) decrease with canopy depth. Approximately half of the urediniospores were retained within the upper portion of the soybean canopy, and the other half was distributed between the mid and low canopy sections. Both row spacing and rainfall intensity had no significant (P > 0.10) effect on the vertical distribution of uredinia throughout the soybean canopy, however, more uredinia cm−2 were observed in the upper canopy level for the lower than the higher rainfall intensity. For the treatments examined in this study, it was estimated that between 2.5 and 4.0% of the viable P. pachyrhizi urediniospores that are removed from the atmosphere by rainfall are deposited on and subsequently infect leaves in the mid and low sections of soybean canopies.
Résumé
Un simulateur de pluie a été utilisé pour évaluer les effets de l'intensité des précipitations et de l'espacement des rangs sur le dépôt humide des urédospores de Phakospsora pachyrhizi à différentes profondeurs dans le feuillage de plants du soja. Des sections de champs de soja, dont les rangs étaient espacés de 19 et 76 cm, ont été ensemencées au semoir à Quincy en Floride, É.-U., et ce, à deux dates différentes. Les sections de champs ont reçu la pluie artificielle injectée d'urédospores à raison de 54 ou 85 mm/h, et le dépôt d'urédospores dans le feuillage a été quantifié à l'aide d'un biotest à base de folioles détachées du plant. La proportion d'urédospores déposées par la « pluie » retenue par le feuillage des plants, évaluée en fonction de la densité des urédosores, a décru significativement (P < 0.05), en fonction de la profondeur du feuillage. Environ la moitié des urédospores se sont retrouvées dans la partie supérieure du feuillage des plants tandis que l'autre moitié s'est répartie dans le feuillage du milieu et du bas. L'espacement des rangs et l'intensité de la « pluie » n'ont eu aucun effet significatif (P > 0.10) sur la distribution verticale des urédosores dans le feuillage. Toutefois, les précipitations de faible intensité ont engendré le dépôt de plus d'urédosores par centimètre carré dans la portion supérieure du feuillage que celles de plus forte intensité. Selon les traitements analysés dans cette étude, il a été possible d'évaluer que 2.5 à 4.0 % des urédospores viables de P. pachyrhizi extraites de l'atmosphère par la pluie sont déposées sur le feuillage du milieu et du bas des plants de soja et, subséquemment, infectent les feuilles.
Introduction
Phakopsora pachyrhizi Sydow is a foliar pathogen of soybeans [Glycine max (L.) Merrill] that has spread throughout the world's major production regions, often causing yield reductions (Bromfield, Citation1984; Levy, Citation2005; Yorinori et al., Citation2005; Miles et al., Citation2007). The pathogen is an obligate parasite that cannot survive harsh winters in the major soybean production regions of the central USA. (Thorson, Citation2008). Reports from the US Department of Agriculture's Integrated Pest Management Pest Information Platform for Extension and Education (ipmPIPE) (Isard et al., Citation2006b) and research in Florida and Louisiana (Jurick et al., Citation2008; Park et al., Citation2008) have confirmed that P. pachyrhizi overwinters primarily on kudzu [Pureria montana (Lour) Merr. var. lobata (Willd.) Maessen & S. Almeida] in the Gulf Coast region from Florida to Texas and in Mexico. Each year since its discovery in North America, P. pachyrhizi urediniospores have been transported hundreds of kilometres from southern sources to the Midwest and northern Great Plains regions, where > 80% of the country's soybean production is located (Thorson, Citation2008; Barnes et al., Citation2009). Fortunately, disease development in these regions has occurred late in the growing season (Hartman et al., Citation2007; Li et al., Citation2008a), and thus losses attributed to the pathogen in these regions have been minimal. However, in experimental plots, yield losses as high as 33–35% have been recorded in Georgia and Florida (Kemerait et al., Citation2006; Mueller et al., Citation2008), and a 35% loss on average was reported in Alabama (Sikora et al., Citation2009). It follows that substantial yield reductions could occur within the central USA if viable P. pachyrhizi urediniospores are transported and initiate disease earlier in the growing season (Pivonia & Yang, Citation2006).
The aerial dispersal of P. pachyrhizi urediniospores has been documented at local, regional and global scales (Pivonia & Yang, Citation2004; Isard et al., Citation2005; Krupa et al., Citation2006; Pan et al., Citation2006). In North America, the Integrated Aerobiology Modeling System (IAMS) is used to simulate the aerial dispersal of P. pachyrhizi urediniospores from known sources on a daily basis during the growing season, and the output is used by extension specialists for risk assessment of soybean rust (Isard et al., Citation2006b). In general, the aerial dispersal process of urediniospores can be divided into the three steps of production and escape, horizontal transport, and deposition (Isard & Gage, Citation2001). More information is currently needed about the deposition processes involved in the dispersal, especially long-distance dispersal of P. pachyrhizi urediniospores, to improve the IAMS forecasts and resulting disease management recommendations. Soybean rust infections have been observed to develop first in the middle and bottom sections of soybean canopies (Dorrance et al., Citation2008; Narvaez et al., Citation2010). Quantifying the number of viable urediniospores deposited in a soybean field, and the proportion of those urediniospores that are deposited in environments favourable for infection, are critical factors in predicting the likelihood that soybean rust will establish (Aylor, Citation1999; Isard et al., Citation2005; Del Ponte & Esker, Citation2008).
Urediniospores transported through the atmosphere are deposited onto host surfaces by the processes of gravitational settling and turbulence (dry deposition) and/or rainfall washout (wet deposition) (Gregory, Citation1973; Aylor, Citation1986). Rainfall washout is particularly important in the spread of fungal pathogens vast distances from their sources, and has been implicated as the primary process involved in the deposition of P. pachyrhizi urediniospores into the central US soybean producing regions (Krupa et al., Citation2006; Isard et al., Citation2007; Barnes et al., Citation2009). This is because rainfall can remove spores from a column of air extending thousands of metres upward and deposit them onto hosts in wet and cloudy environments suitable for the germination and subsequent infection of many pathogens (Aylor, Citation1986). Spore wet deposition is dependent upon: (i) the altitude from which precipitation falls; (ii) aerial concentration of spores in air layers below this altitude; (iii) rain droplet capture efficiency of spores; (iv) rainfall rate; and (v) duration of rainfall (Chamberlain, Citation1953; Hirst, Citation1953; Aylor, Citation1986). The small size (18–38 μm dia.) and highly echinulated surface of P. pachyrhizi urediniospores are characteristics that make them suitable for efficient collection by raindrops (Gregory, Citation1973; Bromfield, Citation1984; Aylor, Citation1986).
The importance of rainfall in the dispersal of microbes has been the focus of much research since the 1880s (Chamberlain, Citation1967; Gregory, Citation1973; Fitt et al., Citation1989; Madden, Citation1992). However, there is limited information about the vertical distribution of fungal spores deposited by rainfall within plant canopies. Knowledge about fungal spore deposition depth is important for determining the proportion of spores that land in environments favourable to germination. In the present study, a rainfall simulator (Dufault & Isard, Citation2010) and detached soybean leaf bioassay were used to examine the wet deposition of P. pachyrhizi urediniospores into a soybean canopy. The objective of this study was to determine the effects of rainfall intensity and row spacing on the vertical distribution of urediniospores deposited by rainfall within a soybean canopy. It was hypothesized that greater proportions of urediniospores would be deposited in the middle and lower sections of soybean canopies by higher intensity rainfall events and in wider soybean plant row spacing than in lower intensity rainfall events and narrow plant row spacing.
Materials and methods
Field sites
Two experimental repetitions were conducted during June and July 2007 in soybean fields of the Delta and Pine Land determinate cultivar DP7220RR (Monsanto Co.) at the University of Florida, North Florida Research and Education Center (NFREC) in Quincy, FL. These experiments were established in separate field sites (100 × 30 m) 300 m apart that were drill planted (370,000 seeds ha−1) separately on Julian dates 121 and 152. Within each field site, three 30 × 15 m subdivisions were demarcated and split equally into two randomly assigned field sections (15 × 15 m) of 19 and 76 cm row spacing, producing a total of six field sections at each field site. Both experiments were completed over a 20 day period, the first occurring when the average soybean plant height was 75 cm and the second when the height of the plants in the field averaged 35 cm. Simulations of high and low rainfall intensities were replicated four times, at different locations within the field sections planted at 19 and 76 cm row spacing for each of the two experiment repetitions, generating a total of 32 rainfall simulations (). For this study, the rainfall simulator was designated as the experimental unit and used to establish simulation areas of 2 × 2 m containing between 150 and 200 plants at the different locations in each field section.
Table 1. Environmental variables and the Phakopsora pachyrhizi urediniospore suspensions information associated with the different row spacing, plant height and rainfall intensity combinations
Inoculum and detached leaf bioassay
Soybean plants (DP7220RR) were grown in a greenhouse in round plastic pots (19 cm diameter × 18 cm deep) filled with Miracle-Gro Enriched Potting Mix (Micracle-Gro Lawn Products, Inc; N2H3-P2O5-K2O: 0.21-0.07-0.14). The seeds were sown 2 cm below the soil surface and the plants were fertilized once each month with 5 cm3 of Osmocote® Outdoor & Indoor Smart Release Plant Food (The Scott's Company, N-P-K: 19-6-12).
Soybean plants used for urediniospore inoculum production were mist-inoculated (Isard et al., Citation2006a) at growth stages V2 to V4 with P. pachyrhizi urediniospores that had been collected from kudzu. These urediniospores were collected at multiple times and at different locations from infected kudzu patches within the Florida counties of Gadsden and Duval. After inoculation, the plants were maintained in a greenhouse with a relative humidity > 90%. Urediniospores were vacuum-collected from infected soybean leaves into 20 mL glass vials with a large single cyclone spore collector (G-R Manufacturing Co., Manhattan, KS). The spores were weighed into allotments of 0.15 g and stored in 1.5 mL Eppendorf tubes at 20 ºC for < 24 h.
The detached leaf bioassay used in the experiments was adapted from previously described bioassay techniques (Burdon & Marshall, Citation1981; Twizeyimana et al., Citation2007; Park et al., Citation2008). In the current study, a single detached soybean leaflet was placed adaxial side up onto the surface of 10 g L−1 water agar (Difco Bacto) in 100 × 15 mm plastic Petri dishes. After exposure to simulated rainfall treatments, these dishes were covered and incubated for 14 days on an indoor bench exposed to 12–14 h of natural sunlight (max solar radiation intensity: 45 W m−2) with a mean temperature of 24 ºC (± 5 °C). It was observed that tissue on an undisturbed soybean leaflet for these exposure conditions remained green on the agar surface for periods of 28–30 days. After the 14 day incubation period, disease severity (uredinia/unit area) was calculated by counting the number of uredinia/leaflet using a dissecting microscope (100 ×) and measuring leaflet area with a LI-3000 Portable Area Meter (LI-COR Environmental).
Soybean plants used in the detached leaf bioassay were grown in a greenhouse at the NFREC. Although soybean rust was present in low quantities on the research station and the greenhouse was not a secure facility, careful visual inspection of soybean plants on a daily basis from May through August 2007 indicated that the greenhouse remained rust-free. This was further supported by multiple bioassay leaf samples producing zero uredinia after each experimental simulation. Preliminary standardization experiments revealed that leaflets between the ages of 18 and 36 days (growth stages R2–R5) were uniformly infected by low (4000 urediniospores/leaflet) and high (130,000 urediniospores/leaflet) amounts of inoculum. Consequently, leaflets between the ages of 18–36 days were used in the rainfall simulation experiments.
Rainfall simulation and assessment
Before each rainfall simulation, average plant height was calculated using five height measurements from random locations in each field section (). A portable, single nozzle, plot rainfall simulator that produces rainfall comparable to the short duration (< 5 min) precipitation events with intensity rates of 45 and 85 mm h−1 was used in this study (Dufault & Isard, Citation2010). These intensities are commonly observed for short periods (e.g. 1–5 min intervals) during warm weather convective storms in Illinois (Huff, Citation1970, Citation1974) and throughout the rest of the USA (Bodtmann & Ruthroff, Citation1976). The simulator was equipped to inject pressurized spore suspensions into the water flow that is uniformly dispersed over an area of 2 × 2 m. The simulator was positioned within a field section so that a simulation area covered 150 to 200 soybean plants in either 10 or 3 rows of the 19 and 76 cm row spacing treatments, respectively.
Inside the simulation areas, urediniospore deposition in the canopy was sampled at two randomly chosen locations within the rows and away from the edge of the simulation area using a detached leaf bioassay. A bioassay was used to sample urediniospore wet deposition because it provided an accurate estimate of viable urediniospore retention on soybean leaf tissue. Petri dishes (100 × 15 mm) containing 10 mL of 10 g L−1 water agar and one detached soybean leaflet placed adaxial side up were affixed to aluminium plates mounted parallel to the ground on plastic poles. These leaflets were placed on water agar to avoid dehydration and distortion of the leaf tissue during simulations. The plates were positioned to prevent overlapping and were adjusted relative to plant canopy height (ht) for the low (0.3 ht), mid (0.6 ht) and high (1 ht) collection level treatments. Once the leaflets were attached to the aluminium plates, the simulator's curtains were closed and urediniospore suspensions were mixed. A urediniospore suspension consisted of 0.15 g of urediniospores in 2 L of double distilled water that had been agitated for approximately 1 min. The urediniospore suspensions were mixed immediately before each rainfall simulation to prevent spore germination within the suspension. Approximately 20 mL of the urediniospore suspension was collected before each simulation and stored on ice (< 1 h) for determining the germination proportion and concentration of urediniospores in suspension. Each rainfall simulation consisted of a 30 s period of rainfall without spores and then a 4.5 min (range: 4.4–5.2 min) period of rainfall with spores. After each simulation, any excess water was drained from the Petri dishes and the sample dishes were collected, covered and transported to the lab for incubation and disease assessment.
The urediniospore viability of each simulation suspension was determined by pipetting 1 mL of collected suspension onto three separate 100 x 15 mm plastic Petri dishes containing 10 g L−1 water agar and calculating the proportion of spores that germinated. These plates were incubated in complete darkness at 24 ºC (± 5 ºC) for 16 h. The number of germinating urediniospores observed in the first 100 spores examined on each plate was recorded using a microscope (200 ×) and the germination proportion for each suspension was averaged across three Petri dishes (). The estimated urediniospore concentration (spores mL−1) calculated from four hemacytometer counts of the nine 1 mm2 grids was recorded for each rainfall simulation suspension ().
Data analysis
The uredinia density (uredinia cm−2) proportion was calculated by dividing the uredinia density for each collection level sample by the sum of the uredinia density for all three collection level samples at a given measurement location. For example, if the uredinia density at one measurement location was 20, 16 and 4 uredinia cm−2 for the high, mid and low collection levels, respectively, then the individual uredinia density proportions would be 20/40 = 0.50, 16/40 = 0.4 and 4/40 = 0.10. These proportions were then averaged between the two simulation area measurement locations for each rainfall simulation run. A split–split plot mixed linear model with a covariate analysis of variance (ANOVA) (Aldworth & Hoffman, Citation2002) was used to examine the effects of rainfall intensity (RI), row spacing (RS), collection level (CL) and the covariate plant height (PH) on the logit [0.5*log(y/1-y)] transformed proportion of uredinia cm−2. The logit transformation was used to limit errors associated with proportion data having non-normal distributions and non-constant variances in linear ANOVA procedures (Kuehl, Citation2000; Johnson & Wichern, Citation2002). All analysis was completed by using PROC MIXED in SAS version 9.1 (SAS Institute, Cary, NC). The denominator degrees of freedom (DDF) were calculated using the SAT option for the Satterthwaite approximation (Aldworth & Hoffman, Citation2002). Pairwise comparisons of the means and their interactions were performed using a Tukey test (Honest Significant Difference).
Results
Analysis of covariance indicated that after adjusting for plant height, the collection level (CL) treatment significantly (P < 0.01) affected soybean rust uredinia density proportion on leaflets that had been exposed to simulated rainfall injected with P. pachyrhizi urediniospores (). The proportion of uredinia cm−2 recorded at different collection levels decreased with increasing depth in the soybean canopy (). Significantly greater proportions of uredinia cm−2 were recorded at the high CL (0.53) than at either the mid (0.32) and low (0.15) CLs. However, no significant difference (P > 0.10) in the proportion of uredinia cm−2 was observed between the mid and low CL treatments. Between 40 to 55% of the soybean rust uredinia leaflet density was observed at the mid and low collection levels for all treatments examined in this study ().
Table 2. Main and interaction effects of rainfall intensity, row spacing, collection level and the covariate plant height on the logit [0.5*log(y/1-y)] transformed proportion of uredinia cm−2 based on a linear mixed model analysis of variance. Grey shading indicates significant (P < 0.01) results
Fig. 1. The mean proportion of uredinia cm−2 (± standard error) observed within the soybean canopies at three collection level treatments adjusted relative to the average plant canopy height (ht) for the levels of low (0.3 ht), mid (0.6 ht) and high (1. 0ht). Circles represent the covariate of average soybean canopy plant height.
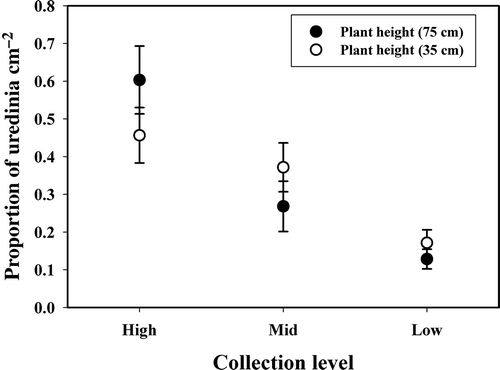
The interaction results of CL*row spacing (RS) and CL*rainfall intensity (RI) are shown in . The 19 and 76 cm RS treatments had approximately half of the uredinia cm−2 observed in the high CL (0.50 and 0.55, respectively), with only 0.14 and 0.16 being noted in the low CL, respectively. The proportion of uredinia cm−2 recorded at the high sampling position for the 85 mm/h RI treatment (0.45) was notably lower than that of the 45 mm/h treatment (0.61). However, for the 45 and 85 mm/h RI treatments similar proportions of 0.14 and 0.16 were observed at the low CL treatments, respectively.
Fig. 2. The mean proportion of uredinia cm−2 (± standard error) observed within the soybean canopies at three collection levels for the treatments of rainfall intensity and row spacing. The soybean canopy collection levels were adjusted relative to the average plant canopy height (ht) for the levels of low (0.3 ht), mid (0.6 ht) and high (1.0 ht).
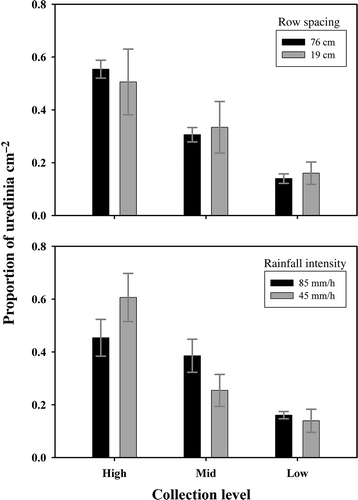
Discussion
Environmental variables critical to the germination and infection processes of soybean rust urediniospores (e.g. leaf wetness, ultraviolet radiation and temperature) vary with height and row spacing in a soybean canopy (Luxmoore et al., Citation1971; Ferrandino, Citation2008; Schmitz & Grant, Citation2009; Narvaez et al., Citation2010). In general, urediniospores that are deposited into the mid and low levels of soybean canopies will be exposed to more favourable environmental conditions for germination and infection than those deposited at higher levels. Consequently, the probability of a soybean rust infection occurring at a deposition site is highly dependent upon the depth at which P. pachyrhizi urediniospores are deposited within a canopy. This study demonstrates the importance of rainfall intensity, plant height and row spacing in determining the vertical distribution of wet deposited P. pachyrhizi urediniospores within a soybean canopy.
Our results indicate that the proportion of P. pachyrhizi urediniospores wet deposited and retained within a soybean canopy (estimated from uredinia density) decreases with canopy depth (). Approximately half of the wet deposited urediniospores were retained in the upper section of the soybean canopy, with the other half being distributed throughout the mid and low canopy sections. In general, more urediniospores were retained within the mid canopy section compared to the low canopy section, but differences between these levels were not significant. The row spacing and rainfall intensity treatments used in this study had no significant effect on the vertical distribution of wet deposited urediniospores retained within the soybean canopy, contrary to our initial hypothesis. However, noteworthy interaction trends were apparent between these variables and the collection level treatments (). The proportion of urediniospores retained in the low canopy section was small, ranging from 0.14 to 0.16, regardless of the row spacing or rainfall intensity treatment. For the short duration rainfalls that were simulated in this study, a greater proportion of wet deposited urediniospores were retained in the upper canopy section of the low intensity rainfall events compared with high intensity events (). These results suggest that the intensity of a rainfall event may impact the proportion of urediniospores retained deep within the soybean canopy. It is possible that rainfall events of different durations and/or intensities than those examined in this study might significantly affect the vertical distribution of wet deposited urediniospores; however, further research is needed to elucidate these relationships.
This study provides an estimate of the proportion of P. pachyrhizi urediniospores in rainwater that produce primary infections on soybean leaves. As far as the authors are aware, this is the first such estimate, and the information has been incorporated in IAMS forecasts for risk of soybean rust establishment. Measurements of leaf area obtained by electronically scanning soybean leaves collected from all plants in a 0.5 × 0.5 m sampling area at NFREC (19 cm row spacing with plants 75–100 cm in height) indicates that total leaf area ranges between four and seven times the sampling area (Andrade et al., Citation2009). Using these estimates of leaf area and the (1) mean number of viable urediniospores deposited per unit area within the simulation area (203 spores cm−2) (); and () overall experimental means of the uredinia density from bioassay leaflets exposed to simulated rainfall at low (mean = 0.41 spores cm−2), mid (mean = 0.73 spores cm−2), and high (mean = 1.30 spores cm−2) levels within the canopy, we estimated that between 5 and 8% of the viable P. pachyrhizi urediniospores that fall in a rainstorm on a mature soybean canopy produce uredinia. Roughly half of these infections occurred on leaves positioned at mid and low measurement levels (), where environmental conditions in a soybean field are considered most favourable for germination, and primary infections are typically first identified (Dorrance et al., Citation2008; Schmitz & Grant, Citation2009; Narvaez et al., Citation2010). Thus, it was calculated that roughly 2.5–4% of all the applied viable urediniospores produced uredinia in the mid and low canopy sections for all treatments. These percentages would likely be lower in field situations where conditions for germination and infection of urediniospores are less ideal than the incubation conditions used in this study. Also, since there is evidence that a majority of the spores washed from the atmosphere during a precipitation event at large distances from their source are deposited during the first 20 min of a rainstorm (Gregory, Citation1973; Li et al. Citation2008b), subsequent rainfall might remove P. pachyrhizi urediniospores from soybean leaves, reducing the proportion that result in primary infections even further.
Acknowledgements
This research was funded by grants from the USDA Cooperative States Research, Education and Extension Service, National Research Initiative Animal and Plant Biosecurity grant program and the Pennsylvania State University. We thank Dr Dario Narvaez and all the staff at the NFREC for their help with these experiments. We would also like to express our gratitude to Dr Glen Hartman and his staff at the University of Illinois for their support and help.
References
- Aldworth , J. and Hoffman , W.P. 2002 . Split-plot model with covariate: a cautionary tale . Amer. Statistic , 56 : 284 – 289 .
- Andrade , D. , Pan , Z. , Dannevik , W. and Zidek , J. 2009 . Modeling soybean rust spore escape from infected canopies: model description and preliminary results . J. Appl. Meteor. Climatol , 48 : 789 – 803 .
- Aylor , D.E. 1986 . A framework for examining inter-regional aerial transport of fungal spores . Agric. For. Meteorol. , 38 : 263 – 288 .
- Aylor , D.E. 1999 . Biophysical scaling and the passive dispersal of fungus spores: relationship to integrated pest management strategies . Agric. For. Meteorol , 97 : 275 – 292 .
- Barnes , C.W. , Szabo , L.J. and Bowersox , V.C. 2009 . Identifying and quantifying Phakopsora pachyrhizi spores in rain . Phytopathology , 99 : 328 – 338 .
- Bodtmann , W.F. and Ruthroff , C.L. 1976 . The measurement of 1 min rain rates from weighing raingage recordings . J. Appl. Meteorol. , 15 : 1160 – 1166 .
- Bromfield , K.R. 1984 . Soybean rust. Monograph , 1 – 58 . St. Paul , MN : The American Phytopathological Society .
- Burdon , J.J. and Marshall , D.R. 1981 . Evaluation of Australian native species of Glycines for resistance to soybean rust . Plant Dis , 65 : 44 – 45 .
- Chamberlain , A.C. 1953 . Aspects of travel and deposition of aerosol and vapor clouds , London : Atomic Energy Research Establishment. 1261, HMSO . Report 1261
- Chamberlain , A.C. 1967 . “ Deposition of particles to natural surfaces ” . In Airborne microbes , Edited by: Gregory , P.H. and Monteith , J.L. 138 – 164 . Cambridge : Cambridge University Press . Symposium of the Society for General Microbiology
- Del Ponte , E.M. and Esker , P.D. 2008 . Meteorological factors and Asian soybean rust epidemics – a systems approach and implications for risk assessment . Sci. Agric. (Piracicaba, Braz.) , 65 : 88 – 97 .
- Dorrance , A.E. , Draper , M.A. and Hershman , D.E. 2008 . Using foliar fungicides to manage soybean rust , Land-Grand Universities Cooperation NCERA-208 and OMAF, Bulletin SR-2008 .
- Dufault , N.S. and Isard , S.A. 2010 . “ A portable rainfall simulator for evaluating the wet deposition of plant pathogens ” . In App. Eng. Agric Vol. 26 , in press
- Ferrandino , F.J. 2008 . Effect of crop growth and canopy filtration on the dynamics of plant disease epidemics spread by aerially dispersed spores . Phytopathology , 98 : 492 – 503 .
- Fitt , B.D.L. , McCartney , H.A. and Walklate , P.J. 1989 . The role of rain in the dispersal of pathogen inoculum . Annu. Rev. Phytopathol , 27 : 241 – 270 .
- Gregory , P.H. 1973 . Microbiology of the atmosphere , 377 Aylesbury : Leonard Hill Books .
- Hartman , G.L. , Hines , R.A. , Faulkner , C.D. , Lynch , T.N. and Pataky , N. 2007 . Late season occurrence of soybean rust caused by Phakopsora pachyrhizi on soybean in Illinois . Plant Dis , 91 : 466
- Hirst , J.M. 1953 . Changes in atmospheric spore content: diurnal periodicity and the effects of weather . Trans. Br. Mycol. Soc , 36 : 375 – 393 .
- Huff , F.A. 1970 . Spatial distribution of rainfall rates . Water Resources Res. , 6 : 254 – 260 .
- Huff , F.A. 1974 . Statistics of precipitation . Journal Recherches Atmospheriques , 8 : 74 – 88 .
- Isard , S.A. and Gage , S.H. 2001 . Flow of life in the atmosphere: an airscape approach to understanding invasive organisms , East Lansing , MI : Michigan State University Press .
- Isard , S.A. , Gage , S.H. , Comtois , P. and Russo , J.M. 2005 . Principles of the atmospheric pathway for invasive species applied to soybean rust . BioScience , 55 : 851 – 861 .
- Isard , S.A. , Dufault , N.S. , Miles , M.R. , Hartman , G.L. , Russo , J.M. , De Wolf , E.D. and Morel , W. 2006a . The effect of solar irradiance on the mortality of Phakopsora pachyrhizi urediniospores . Plant Dis , 90 : 941 – 945 .
- Isard , S.A. , Russo , J.M. and De Wolf , E.D. 2006b . The establishment of a national Pest Information Platform for Extension and Education. Online . Plant Heath Progress , DOI: 10.1094/PHP-2006-0915-01-RV
- Isard , S.A. , Russo , J.M. and Ariatti , A. 2007 . The Integrated Aerobiology Modeling System applied to the spread of soybean rust into the Ohio River valley during September 2006 . Aerobiologia , 23 : 271 – 282 .
- Johnson , R.A. and Wichern , D.W. 2002 . Applied Multivariate Statistical Analysis , Upper Saddle River , NJ : Prentice Hall .
- Jurick , W.M. II , Narvaez , D.F. , Brennan , M.M. , Harmon , C.L. , Marois , J.J. , Wright , D.L. and Harmon , P.F. 2008 . Winter survival of soybean rust pathogen, Phakopsora pachyrhizi, in Florida . Plant Dis , 92 : 1551 – 1558 .
- Kemerait , R.C. , Sconyers , L.E. , Jost , P.H. and Mills , W.A. 2006 . “ Evaluation of fungicides for control of Asian soybean rust in Attapulgus, Georgia, 2005 ” . In Fungic. Nematic Tests 61, FC072
- Krupa , S.V. , Bowersox , V. , Claybrooke , R. , Barnes , C.W. , Szabo , L.J. , Harlin , K.S. and Kurle , J. 2006 . Introduction of Asian soybean rust urediniospores into the midwestern United States – a case study . Plant Dis , 90 : 1254 – 1259 .
- Kuehl , R.O. 2000 . Design of Experiments: Statistical Principles of Research Design and Analysis , Boston , MA : Duxbury .
- Levy , C. 2005 . Epidemiology and chemical control of soybean rust in Southern Africa . Plant Dis , 89 : 669 – 674 .
- Li , X. , Engelbrecht , C.J. , Mueller , D.S. and Yang , X.B. 2008a . First report of soybean rust caused by Phakopsora pachyrhizi in Iowa and its statewide occurrence . Plant Dis , 92 : 975
- Li , X. , Yang , X.B. , Mo , J. and Guo , T. 2008b . Estimation of soybean rust uredospore terminal velocity, dry deposition, and the wet deposition associated with rainfall . Eur. J. Plant Path , 123 : 377 – 386 .
- Luxmoore , R.J. , Millington , R.J. and Marcellos , H. 1971 . Soybean canopy structure and some radiant energy relations . Agron. J. , 63 : 111 – 114 .
- Madden , L.V. 1992 . Rainfall and the dispersal of fungal spores . Advan. Plant Path , 8 : 39 – 79 .
- Miles , M.R. , Levy , C. , Morel , W. , Meuller , T. , Steinlage , T. , van Rij , N. , Frederick , R.D. and Hartman , G.L. 2007 . International fungicide efficacy trials for the management of soybean rust . Plant Dis , 91 : 1450 – 1458 .
- Mueller , T.A. , Miles , M.R. , Hartman , G.L. , O'Brien , G.K. , Marois , J.J. and Wright , D.L. 2008 . Evaluation of fungicide timing for the control of soybean rust in northwest Florida, 2006 , Plant Disease Management Reports (online). Report 2: FC086. DOI:10.1094/PDMR02 .
- Narvaez , D.F. , Jurick , W.M. II , Marois , J.J. and Wright , D.L. 2010 . Effects of surface wetness periods on development of soybean rust under field conditions . Plant Dis , 94 : 258 – 264 .
- Pan , Z. , Yang , X.B. , Pivonia , S. , Xue , L. , Pasken , R. and Roads , J. 2006 . Long-term prediction of soybean rust entry into the continental United States . Plant Dis , 90 : 840 – 846 .
- Park , S. , Chen , Z.-Y. , Chanda , A.K. , Schneider , R.W. and Hollier , C.A. 2008 . Viability of Phakopsora pachyrhizi urediniospores under simulated southern Louisiana winter temperature conditions . Plant Dis , 92 : 1456 – 1462 .
- Pivonia , S. and Yang , X.B. 2004 . Assessment of the potential year-round establishment of soybean rust throughout the world . Plant Dis. , 88 : 523 – 529 .
- Pivonia , S. and Yang , X.B. 2006 . Relating epidemic progress form a general disease model to seasonal appearance time of rusts in the United States: implications for soybean rust . Phytopathology , 96 : 400 – 407 .
- Schmitz , H.F. and Grant , R.H. 2009 . Precipitation and dew in a soybean canopy: spatial variations in leaf wetness and implications for Phakopsora pachyrhizi infection . Agric. For. Meteorol , 149 : 1621 – 1627 .
- Sikora , E.J. , Delaney , D.P. , Delaney , M.A. , Lawrence , K.S. and Pegues , M. 2009 . “ Evaluation of sequential fungicide spray programs for control of soybean rust. Online ” . In Plant Health Progress DOI: 10.1094/PH-2009-0402-01-RS
- Thorson, T. (2008). Crop production – National Agricultural Statistics Service (NASS). Agricultural Statistics Board USDA http://www.nass.usda.gov (Accessed: 1 July 2009 ).
- Twizeyimana , M. , Ojiambo , P.S. , Ikotun , T. , Paul , C. , Hartman , G.L. and Bandyopadhyay , R. 2007 . Comparison of field, greenhouse, and detached-leaf evaluations of soybean germplasm for resistance to Phakopsora pachyrhizi . Plant Dis , 91 : 1161 – 1169 .
- Yorinori , J.T. , Paiva , W.M. , Frederick , R.D. , Costamilan , L.M. , Bertagnolli , P.F. , Hartman , G.L. , Godoy , C.V. and Nunes , J. Jr. 2005 . Epidemics of soybean rust (Phakopsora pachyrhizi) in Brazil and Paraguay from 2001 to 2003 . Plant Dis , 89 : 675 – 677 .